THE RATIONALE FOR MICROABRASIVE CLEANING: A CASE STUDY FOR HISTORIC GRANITE FROM THE PENNSYLVANIA CAPITOL
J. CHRISTOPHER FREY, & TIMOTHY NOBLE
5 PROBLEMATIC CONDITIONS PRIOR TO STUDY
Prior to study, exterior granite surfaces were characterized by a series of interrelated problematic conditions that had compromised both the appearance of the building and the microstructural integrity of many stone surfaces. While some conditions such as heavy soiling and superficial iron staining had developed as a result of the building's immediate weathering environment, other conditions such as physicochemical alteration, decreased surface permeability, and the formation of soluble salts were attributed to previous chemical cleaning treatments.
Cleaning programs had been regularly executed throughout the building's history to address heavy soiling that began to develop soon after original construction. Newspaper accounts indicate that cleaning measures were undertaken for the base of the dome as early as 1932, and for the entire building in 1936–37. Although the nature of the dome-cleaning treatment was not disclosed, the more extensive cleaning program was reported to have employed a combination of acid solutions and steam. A similar program was undertaken in 1957 and again in the late 1960s or early 1970s under a cleaning, bird-proofing, and repointing project for the building's north and south wings (Heritage Studies 1987, 502–4). Unfortunately, maintenance records that might have shed more light on the type and scope of previous cleaning treatments have been discarded, so the nature of previous treatments can only be assessed by comparing damage patterns with those that are known to have occurred in similar cases.
Some previous treatments that initially achieved visually acceptable results have since been found to play significant roles in long-term deterioration (Weaver 1993, 161). Physical evidence such as bleaching, dissolved silicates, and significantly roughened surface topography suggested that previous cleaning treatments consisted of a combination of hydrochloric acid, hydrofluoric acid, ammonium bifluoride, and perhaps localized sandblasting (Weaver 1998). Previous use of chlorine-bearing (HCl) cleaners is further suggested by the presence of chloride salts, sodium chloride (NaCl) and potassium chlorate (KCl), that were confirmed by qualitative anionic analyses in multiple locations throughout the building.
5.1 ATMOSPHERIC DEPOSITION
Located in what has historically been recognized as an area polluted by nearby railroad yards and industry, as well as by wind drift from western Pennsylvania's steel mills, the capitol building's granite walls began to exhibit heavy soiling and other deterioration within a decade after construction. Although industrial and heavy transportation emissions in the vicinity of the building had likely decreased significantly by the end of the 20th century, atmospheric deposition still was noticeable on many building surfaces. Deposition consisted primarily of dark deposits lodged both within microscopic steps and crevices on quartz and feldspar surfaces and within intergranular boundaries. Distribution was uneven, owing to poor adhesion of some grains to the smooth surfaces (Moses 1997). Heavier encrustations were present beneath the overhanging ledges of projecting elements such as cornices and belt courses, surfaces where moisture collects but that do not receive runoff during rain events (fig. 2). Six samples removed from soiled building surfaces tested positive for sulfate (SO4-) compounds using standard anionic analysis. To perform this test, samples were ground with a mortar and pestle, and the resulting powder was placed in a test tube. To the tube was added 20 cc of deionized water, and it was then shaken to dissolve soluble material. The insoluble portion of each sample was allowed to settle out at the bottom of the test tube. The resulting fluid was drained off and reacted with hydrochloric acid (HCl) and a 10% solution of barium chloride (BaCl2), forming a white precipitate in accordance with the following reaction:
(Teutonico 1988).
5.2 CHROMATIC VARIATION AND SURFACE ALTERATION
Considerable chromatic variation between stones was present even though all granite originated from the same quarry. Variation in color resulted from a number of factors, including the mineralogical composition of each stone, the effects of previously applied treatments, and differential weathering. Some variations were likely deliberate; for instance, the uniform pinkish hue of granite used for roof-level balustrades appears to have been a subtle design feature intended to call attention to this particular architectural element. The presence of darker, lighter, and differently colored stones within flat wall surfaces that were intended to appear monolithic, however, is more likely the result of unintended mineralogical variation.
The presence of severely bleached stones is consistent with the effects of inappropriately applied, overly aggressive hydrochloric acid– and hydrofluoric acid–based treatments that physically altered many surface grains and left many more susceptible to additional weathering. When applied in appropriate dilutions and with appropriate dwell times, such cleaning treatments have been found to be effective at cleaning masonry without causing damage. However, conditions at the surface of the stone prior to this study suggest that previously applied treatments may not have been properly diluted, may have been left
Fig. 2.
Heavy atmospheric deposition beneath overhangs. Photograph by authors, 1997
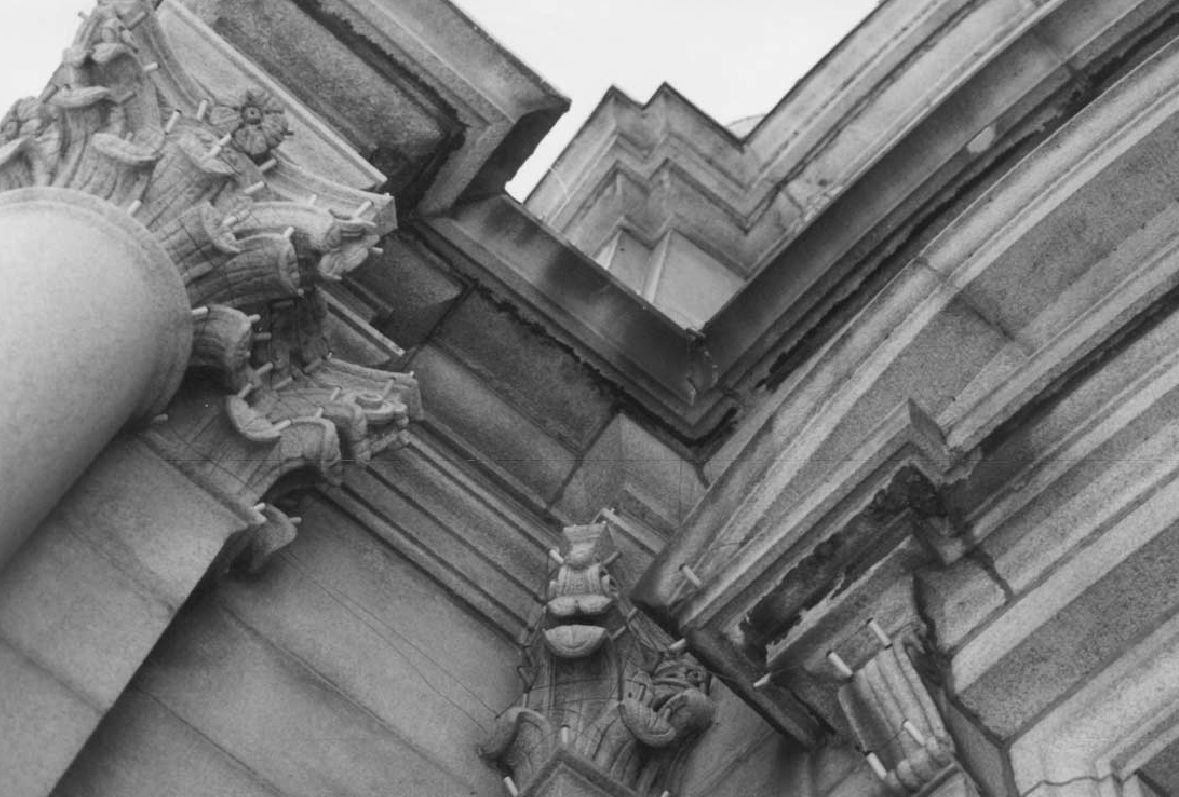 |
on the surface for an inappropriate amount of time, or may not have been completely rinsed or neutralized after application. Microscopic roughening of feldspar grains had created a chalky appearance. Quartz grains were found to possess networks of microscopic cracks that created an appearance similar to a clouded, thermally crazed glass. The effect of altered quartz was twofold; not only did it make previously transparent/translucent crystals appear somewhat opaque, the muddled surfaces prevented light from refracting behind the surface of the granite—decreasing the overall sense of depth. Biotite crystals were found to have undergone chloritization, a process that altered them from black specks into greenish, clayey minerals that have a lower degree of weather resistance (fig. 3, see page 68). The alteration of the biotite left many surfaces devoid of a compositional element that significantly contributed to the original stone's overall character and color.
The extent to which acidic treatments altered stone color was likely enhanced by the presence of fine microcracks created during the tooling process. By opening up additional avenues for the penetration of potentially deleterious chemical compounds, microcracks allowed for additional chemical exposure to subsurface quartz, feldspar, and biotite grains. Though most pronounced at the immediate surface, some samples displayed evidence of the alteration of constituent minerals to a depth of 3–5 mm, coinciding with the depth of the microcracking.
Digital chromatic assessments confirmed notable color differences between altered stone at the surface and stone directly beneath the surface that had not been subjected to weathering or treatment. While the actual readings for each sample depended in part on the specific mineralogical composition of the assessed areas, surface samples in multiple areas were found to be noticeably different from exfoliated areas, the latter of which closely matched the color of unaltered subsurface samples. On average, unaltered subsurface stone was medium gray, with a color corresponding to Munsell 2.37GY 4.08/0.46. Exposed surfaces that had been chronically exposed to weathering and chemical treatments were light olive gray, with an average color corresponding to Munsell 4.86Y 5.34/0.98. With a slightly different hue and higher value and chroma readings, exposed surfaces generally possessed a somewhat bleached yellowish appearance when compared with core specimens. While subsurface core samples may not precisely represent the original color of the building (noting that the tooled finish may have slightly affected the natural color of the stone), they were used to provide a standard reference for the stone's original chromatic values.
5.3 DECREASED SURFACE TOPOGRAPHY
Weathering and previous treatments had also substantially altered the fundamental topography of surface crystals. The weathering away of weaker minerals is a natural occurrence that explains a certain degree of loss. However, the extent of localized dissolution, erosion, and loss of detail was more consistent with chemical applications that dissolved a significant amount of surface and compromised the microstructural integrity on a localized level.
Whereas the interfingering of feldspar, quartz, and biotite crystals had originally created a highly variegated surface topography characterized by sharp, angular crystalline boundaries, exposed grains had eroded to the extent that intergranular definition was substantially altered. The end effect was a muddled surface where some grains were indistinct from one another (fig. 4) and the loss of minerals that had contributed significantly to the color and depth of the stone (biotite).
5.4 LOSS OF TOOLING PATTERNS
Loss of surface detail also extended to original tooling patterns. Serrations that most likely were originally sharp and distinct had become severely rounded, and many had even become completely flattened. The loss of tooling altered the appearance
Fig. 3.
Alteration of biotite to chlorite (arrows) prior to cleaning. RLM at 10x. Photograph by authors, 1997
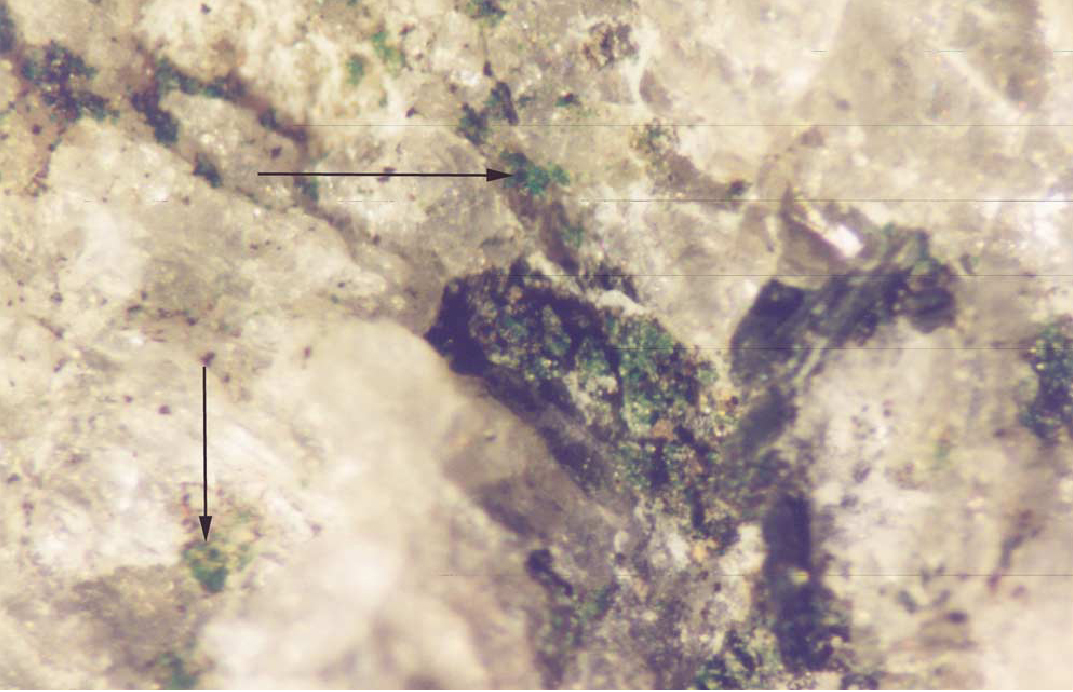 |
Fig. 4.
Heavily altered surface characterized by obscured mineral relationships. SEM at 137x. Courtesy of Artech Testing, 1997
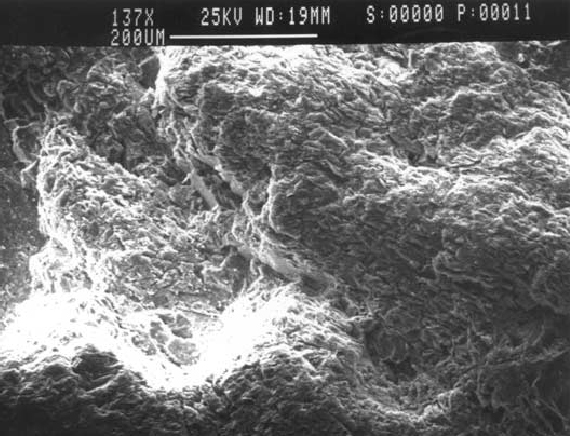 |
of the building by creating flat surfaces that were devoid of articulation.
5.5 DECREASED SURFACE PERMEABILITY
The compromised surface and 3–5 mm zone of alteration displayed evidence of decreased surface permeability compared to unaltered stone. Surface and immediate subsurface pores within the 3–5 mm zone of alteration had become clogged both by pollutant particulates and by dissolved mineral fragments, particularly fine-grained clays and chlorite that are produced by the weathering of feldspars and biotites, respectively.
Tests executed in accordance with RILEM II.4, Water Absorption Under Low Pressure, were conducted in areas where surface alteration was intact as well as in areas where the zone of alteration had been lost due to exfoliation and the unaltered subsurface had become exposed. Exfoliated surfaces absorbed greater quantities of water than surfaces where the zone of alteration was intact, further suggesting an inverse relationship between degree of alteration and surface permeability. The decreased permeability of the altered surface created a performance imbalance whereby the surface of the stone behaved differently than material beneath did. The presence of a less permeable surface created the potential for moisture-related deterioration such as exfoliation related to freeze-thaw cycles and the crystallization of soluble salts (sulfates and chlorides) that were found to be present in multiple locations. This threat was particularly problematic given the relative weakness of the compromised 3–5 mm zone of alteration, which in theory would be especially sensitive to deterioration mechanisms.
5.6 LOCALIZED IRON STAINING
SEM-EDS determined that fine-grained material present within intergranular boundaries was iron-bearing, consisting of iron oxides that most likely were either deposited on the surface before migrating into the stone or formed as certain stone constituents weathered (fig. 5). RLM and PLM further confirmed the presence of iron oxides in the form of orange-brown streaks within feldspar cleavage planes. Macroscopically, the presence of iron oxides translated to highly visible orange and yellowish-brown stains.
The presence of oxides was attributed to a number of factors, including: (1) the oxidation of iron-bearing pollutant particulates that had been carried by wind drift to building surfaces from nearby railroad yards; (2) the release and subsequent oxidation of free iron that occurs naturally with the weathering of biotite (K [Mg, Fe]3 [(OH,F)2 / AlSi3O10]) and more aggressively in the presence of hydrochloric acid (Tabasso 1988); (3) the impact of machinery and tools such as steel snow shovels against ground-level surfaces; and (4) deposition from corroding architectural fixtures, hardware, and mechanical equipment. Staining was most severe on building elements that are subject to accelerated wet-dry cycles, such as the tops of both balustrade capstones and belt courses, as well as on the entire south facade of the building's south wing. In most locations, staining was superficial; areas subjected to chronic deposition and oxidation, however, had stains that were several millimeters deep.
|