POLARIZED LIGHT MICROSCOPY IN CONSERVATION: A PERSONAL PERSPECTIVE
WALTER C. McCRONE
4 TECHNIQUES
Sampling of art and archaeological subjects and paintings (McCrone 1982) is usually done with very fine needles (fig. 8) often made as needed by etching the ends of 0.5 mm tungsten wire (24 gauge) with sodium nitrite as described in the Particle Atlas(McCrone et al. 1992). A reasonable substitute may be made in a few minutes from a steel dissecting needle using very fine emery paper. The tip diameter viewed from the side should be 5–10 μm in order to handle tiny samples in that size range. Samples smaller than the resolution limit of the unaided human eye are less than 0.1 mm in size, but they often consist of hundreds of individual pigment particles. This sample size is more than adequate for characterization or identification of the pigment components by PLM.
Fig. 8.
A dissecting needle and a fine tungsten needle used for sampling paintings and manipulating tiny particles, 70x
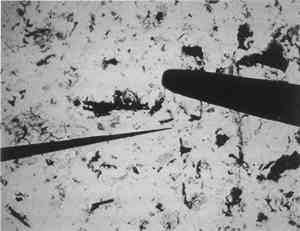 |
Low magnification, say 10–50x with the stereomicroscope, aids in sampling paint surfaces, either ultramicro samples with the fine tungsten needle or small cross sections with a slightly coarser needle tip diameter or a fine scalpel. We make our own needles and scalpels. We prepare tiny scalpels by breaking 1–2 mm lengths of a fresh, two-edged safety razor using fine-tipped pliers. When necessary, we grind the pliers tips to about 1 mm2 gripping area. The small bits of razor blade, with only 2–5 mm of cutting edge, are placed in a vial for future use. When needed, a likely candidate is removed with fine-tipped forceps and oriented in a needle-holding chuck with the razor edge exposed and oriented at an appropriate angle (usually 30–60� from the length of the chuck). Tightened firmly, it furnishes a very sharp edge nicely oriented to scrape a surface or cut a thin section to mount for study and testing by stereomicroscope and PLM. The thin section is held in place on edge in a drop of thermoplastic Aroclor 5442 or embedded in polyester or epoxy in order to grind and polish a more formal surface through the various paint layers. Staining and other tests are performed directly on either type of section, and individual paint layers are sampled with a fine tungsten needle for study by PLM.
Samples for identification are usually mounted dry under a coverslip taped onto one end of a microscope slide (McCrone 1982). Tinier portions of that sample are taken with the fine tungsten or steel needle tip and mounted in the center of the same slide using Aroclor, an ideal permanent mounting medium (McCrone 1984b). Any individual particle is removed later if necessary (McCrone 1984a) for characterization by other microanalytical methods (SEM/EDS, FTIR/microscopy, etc.).
The PLM is used by mineralogists to identify rocks and minerals and by chemical microscopists to identify a wide variety of microscopic particles, for example, pollens and spores, manmade and natural fibers, minerals, crime lab trace evidence, industrial powders, metals, contaminants, and settled dust (McCrone et al. 1992). Thousands of tiny particles are identified by noting size, shape, color, transparency, surface topography, edge contrast, etc., almost at sight by a trained polarized light microscopist. Cotton (fig. 9) and hemp fibers (fig. 10) are each identified as such, at sight, as are softwood (fig. 11), and wool (fig. 12), as well as pigments such as gamboge (fig. 13), alizarin (fig. 14), vermilion (fig. 15), and charcoal (fig. 16). Fortunately, a polarized light microscopist becomes so familiar with the appearance of many substances that even with quick confirmatory tests and observations, recognition is nearly as rapid as identifying microscopes, telephones, cameras, or an automobile macroscopically. The quick confirmatory tests are as simple as crossing the polars, focusing up and down, or rotating the microscope stage. With very little more time, the species of pollens, animal hairs, or vegetable fibers, composition of gilt, or the identification of paint media is determined by the PLM microscopist experienced in those areas.
Fig. 9.
Cotton fibers showing characteristic twists, crossed polars, 70x
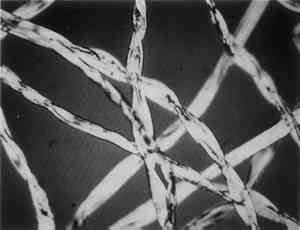 |
Fig. 10.
Hemp fibers showing typical nodes (cross markings), 70x
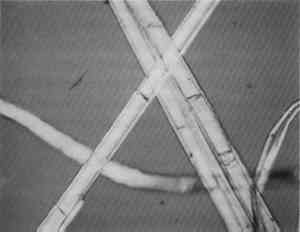 |
Fig. 11.
Softwood fibers showing pits on flat ribbonlike fibers, crossed polars, 70x
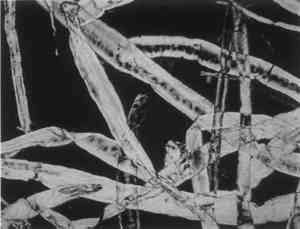 |
Fig. 12.
Wool, 70x
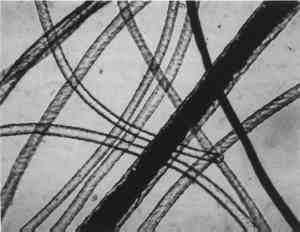 |
Fig. 13.
Noncrystalline gamboge is unique in shape and color. 70x
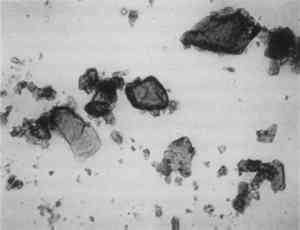 |
Fig. 14.
Alizarin carries a post-1826 date. 70x
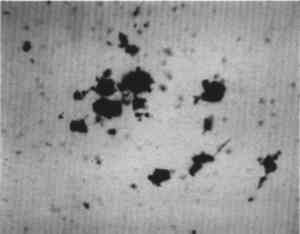 |
Fig. 15.
Vermilion is used in three different forms with three different dates of first use. 70x
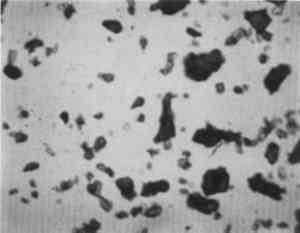 |
Fig. 16.
Charcoal often shows shape evidence for its wood fiber origin. 70x
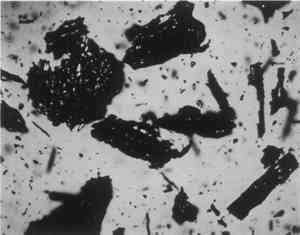 |
For those particles not identified at sight, PLM has an arsenal of tests designed to produce sufficient additional data to help in identification (McCrone et al. 1984). First, the refractive index relative to the mounting media is quickly estimated qualitatively. The readings, based on darkness of the particle boundaries (contrast) and the Becke line behavior on focusing up and down through best focus might be nearly equal, higher, lower, much higher, or much lower. Rotating the stage may yield a similar observation for a different orientation of the particle relative to the vibration direction of the polarized light.
Next, crossing the polars causes some substances to disappear against the resulting black background (e.g., cubic crystals and glasses). Other particles appear bright, and the colors (and their intensity) correspond to the difference between the two refractive indices shown by those particles; this is estimated (e.g., small, medium, or large) or measured quantitatively. On rotation of the stage, all such colored particles disappear (show extinction) every 90�. The particle orientation at extinction is characteristic and helps as an additional identifying characteristic.
All these observations are very rapidly carried out. There are others, less rapidly performed but increasingly definitive. These include actual measurement of one or more refractive indices by immersion in standard Cargille refractive index liquids to find a match; addition of a compensator to determine the orientation of the high refractive index; measurement of the extinction angle (the angle between extinction and a prominent particle shape direction); and measurements on the interference figure. The latter might well be described as a “fingerprint” for a crystalline substance. A valuable group of quantitative and qualitative measurements are made on such figures. All these observations and measurements usually consume 15–20 minutes.
In addition, one may elect to measure thermal properties using a hot stage or to perform microchemical tests in an aqueous drop using standard reagents for inorganic ions or for organic compounds. A series of staining tests on single particles of a starch grain or a crystal of Prussian blue are examples of this quick test procedure. Seldom is it necessary to perform more than a few of these tests given the knowledge that the particles are one of about 10 pigments of a given color, one of the small number of paint media, or one of the common starch grains or bast fibers.
Still, a microscopist should ideally use the best and surest analytical tool to solve the tougher problems. One such problem is the differentiation of cadmium red, red lead, vermilion, hematite, and red ochre. All are finely divided red pigments, and all have refractive indices higher than any Cargille refractive index liquid. Most pigments are identifiable at sight, but an elemental analysis by SEM/EDS helps solve problems like the identification of these red pigments or other look-alikes. Vermilion shows mercury and sulfur, red lead shows lead, iron earth pigments show iron and (with the umbers) manganese, cadmium red shows cadmium, sulfur, and/or selenium. These microchemical tests can be made in single-drop tests on a microscope slide, but, when available, most microscopists prefer to use SEM/EDS. This technique also helps to differentiate among ultramarine, smalt, and Prussian blue or among lead-tin yellow, zinc yellow, and Naples yellow, and other such look-alike problems. Some microscopists even add the whites (lead, zinc, and titanium) to this list. With care and a good microscopical background, however, all can be differentiated by PLM.
It is also possible to carry out chemical reactions as another means of identifying pigments, corrosion products, minerals, or metals. These tests generally involve single-drop volumes of test substance and reagent resulting in precipitation of characteristic crystal formations, performed on tiny subnanogram samples when necessary (McCrone 1986). Single particles of most pigments are identified by microchemical tests using PLM. Likewise, single fibers and very short lengths thereof are uniquely characterized and identified microscopically by their optical properties. Many staining reactions as well as ultraviolet fluorescence are also adaptable to observation with PLM.
In sum, the combination of PLM, FTIR/microscopy, and SEM/EDS effectively and efficiently solves 99% of the problems we face. I would have to add that a trained polarized light microscopist could likely solve more of the conservator's problems than both of the other two together, but having all three facilitates the solution of many problems.
|