CHARACTERIZATION BY FTIR OF THE EFFECT OF LEAD WHITE ON SOME PROPERTIES OF PROTEINACEOUS BINDING MEDIA
SILVIA A. CENTENO, MARCELO I. GUZMAN, AKIKO YAMAZAKIKLEPS, & CARLOS O. DELLA V�DOVA
ABSTRACT—Cracking, flaking, and separation of paint films containing lead white from the substrate on medieval parchment manuscripts and other works of art have been frequently reported by conservators. In this study, Fourier transform infrared spectroscopy (FTIR) was used to follow up the changes induced by the pigment on some properties of proteinaceous binding media commonly mentioned in medieval treatises. Evidence of the removal of water molecules from the vicinity of the peptide linkage groups C=O and CNH, with a small change in the protein's secondary structure due to aggregation, was obtained. The properties of the lead white–binder samples were compared to those of massicot-containing mixtures. Massicot was also found to promote aggregation of the binders, though it induces a smaller change than does lead white in the hydrogen-bonding characteristics of the peptide CNH group.
TITRE—Caract�risation par FTIR de l'effet du blanc de plomb sur certaines propri�t�s des liants prot�iques. R�SUM�—Les restaurateurs ont fr�quemment not�, dans le cas des manuscrits m�di�vaux en parchemin ou d'autres oeuvres de cette p�riode, la pr�sence de craquelures, d'�caillage et de soul�vements dans les peintures � base de blanc de plomb. Dans cette �tude, on a utilis� la spectroscopie infrarouge � transform� de Fourier ( FTIR) pour suivre l'�volution des changements induits par le pigment sur certaines propri�t�s des liants prot�iques couramment mentionn�s dans les trait�s m�di�vaux. Les r�sultats obtenus indiquent qu'il y a retrait des mol�cules d'eau � proximit� des groupes C=O et CNH du lien peptidique, accompagn� d'un changement mineur par agr�gation dans la structure secondaire des prot�ines. On a compar� les propri�t�s des �chantillons de blanc de plomb-liant avec ceux � base de massicot. On a constat� que le massicot favorise �galement l'agr�gation des liants, mais qu'il induit un changement moins important que le blanc de plomb dans les caract�ristiques de la liaison hydrog�ne du groupe peptidique CNH.
TITULO—Caracterizaci�n por medio de FTIR del efecto del blanco de plomo sobre algunas propiedades de medios aglutinantes prot�icos. RES�MEN—El agrietamiento, la descamaci�n y la separaci�n del sustrato de pel�culas de pintura que contienen blanco de plomo en manuscritos medievales en pergamino y otras obras de arte han sido frecuentemente reportados por conservadores. En este estudio, se us� espectroscop�a infrarroja por transformada de Fourier ( FTIR) para caracterizar los cambios inducidos por el pigmento en algunas propiedades de medios aglutinantes prot�icos com�nmente mencionados en tratados medievales. Se obtuvo evidencia de la remoci�n de mol�culas de agua del entorno de los grupos C=O y CNH del enlace pept�dico y de un cambio peque�o en la estructura secundaria de la prote�na debido a la agregaci�n. Las propiedades de las muestras de blanco de plomo con los distintos medios aglutinantes se compararon con las de mezclas que conten�an el pigmento masicote. Se encontr� que el masicote tambi�n promueve la agregaci�n de los medios aglutinantes, no obstante induce un cambio m�s peque�o que el blanco de plomo en los enlaces de puente de hidr�geno caracter�sticos del grupo CNH del enlace pept�dico.
T�TULO—Caracteriza��o por FTIR do efeito do branco-de-chumbo em algumas propriedades das ligas proteicas. RESUMO—O craquelamento, a descama��o e a separa��o de pel�culas de tinta contendo branco-de-chumbo do substrato em pergaminhos manuscritos medievais e em outras obras de arte t�m sido freq�entemente relatados por conservadores. Neste estudo, a espectroscopia infravermelha por transformada de Fourier (FTIR) foi usada para acompanhar as mudan�as induzidas pelo pigmento em algumas propriedades das ligas proteicas comumente mencionadas nos tratados medievais. Obteve-se evid�ncia da remo��o de mol�culas de �gua das proximidades dos grupos de liga��o pept�dica C=O e CNH, com uma pequena mudan�a na estrutura secund�ria da prote�na devido � agrega��o. As propriedades das amostras de brancode-chumbo foram comparadas com as misturas que cont�m massicote. Foi descoberto que o massicote tamb�m promove a agrega��o das ligas, embora ele induza uma mudan�a menor do que o branco-dechumbo nas caracter�sticas das liga��es de hidrog�nio do grupo pept�dico CNH.
1 INTRODUCTION
Lead white, 2PbCO3•Pb(OH)2, can be considered the most important of all white pigments from a historical point of view. It is mentioned in almost all lists of pigments from ancient times to the present (Gettens et al. 1993). It has been used in a variety of media, conferring them with different properties. When used ground in oil media, it is known to accelerate the drying of the oil, giving a product that forms a hard film and that is more resistant than most other oil-pigment mixtures. Changes in the chemical properties of linseed oil mixed with lead white have been characterized by Fourier transform infrared spectroscopy (FTIR) and attributed to the saponification of esters and the deprotonation of carboxylic acids to form lead carboxylates (Meilunas et al. 1990). Using gas chromatography–mass spectroscopy (GC-MS), van den Berg et al. (1999) reported that, as lead white can enhance the photochemical degradation of oil binders, small breakdown products like formic acid are formed and these products interfere with the metal carboxylates already present, leading to an increased extractability of organic fractions and the consequent release of the pigment and loss of mechanical stability of the paint film. Boon et al. (2002) further characterized the dissolution, metal soap formation, and remineralization processes that occur in lead-pigmented ground/intermediate oil paint layers using FTIR, scanning electron microscopy–energy dispersive x-ray spectroscopy (SEM-EDX), and secondary ion mass spectroscopy (SIMS).
Cracking, flaking, and separation of the paint film from the substrate have been reported by several conservators on areas containing lead white on parchment and other works of art (Drayman 1968-69; Bykova et al. 1976; Quandt 1992). Lawson and Yamazaki-Kleps (2002) attributed the problems ranging from friability to cracking, tenting, and cleavage observed in the manuscript “The Belles Heures of Jean of France, Duke of Berry” to the interaction of the lead white with the binding media. However, no systematic study on the effect of the pigment on the properties of proteinaceous or polysaccharide binders commonly used in medieval illuminated manuscripts has been carried out.
Lawson and Yamazaki-Kleps (2002) carried out accelerated aging tests in a fadeometer on samples of proteinaceous, gum, and cellulose derivative binders and their mixtures with lead white. Aging in a fadeometer was found to promote yellowing in all the protein–lead white mixtures. The mixtures containing Kremer gelatin presented the smallest color change of all the binders tested. In the particular case of the medieval manuscript TheBelles Heures of Jean of France, Duke of Berry, yellowing is not apparent in the passages containing lead white, probably because the folios have been kept protected from exposure to light. However, as mentioned above, selective cracking of these areas occurs when compared with paint passages containing other pigments in the same folio.
In this study, mixtures of lead white with different proteinaceous binders were analyzed by FTIR to determine the effect of the pigment on the hydration properties of the fresh binders. Glair, i.e., egg white, and animal glue were commonly mentioned in medieval treatises as binders (see, for example,Thompson 1956, 51–61; Merrifield 1999, 232, 316), so glair prepared by the whipping method, rabbit skin glue, bone glue, and gelatin were chosen for analysis. In addition, whipped glair, rabbit skin glue, and gelatin mixed with the yellow lead–containing pigment massicot, PbO, were studied by FTIR to compare their properties to those of the samples containing lead white.
The composition of gelatin, glair, and animal glue has been published by several authors (Mills and White 1999 and references therein). Gelatin is produced by partial hydrolysis of collagen in either an alkaline or acid medium, both giving a partially denaturated protein as a product. These treatments result in the formation of a high proportion of α-chains. Linked α-chains, such as β-chains (α-chain dimers), nonhydrolyzed or partially renaturated triple helices, and polypeptides resulting from the degradation of α-chains are also present in gelatins (Dupont 2002). Rabbit skin glue and bone glue are generally prepared by the digestion of the animal tissue in hot water, and their main component is also gelatin, whereas the main component of glair is ovalbumin, a glycoprotein (Mills and White 1999 and references therein).
2 EXPERIMENTAL
Lead white (2PbCO3•Pb(OH)2), massicot (PbO), gelatin sheet, genuine rabbit skin glue, and bone glue were purchased from Kremer Pigments Inc. and were used without further purification.
Lead white (2PbCO3•Pb(OH)2) and PbO were characterized by Raman spectroscopy using a Renishaw Raman System 1000, configured with a Leica DM LM microscope. The laser light source used was a diode laser (785 nm) focused on different areas of the samples using 50x objective lens, allowing spatial resolution on the order of 2–3 μm. The power reaching the sample was set between 0.1 and 10 mW using neutral density filters. The spectra of both commercial pigments were found to match those of lead white and massicot in the N. Stolow Reference Pigment Sample Collection, Set B180 (Paper Conservation Department, The Metropolitan Museum of Art).
Glair was prepared by whipping fresh egg white with a pair of wooden sticks until foam was formed. A clear liquid phase separated when the foam was set aside for about an hour, and this liquid was used undiluted as a binding medium. The rest of the binders were prepared as 10% solutions. The concentration of these solutions is probably higher than the ones used in medieval manuscripts, but it was chosen to ease the detection of the IR bands in the mixtures with the pigments. Samples of the binders mixed with lead white and massicot were prepared using 4 ml of the solution of the binder, or pure glair, and 5 g of the pigment. Both the solutions of the binders and their mixtures with the pigments were painted (three brushstrokes in the same direction) on plain microscope slides.
Different sets of samples were conditioned by placing them in a humidity cabinet (Espec LHL-112, Tabai Corp.) at 20�C and 55%, 70%, or 90% RH, respectively, for four days. Once the samples were taken out from the humidity cabinet, they were examined within 5–10 minutes to avoid any changes.
FTIR was carried out on a Bio-Rad FTS 40 equipped with a UMA 500 microscope. The samples were placed between the windows of a diamond anvil cell, and the spectra were recorded with a 2 cm-1 resolution. Purging of the spectrometer with dry nitrogen was carried out to minimize the contribution of H2O vapor absorption to the shape of the amide I and amide II bands.
Fourier self-deconvolution, second-derivative calculations, and curve fitting were applied with the aim to identify the principal bands that make up a more complex one with overlapping features. Second-derivative and Fourier self-deconvoluted spectra using Grams/32 Derivatv. A and Grams/32 Deconvolv. AB softwares from Galactic Industries Corp. were used as peak position guides for the curve-fitting procedure. These initial peak positions were subsequently used in a nonlinear curve fitting of a mixed Gaussian/Lorentzian function to obtain more exact peak parameters. Criteria used to evaluate the resulting fit were the absence of a systematic deviation in the difference between the fitted and the measured spectra, and the minimization of the reduced χ2 value.
3 RESULTS
The FTIR spectra obtained for pure gelatin, glair, rabbit skin glue, and bone glue present the typical features of proteinaceous materials. The spectrum corresponding to a pure gelatin sample in shown in figure 1a. The amide I band region (~1655 cm-1) consists of amide carbonyl C=O stretching with some contribution of CN stretching and CCN deformation. The amide II (~1550 cm-1) band involves both C-N stretching and C-N-H in plane bending (Lin-Vien et al. 1991; Bandekar 1992). Of the different bands originating from the peptide linkage, only the amide I band has proved to contain significant information about the protein secondary structure. Generally, α-helices absorb in the range 1660–1650 cm-1, β-sheets in the range 1640–1620 cm-1 and near 1675 cm-1, β-turns in the range 1695–1660 cm-1, unordered structures and α-helices 1650–1640 cm-1 (Krimm and Bandekar 1986;Susi and Byler 1986). Therefore, for the analysis of possible changes in the protein secondary structure by 2PbCO3•Pb(OH)2 or PbO, we concentrated on the more informative amide I band region. The amide II band positions were analyzed to get information about the environment and hydrogen-bonding characteristics of the peptide CNH bonds.
The FTIR spectrum of the basic lead carbonate 2PbCO3•Pb(OH)2 (fig. 1c) has been fully assigned (White 1974). In the spectra of the lead white–binder mixtures, features corresponding to the lead white pigment overlap those of the pure binder, particularly the ν3 CO3-2 mode at ~1400cm-1 that partially overlaps the amide II band of the protein, so Fourier self-deconvolution, second-derivative calculations, and curve fitting were used to determine the exact peak positions. The spectrum corresponding to the gelatin–lead white mixture is shown in figure 1b.
Table 1 presents a comparison of the amide I and II groups maxima for the pure binders and their mixtures with lead white conditioned at 20�C and 55% RH. The amide I maxima for the mixtures shift toward higher wavenumbers, while the amide II maxima shift toward lower wavenumbers with respect to the values observed for the pure binders. Views of the spectra of pure gelatin and its mixture with lead white showing the 1900–1200 cm-1 range in detail are shown in figure 2. Peaks obtained from the curve fitting on the amide I–amide II region are shown for gelatin and the gelatin–lead white mixture in figures 3a and 3b. These results were found to be completely reproducible and independent of the length of exposure to the infrared (IR) radiation during the measurements.
Upon Gaussian–Lorentzian fitting, a minor peak component at 1683 cm-1 was obtained for pure gelatin (see fig. 3a). This maximum shifts to 1688 cm-1 in the mixture with lead white (see fig. 3b). The area of this peak relative to the area of the amide I band increases by a factor of 10 for the mixture with lead white with respect to the value obtained for the pure binder.
When two other sets of samples were conditioned at 70% RH and 90% RH, respectively, it was found that the amide I maxima progressively shift to lower
Fig. 1.
FTIR spectra of gelatin (a), gelatin mixed with lead white (b), and pure lead white (c), conditioned at 20�C and 55% RH. Arbitrary absorbance units
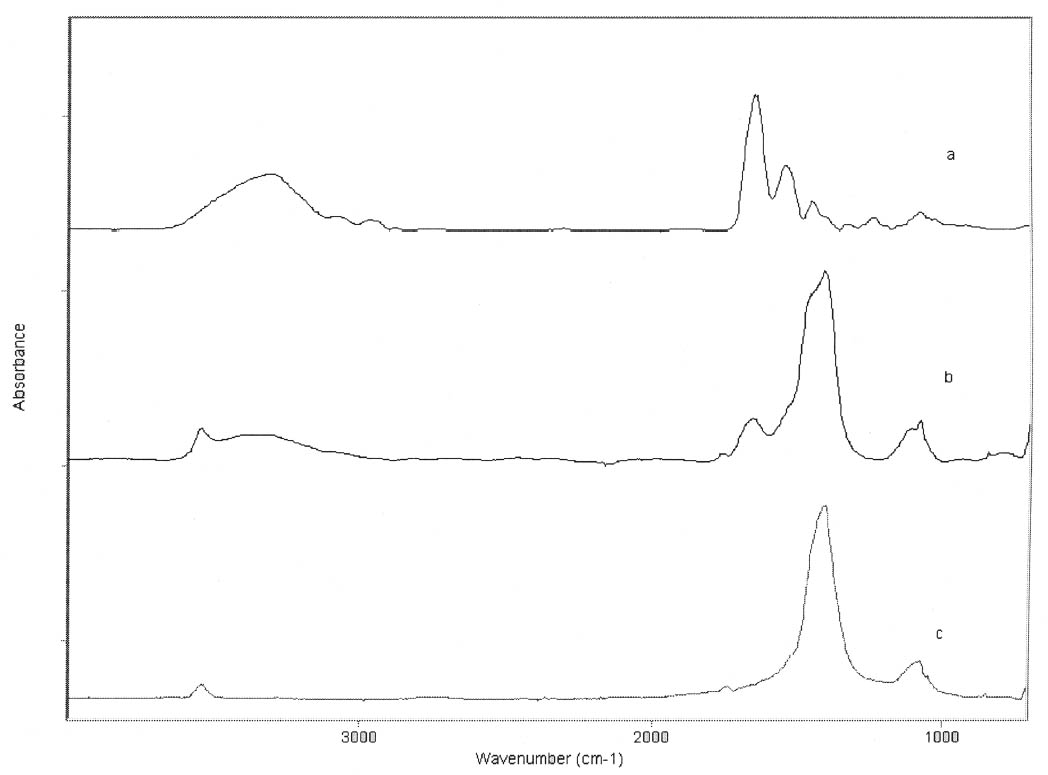 |
Table .
Amide I and Amide II Maxima Wavenumbers for Different Pure Binders and Binder-2PbCO3. Pb(OH)2 Mixtures Conditioned at 20�C and 55% RH; and Maxima Values for Pure Gelatin and Gelatin–Lead White Mixtures Conditioned at 20�C and 90% RH
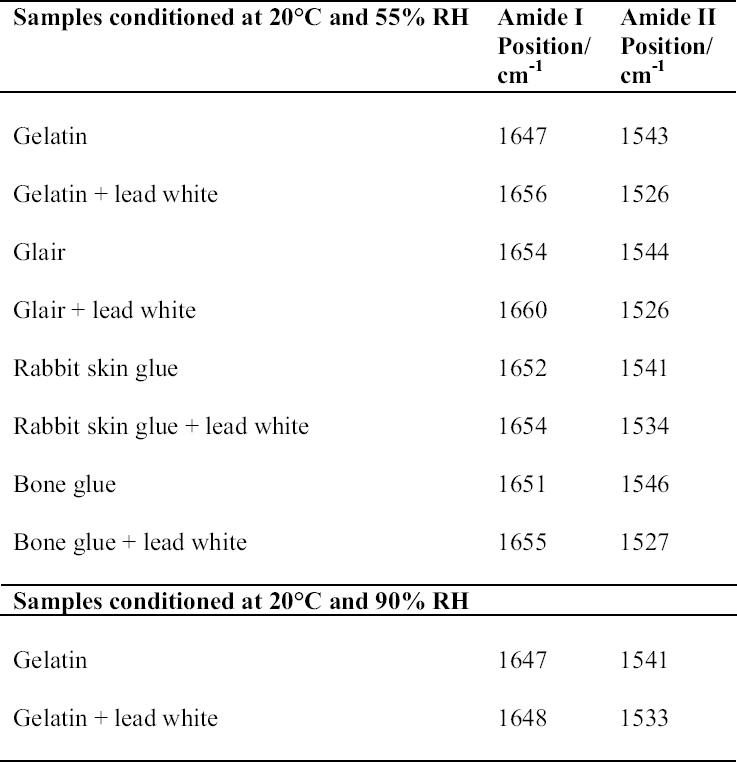 |
Fig. 2.
Detail of the FTIR spectra of gelatin (—) and gelatin mixed with lead white (——), conditioned at 20�C and 55% RH, in the 1900–1200 cm-1 spectral range (full range shown in fig. 1). Arbitrary absorbance units
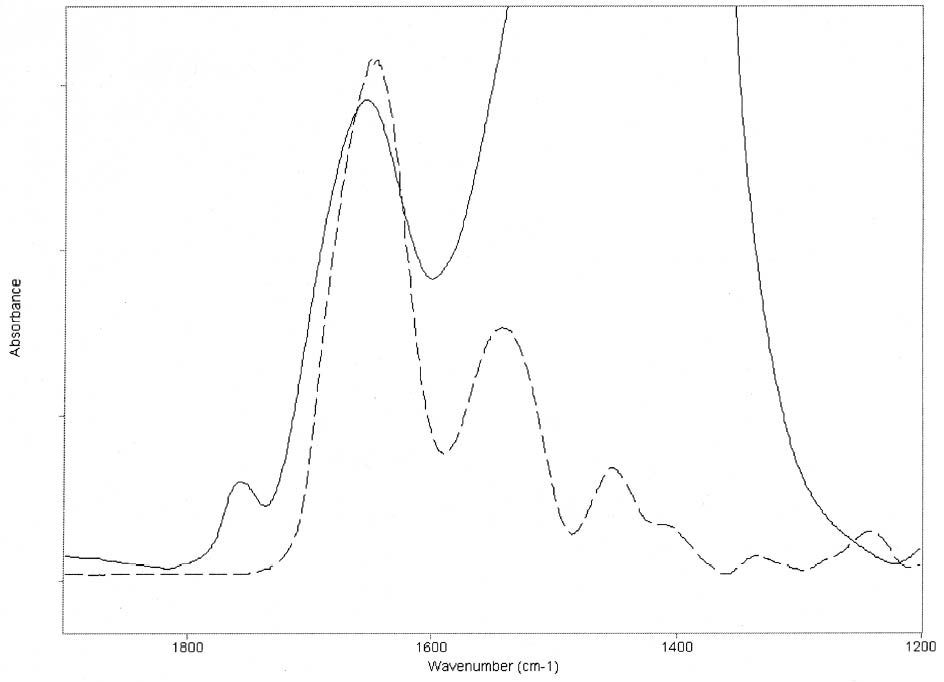 |
Fig. 3.
FTIR spectra of gelatin (a) and gelatin–lead white (b), conditioned at 20�C and 55% RH, showing major peaks obtained from the curve-fitting procedure (dotted lines, except for the 1683 (a) and 1688 cm-1 components (b), respectively, which are shown as continuous lines)
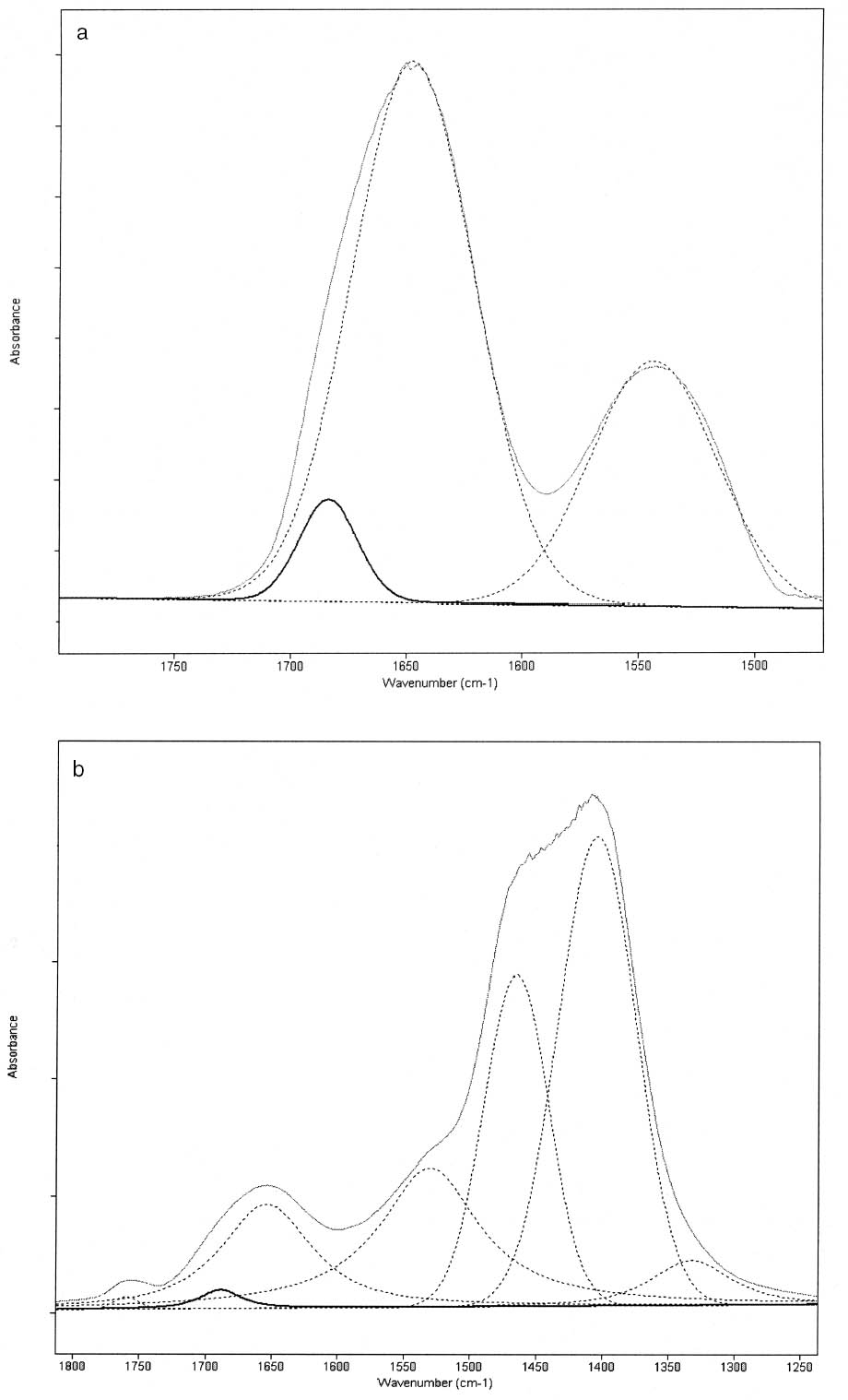 |
wavenumbers and the amide II maxima shift to higher wavenumbers, as the %RH increases. No changes in the intensities among samples conditioned at different %RH could be evaluated given the present experimental conditions. The results obtained for the gelatin–lead white mixtures are shown in figure 4.
The spectrum of the gelatin–lead white mixture originally conditioned at 20�C and 90% RH was measured again after five days of exposure to the laboratory environment (45–50% RH), and it was observed that the maxima for the amide I and amide II groups had shifted to higher and lower wavenumbers respectively (1656 and 1530 cm-1).
The FTIR spectrum of pure PbO does not present any bands in the 1700–1500 cm-1 range (not shown). For the PbO-protein mixtures studied, the amide I maxima values also shift to higher wavenumbers, while the amide II maxima remain within �3 cm-1, with respect to the values obtained for the pure binders (shown in fig. 5 for gelatin and gelatin-massi-cot). No observations on the mechanical properties of massicot-containing paint on works of art have been reported in the literature, to our knowledge.
4 DISCUSSION
The shift observed in the amide I maxima to higher wavenumbers for the mixtures with lead white, with respect to the values observed for the pure binders, when conditioned at 20�C and 55% RH, and the results obtained when the samples were exposed to a higher humidity (70% and 90% RH) indicate that lead white induces a modification in the state of hydration of the binders.
Modifications in the state of hydration of proteinaceous materials are expected to be reflected in changes in the FTIR spectra. These changes, which occur simultaneously, can be separated in two groups: (1) those that result from the influence of the hydrogen bonding of water on hydration sites such as C=O and N-H, resulting in changes in the intensities and positions of the corresponding IR bands (Pesvner and Diem 2001),
Fig. 4.
Detail of the FTIR spectra of gelatin mixed with lead white conditioned at 20�C and 90%, 70%, and 55% RH in the 1900–1200 cm-1 spectral range. Arbitrary absorbance units.
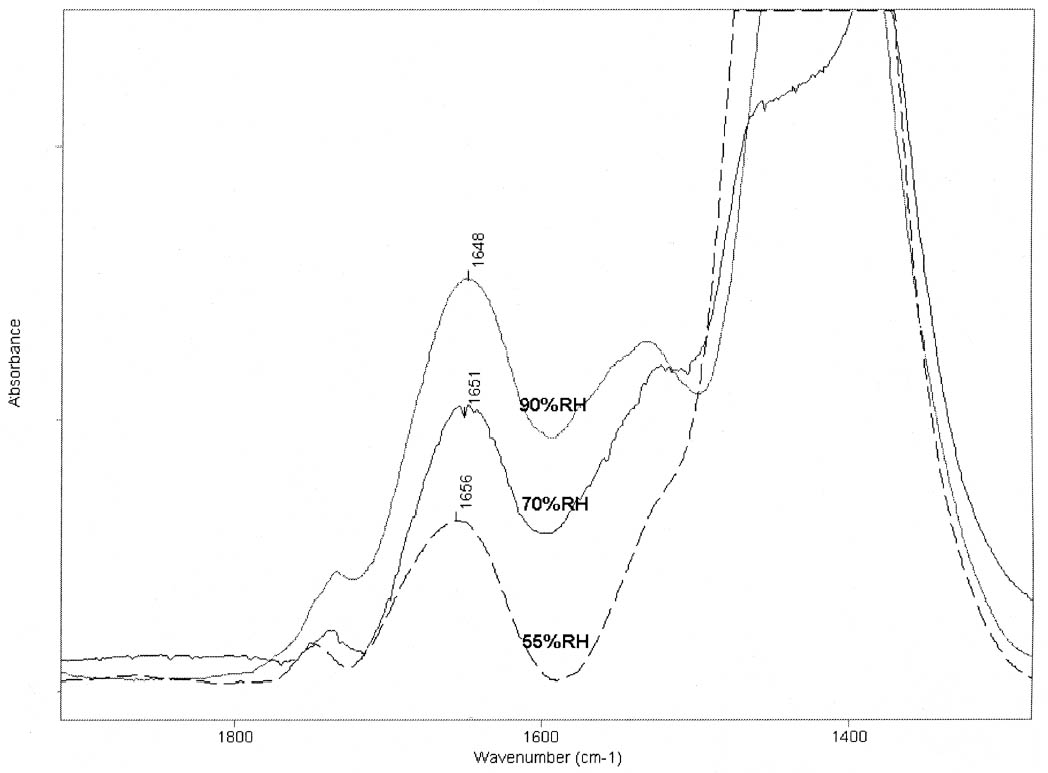 |
Fig. 5.
Detail of the FTIR spectra of gelatin (—) and gelatin mixed with massicot (——), conditioned at 20�C and 55% RH, in the 1900–1200 cm-1 spectral range. Arbitrary absorbance units
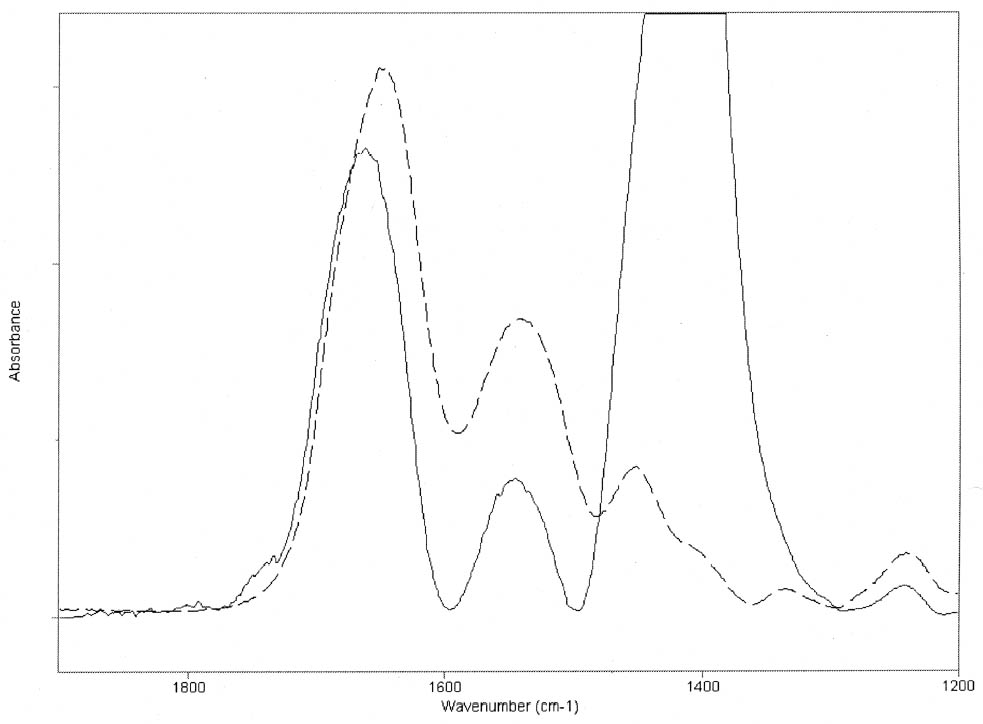 |
and (2) those due to changes in the secondary structure of the protein (Allison et al. 1998; Soulliac et al. 2002). Upon removal of water molecules from the vicinity of the peptide linkage, a shift of the amide I band to higher wavenumbers is expected as a result of the combined effect of an increase in the force constant of the C=O bond (bond strength) and a decrease in the reduced mass of the C-O vibrator in view of the decoupling with O-H. The amide II band is expected to shift to lower wavenumbers as the force constant of the C-N decreases by a decreased “stiffness” of the H-N-CO angle due to a higher “mobility” of H through a decrease in the hydrogen bonding and a decreased strength of the C-N bond. It can be concluded that lead white induces a modification in the environment around the peptide carbonyl C=O and the CNH groups, by reducing the number of surrounding water molecules and, therefore, reducing hydrogen bonding.
The results obtained for the massicot-containing mixtures indicate that this pigment also induces a change in the water structure surrounding the peptide C=O group but a much smaller change in the hydrogen bonding of the CNH group.
The dominant amide I maxima remain around the 1660–1650 cm-1 range for mixtures containing lead white or massicot, so no drastic changes in the proteins' secondary structures are taking place upon mixing with the pigments. However, the slight increase in the area of the 1683 (gelatin)/1688 (gelatin–lead white) cm-1component (see figs. 3a, 3b) relative to the amide I band area is revealing a partial conversion of α-helices to β-turns due to aggregation. No attempt was made to quantify the proportions of the different protein secondary structures due to their different molar absorptivities (De Jongh et al. 1996).
The mechanism by which lead white and massicot induce these changes in the proteins' water structure remains to be elucidated. Some salts have been demonstrated to affect the interactions within the protein through modification of the water structure (Damodaran and Kinsella 1982). Depending on the hydration energy and steric hindrance of the anions, salts may promote aggregation, unfolding, or dissociation of the protein (Meng and Ma 2001).
The results of the experiments carried out are expected to be independent of the substrate over which the paint layer is applied (canvas, parchment, paper, wood) and of the thickness of the paint layer, provided that the pigment-binder composition is uniform throughout it.
It should be taken into account that temperature and pH conditions during the manufacturing process of the binders will also affect the mechanical stability of the dried paint film. The influence of lead white on the partial hydrolysis that gelatin undergoes upon aging (Dupont 2002) and other degradation processes that may take place when the mixtures age naturally or are subject to accelerated aging under different conditions, such as the yellowing reported by Lawson and Yamazaki-Kleps (2002), are worth an in-depth separate study.
In addition, medieval treatises often recommended mixing the proteinaceous material with a glutinous or carbohydrate binder (see, for example, Merrifield 1999, 316, 156). It is well known that some carbohydrates, when added in the right proportions, form hydrogen bonds with the protein and protect it from dehydration-induced damage (Allison et al. 1998; Allison et al. 1999). The characterization of this aspect of the degradation process suggests that the addition of carbohydrates to proteinaceous materials used in consolidation treatments of lead white flaking areas may prove to be beneficial for the long-term stability of the damaged areas, though the mechanical stability and the chemical and color properties of the consolidant mixtures should be systematically evaluated first.
5 CONCLUSIONS
FTIR measurements showed that lead white reduces hydrogen bonding around the peptide C=O and N-H groups by removing water molecules and induces a small change in the protein's secondary structure due to aggregation. The water structure around the peptide linkage temporarily recovers when the samples are conditioned in a high-humidity environment (90% RH), but the low-humidity structure is restored after five days of exposure to standard humidity conditions (45–50% RH). Therefore, humidification is not enough to restore the mechanical properties of the paint films containing lead white in the long term. Massicot was found to promote a similar change as lead white around the peptide C=O but had a less drastic effect on the N-H groups.
The characterization of this aspect of the degradation process suggests that the addition of carbohydrates to proteinaceous materials used in consolidation treatments of lead white flaking areas might be beneficial for the long-term stability of the damaged areas, though the chemical and color properties of the consolidant mixtures should be systematically evaluated first.
ACKNOWLEDGEMENTS
The authors would like to acknowledge Marjorie Shelley, conservator in charge, and Margaret Lawson, associate conservator, at the Sherman Fairchild Center for Works on Paper and Photograph Conservation (MMA) for their support and helpful discussions; the A. W. Mellon Foundation for the fellowship awarded to Marcelo I. Guzman; and Dr. Masanori Sato, conservation scientist in charge, Nara National Cultural Properties Research Institute, Japan, who initiated investigations into media problems of lead white paints in aqueous binders in collaboration with AkikoYamazaki-Kleps.
REFERENCES
Allison, S. D., B.Chang, T. W.Randolph, and J. F.Carpenter. 1999. Hydrogen bonding between sugar and protein is responsible for inhibition of dehydration-induced protein unfolding. Archives of Biochemistry and Biophysics365(2):289–98.
Allison, S. D., T. W.Randolph, M. C.Manning, K.Middleton, A.Davis, and J. F.Carpenter. 1998. Effects of drying methods and additives on structure and function of actin: Mechanisms of dehydrationinduced damage and its inhibition. Archives of Biochemistry and Biophysics358(1):171–81.
Bandekar, J.1992. Amide modes and protein conformation. Biochimica et Biophysica Acta-Protein Structure and Molecular Enzymology1120(2):123–43.
Boon, J. J., J.van der Weerd, K.Keune, P.Noble, and J.Wadum. 2002. Mechanical and chemical changes in Old Master paintings: Dissolution, metal soap formation and remineralization processes in lead pigmented ground/intermediate paint layers of 17th century paintings. ICOM Committee for Conservation preprints, 13th Triennial Meeting, ed. R.Vontobel, Rio de Janeiro. London: James and James. 1:401–06.
Bykova, G. Z., A. V.Ivanova, and I. P.Mokretzova. 1976. Conservation methods for miniature painting on parchment: Treatment of the paint layer. In Conservation and restoration of pictorial art,ed. N.Brommelle and P.Smith. London: Butterworths. 207–09.
Damodaran, S., and J. E.Kinsella. 1982. Effects of ions on protein conformation and functionality. American Chemical Society Symposium Series 206. 327–57.
De Jongh, H. H. J., E.Goormaghtigh, and J.-M.Ruysschaert. 1996. The different molar absorptivities of the secondary structure types in the amide I region: An attenuated total reflection infrared study on globular proteins. Analytical Biochemistry242:95–103.
Drayman, T.1968-69. The conservation of the Petrarch manuscript. Journal of the Walters Art Gallery31–32:119–23.
Dupont, A.-L.2002. Study of the degradation of gelatin in paper upon aging using aqueous size-exclusion chromatography. Journal of Chromatography A950:113–24.
Gettens, R. J., H.K�hn, and W. T.Chase. 1993. Lead white. In Artists' pigments. A handbook of their history and characteristics, vol. 2, ed. A.Roy. Washington, D. C.: National Gallery of Art. 67–81.
Krimm, S., and J.Bandekar. 1986. Vibrational spectroscopy and conformation of peptides, polypeptides, and proteins. Advances in Protein Chemistry38:181–364.
Lawson, M., and A.Yamazaki-Kleps. 2002. Examination and conservation of the fifteenth-century parchment manuscript, The Belles Heures of Jean, Duke of Berry. In Works of art on paper, books, documents and photographs. Techniques and conservation. Contributions to the 2002 Baltimore Congress: Works of art on paper, ed. V.Daniels, A.Donnithorne, and P.Smith. London: International Institute for the Conservation of Historic and Artistic Works. 129–34.
Lin-Vien, D., N. B.Colthup, W. G.Fateley, and J. G.Grasselli, eds.1991. The handbook of infrared and Raman characteristic frequencies of organic molecules.San Diego: Academic Press.
Meilunas, R. J., J. G.Bentsen, and A.Steinberg. 1990. Analysis of aged paint binders by FTIR spectroscopy. Studies in Conservation35(1):33–51.
Meng, G.-T., and C.-Y.Ma. 2001. Fourier-transform infrared spectroscopic study of globulin from Phaseolus angularis (red bean). International Journal of Biological Macromolecules29:287–94.
Merrifield, M. P.1999. Medieval and renaissance treatises on the arts of painting: Original texts with English translations.NewYork: Dover.
Mills, J. S., and R.White. 1999. The organic chemistry of museum objects. 2nd ed.Oxford: Butterworth-Heinemann. 84–94.
Pesvner, A., and M.Diem. 2001. Infrared spectroscopic studies of major cellular components. Part I: The effect of hydration on the spectra of proteins. Applied Spectroscopy55(6):788–93.
Quandt, A. B.1992. The Butler Hours: The conservation and rebinding of a fourteenth century English book of hours. In The Institute of Paper Conservation: Conference papers, Manchester 1992, ed. S.Fairbrass. Worcester: Institute of Paper Conservation. 171–77.
Soulliac, P. O., C. R.Middaugh, and J. H.Rytting. 2002. Investigation of protein/carbohydrate interactions in the dried state. 2. Diffuse reflectance FTIR studies. International Journal of Pharmaceutics235:207–18.
Susi, H., and D. M.Byler. 1986. Resolution-enhanced Fourier-transform infrared spectroscopy of enzymes. Methods in Enzymology130:290–311.
Thompson, D. V.1956. The materials and techniques of medieval painting.New York: Dover.
Van den Berg, J. D. J., K. J.van den Berg, and J.Boon. 1999. Chemical changes in curing and aging oil paints. ICOM Committee for Conservation preprints, 12th Triennial Meeting, Lyon, ed. J.Bridgland. London: James and James. 1:248–53.
White, W. B.1974. The carbonate minerals. In The infrared spectra of minerals, ed. V. C.Farmer. London: Mineralogical Society. 268.
SOURCES OF MATERIALSLead white: 46000 Genuine Flake White, PW1 Cremnitz White Massicot: D-7971 Massicot blh Bone glue: 63000 Bone Glue, pearls Gelatin: 63040 Gelatin, sheets Rabbit skin glue: 63052 Genuine Rabbit Skin Glue Plates, from rabbit skinsKremer Pigments Inc. 228 Elizabeth St. New York, N.Y. 10012 (212) 219-2395 kremerinc@aol.com
AUTHOR INFORMATION
SILVIA A. CENTENO received a Ph.D. in chemistry from Universidad Nacional de La Plata, Argentina, in 1994. In 1995, she was awarded an L. W. Frohlich Fellowship in Conservation at the Sherman Fairchild Center for Objects Conservation, Metropolitan Museum of Art, New York, to work on the investigation of pre-Columbian metalwork from Peru. From 1997 to 2001 she continued to be involved in various research projects at the museum. In 2001 she was appointed associate research scientist at the Sherman Fairchild Center for Works on Paper and Photograph Conservation and the Sherman Fairchild Paintings Conservation Center. Her principal interests include the technical examination of works of art by nondestructive techniques. Address: Sherman Fairchild Center for Works on Paper and Photograph Conservation, Metropolitan Museum of Art, 1000 Fifth Ave., New York, N.Y. 10028; silvia.centeno@metmuseum.org
MARCELO I. GUZMAN received an M.S. degree from Facultad de Qu�mica, Universidad Nacional de Tucuman, Argentina, in 2001. In 2002, he was awarded an A.W. Mellon Fellowship to work in the Sherman Fairchild Center for Works on Paper and Photograph Conservation, Metropolitan Museum of Art, on the investigation of the interaction of lead white with binding media frequently used in medieval illuminated manuscripts. He is currently a Ph.D. student at the Environmental Sciences and Engineering Department, CalTech. Address: 7 Keck Laboratory, M/C 138–78, Pasadena, Calif. 91125; mig@its.caltech.edu
AKIKO YAMAZAKI-KLEPS earned a master of art in conservation from Camberwell College of Arts (1995). She has held internships at the National Diet Library, Japan, the Ashmolean Museum, and the Bodleian Library, Oxford. In 1994, she was the Andrew W. Mellon Fellow in Paper Conservation at the Metropolitan Museum of Art. Since 1996 Yamazaki-Kleps has been paper conservator at the Sherman Fairchild Center for Works on Paper and Photograph Conservation at the Metropolitan Museum of Art, where her responsibilities include addressing media problems on works on paper, parchment, and papyrus. In 1999, she began initial investigations into media problems of lead white paints in aqueous binders, in collaboration with Dr. Masanori Sato, conservation scientist in charge, Nara National Cultural Properties Research Institute, Japan. Her research efforts continue to focus on physical and chemical characteristics of media, binders, and supports, scientific analysis of deterioration mechanisms, and testing of consolidants and treatment methods. Address as for Centeno; akiko.yamazaki-kleps@metmuseum.org
CARLOS O. DELLA V�DOVA received his Ph.D. in chemistry from Universidad Nacional de La Plata, Argentina, in 1983 and his doctor rerum naturalium from Ruhr University, Bochum, Germany. He is the author of approximately 150 publications in peer review journals as well as books. He is currently a researcher at the National Council of Scientific and Technical Research in Argentina, Centro CEQUINOR (UNLP-CONICET), chemistry professor at the Universidad Nacional de la Plata in Argentina, director of the Laboratory for Liaison with the Industry and the Scientific System (LaSeISiC, CICUNLP-CONICET), Argentina, and director in Argentina of the Regional Program for Chemistry of the DAAD-Germany (Deutscher Akademischer Austauschdienst). His main interests are the study of small covalent molecules and their spectroscopical and physicochemical properties. Address: Departamento de Qu�mica, Facultad de Ciencias Exactas, Universidad Nacional de La Plata, Calle 47 esq. 115, (1900) La Plata, Provincia de Buenos Aires, Rep�blica Argentina; carlosdv@quimica.unlp.edu.ar
Section Index |