CHARACTERIZATION BY FTIR OF THE EFFECT OF LEAD WHITE ON SOME PROPERTIES OF PROTEINACEOUS BINDING MEDIA
SILVIA A. CENTENO, MARCELO I. GUZMAN, AKIKO YAMAZAKIKLEPS, & CARLOS O. DELLA V�DOVA
4 DISCUSSION
The shift observed in the amide I maxima to higher wavenumbers for the mixtures with lead white, with respect to the values observed for the pure binders, when conditioned at 20�C and 55% RH, and the results obtained when the samples were exposed to a higher humidity (70% and 90% RH) indicate that lead white induces a modification in the state of hydration of the binders.
Modifications in the state of hydration of proteinaceous materials are expected to be reflected in changes in the FTIR spectra. These changes, which occur simultaneously, can be separated in two groups: (1) those that result from the influence of the hydrogen bonding of water on hydration sites such as C=O and N-H, resulting in changes in the intensities and positions of the corresponding IR bands (Pesvner and Diem 2001),
Fig. 4.
Detail of the FTIR spectra of gelatin mixed with lead white conditioned at 20�C and 90%, 70%, and 55% RH in the 1900–1200 cm-1 spectral range. Arbitrary absorbance units.
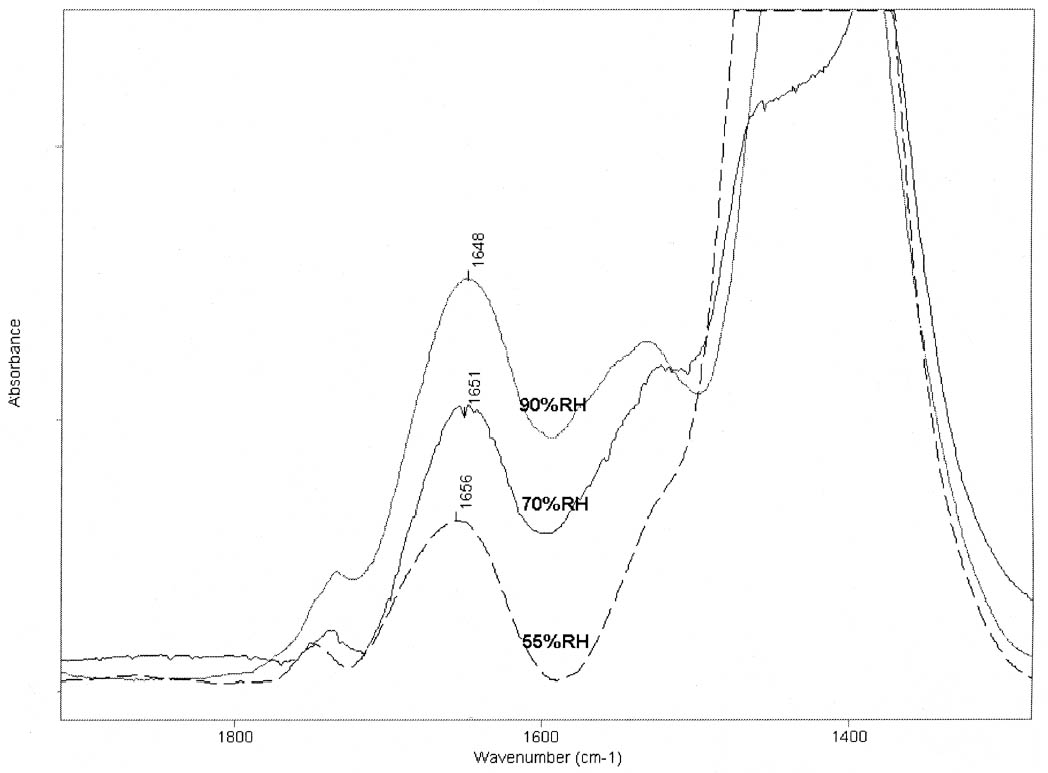 |
Fig. 5.
Detail of the FTIR spectra of gelatin (—) and gelatin mixed with massicot (——), conditioned at 20�C and 55% RH, in the 1900–1200 cm-1 spectral range. Arbitrary absorbance units
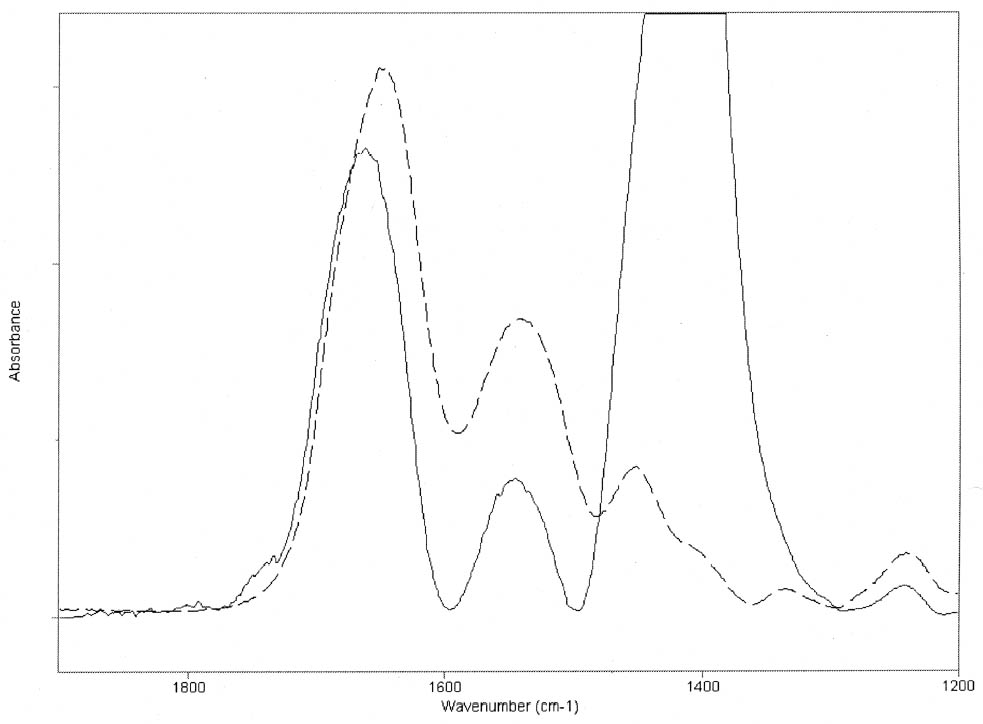 |
and (2) those due to changes in the secondary structure of the protein (Allison et al. 1998; Soulliac et al. 2002). Upon removal of water molecules from the vicinity of the peptide linkage, a shift of the amide I band to higher wavenumbers is expected as a result of the combined effect of an increase in the force constant of the C=O bond (bond strength) and a decrease in the reduced mass of the C-O vibrator in view of the decoupling with O-H. The amide II band is expected to shift to lower wavenumbers as the force constant of the C-N decreases by a decreased “stiffness” of the H-N-CO angle due to a higher “mobility” of H through a decrease in the hydrogen bonding and a decreased strength of the C-N bond. It can be concluded that lead white induces a modification in the environment around the peptide carbonyl C=O and the CNH groups, by reducing the number of surrounding water molecules and, therefore, reducing hydrogen bonding.
The results obtained for the massicot-containing mixtures indicate that this pigment also induces a change in the water structure surrounding the peptide C=O group but a much smaller change in the hydrogen bonding of the CNH group.
The dominant amide I maxima remain around the 1660–1650 cm-1 range for mixtures containing lead white or massicot, so no drastic changes in the proteins' secondary structures are taking place upon mixing with the pigments. However, the slight increase in the area of the 1683 (gelatin)/1688 (gelatin–lead white) cm-1component (see figs. 3a, 3b) relative to the amide I band area is revealing a partial conversion of α-helices to β-turns due to aggregation. No attempt was made to quantify the proportions of the different protein secondary structures due to their different molar absorptivities (De Jongh et al. 1996).
The mechanism by which lead white and massicot induce these changes in the proteins' water structure remains to be elucidated. Some salts have been demonstrated to affect the interactions within the protein through modification of the water structure (Damodaran and Kinsella 1982). Depending on the hydration energy and steric hindrance of the anions, salts may promote aggregation, unfolding, or dissociation of the protein (Meng and Ma 2001).
The results of the experiments carried out are expected to be independent of the substrate over which the paint layer is applied (canvas, parchment, paper, wood) and of the thickness of the paint layer, provided that the pigment-binder composition is uniform throughout it.
It should be taken into account that temperature and pH conditions during the manufacturing process of the binders will also affect the mechanical stability of the dried paint film. The influence of lead white on the partial hydrolysis that gelatin undergoes upon aging (Dupont 2002) and other degradation processes that may take place when the mixtures age naturally or are subject to accelerated aging under different conditions, such as the yellowing reported by Lawson and Yamazaki-Kleps (2002), are worth an in-depth separate study.
In addition, medieval treatises often recommended mixing the proteinaceous material with a glutinous or carbohydrate binder (see, for example, Merrifield 1999, 316, 156). It is well known that some carbohydrates, when added in the right proportions, form hydrogen bonds with the protein and protect it from dehydration-induced damage (Allison et al. 1998; Allison et al. 1999). The characterization of this aspect of the degradation process suggests that the addition of carbohydrates to proteinaceous materials used in consolidation treatments of lead white flaking areas may prove to be beneficial for the long-term stability of the damaged areas, though the mechanical stability and the chemical and color properties of the consolidant mixtures should be systematically evaluated first.
|