THE SWELLING OF ARTISTS' PAINTS IN ORGANIC SOLVENTS. PART 2, COMPARATIVE SWELLING POWERS OF SELECTED ORGANIC SOLVENTS AND SOLVENT MIXTURES
ALAN PHENIX
ABSTRACT—This article describes the swelling responses of two oil paint films as a consequence of immersion in solvents of various kinds. The two test paint films have the same original formulation and are based on proprietary artist's oil colors containing yellow ocher and flake white pigment bound in linseed oil: one was aged by exposure to high light dosage, and the other was unexposed. Lateral, in-plane swelling of the paint films during immersion in solvent was determined by a simple microscopical method using computer-based image analysis. Results are reported for the swelling of both paint films in more than 55 common solvents and 14 binary solvent mixtures containing ethanol. Data are presented as swelling curves of percentage change in area as a function of time and as plots of degree of maximal swelling as a function of selected solvency indicators. The results are discussed in comparison with existing data on the swelling of oil films by organic solvents and in relation to the implications for the cleaning of oil-based paints.
TITRE—Le gonflement des peintures d'artistes dans les solvants organiques. 2e partie: les aspects charact�ristiques du gonflement dans quelques solvants utilis�s seuls ou en m�lange. R�SUM�—Cet article d�crit le gonflement de deux films de peinture � l'huile suite � leur immersion dans divers solvants. Les deux films de peinture soumis aux tests ont la m�me formulation de base et ont �t� fabriqu�s � partir de peintures � l'huile industrielles pour artistes contenant comme pigments de l'ocre jaune et du blanc de plomb li�s dans de l'huile de lin. L'un des films a �t� artificiellement vieilli par exposition � des niveaux de lumi�re intenses, tandis que l'autre n'y a pas �t� expos�. Le gonflement dans le plan pour les films de peinture suite � leur immersion dans un solvant a �t� d�termin� par une simple m�thode microscopique utilisant l'analyse d'images sur ordinateur. Les r�sultats rapport�s montrent le gonflement des deux films de peinture dans plus de 55 solvants couramment utilis�s et 14 m�langes de solvants binaires contenant de l'�thanol. Les donn�es sont pr�sent�es sous forme de courbes de gonflement indiquant le pourcentage de changement de dimension en fonction du temps �coul� et sous forme de diagrammes indiquant le degr� de gonflement maximal en fonction des indicateurs de solvabilit� choisis. Les r�sultats sont compar�s avec des donn�es existantes sur le gonflement des films de peinture � l'huile caus� par les solvants organiques. On y traite aussi des cons�quences de l'utilisation de ces solvants lors du nettoyage des peintures � l'huile.
TITULO—Dilataci�n en solventes org�nicos de pigmentos para artista, Parte 2: Comparaci�n de la capacidad de dilataci�n de solventes org�nicos selectos y mezclas de solventes. RESUMEN—Este art�culo describe las respuestas de dilataci�n de dos pel�culas de �leo, como consecuencia de su inmersi�n en solventes de varios tipos. Las dos pel�culas de pintura tienen la misma f�rmula original y est�n basadas en colores de fabricaci�n comercial para artistas, que contienen pigmentos amarillo ocre y blanco de plomo aglutinados en aceite de linaza: una fue envejecida por exposici�n a altas dosis de iluminaci�n, y la otra no fue expuesta. La dilataci�n lateral planar de las pel�culas de pintura durante la inmersi�n en solvente fue determinada mediante un m�todo microsc�pico simple, usando an�lisis computarizado de im�genes. Se dan los resultados de la dilataci�n de ambas pel�culas de pintura, en m�s de 55 solventes comunes y 14 mezclas de solventes binarios que contienen etanol. Los datos son presentados como curvas de dilataci�n de cambio porcentual por �rea en funci�n del tiempo y como gr�ficas de grado m�ximo de dilataci�n en funci�n de indicadores de solvencia seleccionados. Los resultados son discutidos en comparaci�n con los datos existentes sobre dilataci�n de pel�culas al �leo causada por solventes org�nicos, y en relaci�n con las implicaciones para la limpieza de pinturas en base a �leo.
1 INTRODUCTION: BACKGROUND AND CONTEXT
At the first IIC congress in Rome in 1961, Nathan Stolow presented a distillation of his doctoral research work, completed a few years earlier at the Courtauld Institute, on the effects of organic solvents on pigmented and unpigmented films of linseed oil, in the context of the cleaning of paintings (Stolow 1955, 1963). Stolow's findings, ultimately compiled in his chapter of On Picture Varnishes and Their Solvents, remain possibly the most important source that describes the action of solvents on oil paints (Feller et al. 1985). Stolow investigated two principal effects that were understood to contribute to risk in cleaning with solvents: the physical swelling of the organic binder phase caused by sorption of solvent, and leaching, i.e., the extraction of soluble, low molecular weight components of the organic binder phase.
Perhaps partly because the problem is amenable to investigation by conventional techniques of analytical organic chemistry (gas chromatography, gas chromatography—mass spectroscopy, liquid chromatography—gas chromatography, etc.), the issue of leaching of paint films by organic solvents has received a fair amount of research attention since Stolow's pioneering gas chromatographic investigations of the 1960s (Stolow 1965). This aspect of risk in cleaning is rightly continuing as an important theme in the theoretical investigation of the cleaning process (Erhardt and Tsang 1990; White and Roy 1998; Sutherland and Shibayama 1999; Tumosa et al. 1999). However, in the practical situation of actually removing varnish from a picture with organic solvents, it is arguable whether leaching can ever be anything more than a notional concern, for it is unlikely that a conservator can actually sense if organic molecules are being extracted during the cleaning process. At the present time there is no simple test the practical conservator may perform to detect or quantify extracted compounds at the point of cleaning. In the practice of cleaning, then, the magnitude of risk associated with leaching is extremely difficult, if not impossible, for the conservator to gauge. The constitution of the paint and its environmental and treatment histories will all have a marked influence on the presence, or not, of an abundant solvent-extractable organic phase. Paint films of different types have been shown to vary appreciably in their degrees of extractability.
From the point of view of the practical conservator, it could be argued that the most directly useful parts of Stolow's research are his investigations of the swelling power of various organic solvents on reference paint films. Swelling is a real, tangible concern in the practice of cleaning paintings. If swollen to a significant degree due to sorption of solvent, the paint medium has a reduced binding power and the pigment is vulnerable to removal by the mechanical action of the cleaning swab applied to the surface. The preliminary cleaning tests routinely conducted by conservators, such as Ruhemann's Safety Margin Test, are essentially directed toward assessing and controlling the risk presented by swelling of the paint set against the solubility of the varnish (Ruhemann 1968). The degree of equilibrium swelling was shown by Stolow to be an indicator of the magnitude of solvation interaction between a liquid and the organic phase of the paint. A key finding was the variation in the degree of swelling of reference paint films by organic solvents in relation to their Hilde-brand solubility parameter ∂ (Stolow 1963, 1976). Solvents and solvent mixtures of intermediate polarity, i.e., those having, for example, ∂ values in the range ca. 8.8–11 (cal/cm3)1/2 [18–22.5 MPa1/2], produced the greatest degrees of swelling. This observation is comparable with the results of the very few other paint swelling studies that exist in the paint technology literature, notably Browne (1956), Brunt (1964), and Eissler and Princen (1968, 1970).
The application of oil paint swelling data to actual cleaning practice owes much to later interpretations using more sophisticated treatments of the solubility parameter element. Of particular importance is the work of Hedley (1980), who took some of Stolow's published swelling data and combined it with the empirical approach of Ruhemann (1968) to develop a broad framework for solvent selection in varnish removal. Using the Teas fractional solubility parameter chart, Hedley identified for oil paint a peak swelling region, which represented a zone of solvent power associated with increased risk in varnish removal. This approach was developed further by Michalski (1990). Despite shortcomings in the Teas fractional solubility parameter approach, which are now well established (Blank and Stavroudis 1989; Michalski 1990; Phenix 1998b), there is no doubt that these interpretations have been valuable, influential contributions to the technical and theoretical foundations of solvent cleaning (Banik and Krist 1984; Stoner 1994; Cremonesi 2000). It must be emphasized, however, that they are constrained by the limitations of the swelling data on which they are based. These limitations are significant, and it is perhaps now appropriate to reconsider the validity of the Hedley-Stolow peak swelling region.
2 REASSESSING THE HEDLEYSTOLOW PEAK SWELLING REGION
As Ruhemann (1968) clearly recognized, there are difficulties in correlating the results of experimental swelling studies with the practice of cleaning old paintings. These difficulties derive from the nature of paint films that have been tested. All the available swelling data relate to young paint films, less than 25 years old: and, within conservation, swelling data on paint films composed of linseed stand oil have been presented most widely. Such films are likely to be significantly different in chemical character from those of old paintings. Both the comparatively young age and the choice of stand oil as binder suggest that existing data may significantly underestimate the response of old paint films to solvents at the more polar end of the spectrum, particularly to those that are strongly dipolar or engage in hydrogen bonding. Dried films of stand oil are known to be considerably less oxygenated than those of blown or boiled oils or simple linseed oil dried normally in air (Mills and White 1999). For example, according to Stolow's data on the lead white—stand oil films, ethanol is a distinctly low swelling solvent comparable in effect with the aliphatic hydrocarbons. However, the practical conservator learns very quickly that, in the order of things, ethanol can have a moderately strong effect on paint. It might be expected that the swelling regions of old oil paint films are shifted toward the direction of higher polarity. Practical experience of cleaning also suggests that, in many cases, paint films can be vulnerable to solvents and solvent mixtures over a much broader range than indicated by the Hedley-Stolow peak swelling region, particularly on the polar side.
By basing his peak swelling region only on Stolow's lead white-stand oil swelling data (Feller et al. 1971), Hedley inadvertently carried this limitation over into his Teas chart treatment. While acknowledging its continuing value as a guide to solvent usage in cleaning, we must now perhaps face the fact that Hedley's solubility parameter framework is constrained by its reliance on young stand oil paint films as the model of oil paint behavior. There is strong evidence that this kind of film has distinctive properties. Stolow, for example, also presented data on the swelling of other linseed oil film types as a function of solvent solubility parameter, ∂ (Stolow 1955, 199–200a; 1957, 399). What these plots reveal is the anomalous behavior of the stand oil film: all the other films show significant degrees of swelling caused by the polar solvents including the alcohols. Stolow accepted that “on the whole, stand oil films behaved somewhat differently from non-stand oil films” (Stolow 1955, 201).
The different swelling behavior of stand oil compared to other linseed oil films is shown schematically in figure 1. These differences were attributed to the solubility parameter properties of the solvents rather than to the internal chemistry of the paint films. The attraction of the swelling data on the stand oil paint films may have been the relative symmetry in the curves of swelling vs. ∂, which complied with the idea of a Gaussian function (Stolow 1955, 198). Also interesting in Stolow's swelling data for n-alcohols with stand oil is the variation in degree of swelling with molecular size: the larger the molecule, the greater degree of swelling measured, a finding that is rather at odds with conventional wisdom that, all other things being equal, smaller, more compact molecules cause greater degrees of swelling (Hansen 2000, 19). The lower-swelling power of iso-and sec-alcohols compared to corresponding n-isomers is also indicated in Stolow's research. The high-swelling effects known by practical conservators of strongly dipolar solvents, such as N, N-dimethylformamide (∂ =12.1 (cal/cm3)1/2), are also difficult to rationalize with the Stolow-Hedley peak swelling region.
Fig. 1.
Schematic representation of different swelling behavior of stand oil films compared to other linseed oil films, from data in Stolow 1955, Stolow
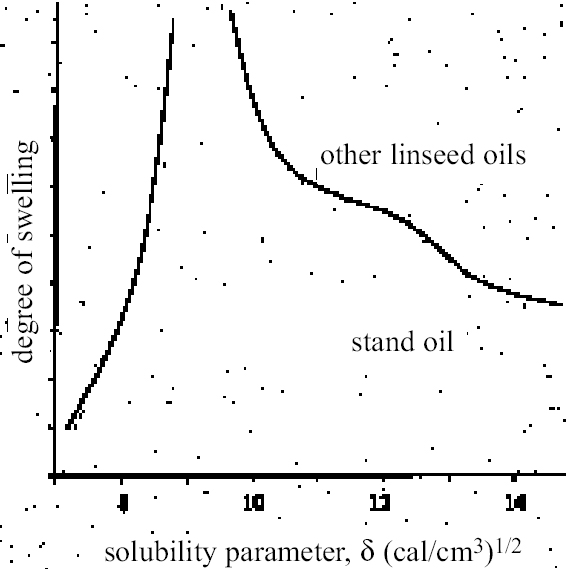 |
There are other reasons to believe that old oil paint films may be more strongly affected by polar solvents than implied by the data of Stolow (1976) or by the interpretation of Hedley (1980), and these derive from recent advances in understanding of the chemistry of deterioration processes in oil paints. There is now good evidence to suggest that real, old paint films are significantly different in their internal chemistry from the young paint films that have formed the basis of most scientific studies of the cleaning process. The methodological problems this evidence has presented in relation to scientific investigations of cleaning have been discussed elsewhere (Phenix 1998a). That oil paint films become more polar on aging, and consequently become increasingly sensitive to polar solvents, including water, has been recognized for many years (Frilette 1946; Elm 1949). Explaining the increased tendency of old (oil-modified alkyd) paint films to be plasticized by water, Brunt (1962) noted that “the oxidative drying process results in the building of a large number of hydrophilic groups because the acid number greatly increases. It is very probable that these polar groups are responsible for the sensitivity to water. A small quantity of water gives much plastification.”
Oxidation is not the only aging process that may contribute to the increased polarity of the organic phase of old oil paint films. Recent research on curing and aging of oil paint films points to hydrolysis (or de-esterification) of the glyceride ester polymer network also as possibly a significant factor in the chemical alteration of oil paint binder due to aging (Schilling et al. 1997, 1999; van den Berg et al. 1999; Erhardt et al. 2000). If this is the case, then de-esterification/hydrolysis would result in quite marked changes in the internal cohesive chemistry of paint films. De-esterification/hydrolysis of the cross-linked polyester network of a dried, cured oil paint film leads to the formation of a residual cross-linked polymeric fraction that contains carboxylate groups, plus low molecular weight, mobile compounds (free fatty acids, diacids, and glycerol); and all of these substances are comparatively polar. The presence of hydroxy functional groups in long-chain fatty acids, for example, is known to increase significantly their solubility in polar solvents; and, by the same principle, fatty acid monoglycerides have greater solubility in polar solvents than diglycerides, which in turn have greater polar solubility than triglycerides (Schmid 1973).
The increased ionic character of the residual polymer phase proposed in this scheme is particularly significant. Coordination between anionic groups on the polymer with multivalent metal ions, either at pigment surfaces or dissolved in the organic phase, is suggested as contributing significantly to cohesion of the paint film, increasing compactness and stiffness. Aging of paint films will, by both oxidation and/or hydrolysis, lead to increased polarity in the paint film and to the increasing anionic functionality of the paint binder. The formation of oxygenated functional groups (ethers, peroxy cross-links, hydroxyls, carbonyls, etc.) into both the stationary and mobile phases will inevitably increase sensitivity of the organic phase to polar and hydrogen-bonding solvents. Since metal ion—medium interactions would contribute strongly to the overall cohesion of the paint film, these liquids might also disrupt paint film cohesion and pigment binding by interfering with the carboxylate—metal ion interaction, in addition to any solvation or swelling effect on the organic binder phase. Ion-dipole and ionic interactions between paint and solvent may become increasingly important in governing the response of paint films to cleaning liquids as they increase in age or state of deterioration.
The mass of the organic phase of the paint film is known to diminish significantly as a consequence of aging, through loss of volatile scission products and molecules not immobilized in the polymer network. In combination with the increased cohesiveness conferred by internal electrostatic and H-bonding forces, this effect may result in severely aged paint films showing only low magnitudes of swelling in solvents compared to their young counterparts. It is conceivable that solvent sorption may cause the cohesion of old paint films to be interfered with by processes additional to, and more chemically complex than, the simple solvation swelling of the organic binder phase. Swelling—that is, gelation, softening and physical enlargement of the organic binder phase—may be a phenomenon of risk in cleaning that is most pertinent to relatively young or mediumrich oil paints. In the long run it may be that alternative investigative approaches will be necessary to examine properly the influence of solvents on the cohesion of paints that are appreciably aged.
For the moment, however, there is value in reexamining the solvent-induced swelling behavior of typical young-mature oil paint films to clarify their sensitivities to solvents of various types. It is hoped that the results will help in providing a better understanding of paint-solvent interactions and will contribute to an improved theoretical framework for selecting solvents in the cleaning of paintings. Essentially, the goal is to provide a broader range of scientific evidence that will improve the precision with which practical conservators might deploy these chemical tools in execution of the craft of cleaning paintings. The primary objective has been to measure the degree and rate of swelling of selected paint films when immersed in a range of organic solvents repre-sentative of those commonly used in the cleaning of paintings and of specific chemical classes.
3 EXPERIMENTAL METHOD
The method used for measuring the swelling of artist's paints during immersion in solvents is that reported elsewhere in this journal (Phenix 2002).
3.1 PAINT FILMS
The results reported here relate to just two paint films from the group described in Phenix (2002), namely samples 16 and 17. These are paint films prepared in 1991 essentially from proprietary oil paints (Winsor & Newton Artists' Oil Colours), with added dry pigment to increase the pigment volume concentration. They are composed identically as follows: yellow ocher oil paint (3 parts by weight) + flake white oil paint (3 parts by weight) + yellow ocher dry pigment (1 part by weight) + flake white dry pigment (1 part by weight). The paints were cast as uniform films on polyester film and allowed to dry for several weeks. Both sets of samples 16 and 17 were then housed in a purpose-built light-aging apparatus for approximately 18 months (Phenix 2002). Paint film 17 was exposed to UV-filtered daylight (estimated at 50–70 Mlux hours), while 16 was not exposed to light, being completely covered with aluminium foil for the period in the light-aging chamber. Paint films 16 and 17 are, therefore, identical except that 17 has had a substantial period of light exposure. They are now 140 μm and 130 μm thick respectively.
Organic chemical analysis by gas chromatography– mass spectroscopy was conducted to characterize the nature of the paint samples and of soluble, extractable components (Sutherland 2000). The analyses were conducted following protocols established specifically for the quantification of organic compounds extractable from oil paints (Sutherland 2001, 89, 141–57). A summary of results is compiled in table 1. Both paint films 16 and 17 show a high proportion of oleic acid, indicating that, even though 17 has been exposed to light-aging, drying/oxidation has not progressed very far. These might be classed as youngmature paint films. The palmitate: stearate (P:S) ratios of 1:9 are typical for linseed oil. The azelate: palmitate (A:P) ratios differ slightly, with the light-aged sample 17 showing a slightly higher A:P ratio than 16, which is consistent with the higher degree of oxidation one would expect in a light-aged sample. Similarly, the oleate: palmitate ratio for 17 (0:9) is lower than that for 16 (1:1).
Interestingly, both paint films were shown by analysis to have relatively low overall solubility. In fact, these samples were originally selected for intensive study partly because they tended to produce less variable results than other paint films on account of their lower sensitivity to leaching. The low abundance of soluble components compared to other young paint films probably derives from the period in the aging apparatus where elevated temperatures (possibly exceeding 40�C) will have encouraged volatilization of low molecular weight species (Erhardt et al. 2000). The two paint films are more or less similar in terms of solubility: the additional lightaging experienced by 17 appears to have had only minor effect. The greater solubility of both samples in xylene compared to ethanol is slightly unusual (Sutherland 2000).
Table .
Summary of Gas Chromatography—Mass Spectroscopy Analysis on Yellow Ocher—Flake White Oil Test Paint Films 16 and 17
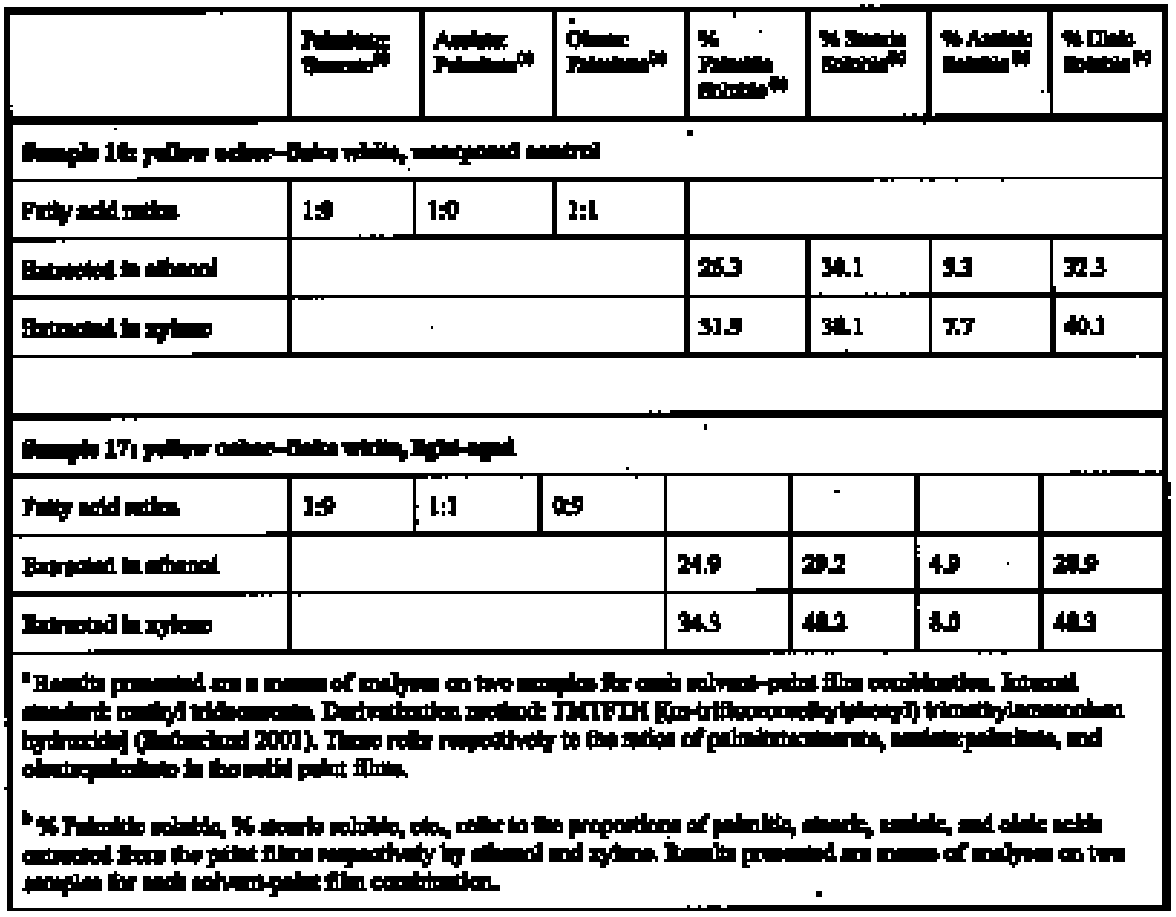 |
As reported previously, the method employed here allows the swelling of several paint samples to be measured simultaneously. Results for a group of samples of the same type in any given solvent are combined to produce a mean swelling curve. The sample sets from which the mean swelling curves are derived vary in size depending on the variability of results and the number of repeat experiments required to reduce the standard error to an acceptable level.1 For the results reported here, the number of samples of each paint film in a particular solvent range from a minimum of 3 to a maximum of 18, with 4 or 5 being the most usual.
3.2 SOLVENTS
For the most part, the test liquids used in this study came from newly purchased batches of analytical grade or general-purpose grade solvent. In a few instances, swelling experiments were carried out using existing stock available at the Courtauld Institute.
4 RESULTS AND DISCUSSION
4.1 SWELLING BY INDIVIDUAL SOLVENTS
Representative mean swelling curves for the two paint films, 16 and 17, during immersion in various organic solvents are shown in figures 2–8. The solvents are organized according to chemical class (hydrocarbons, ethers, esters, etc.). The first graph in each pair (figs. 2a, 3a, etc.) relates to paint film 16 and the second (figs. 2b, 3b, etc.) to paint film 17. Table 2 comprises, for both paint films 16 and 17, a compilation of data on the maximum values of swelling and on the rates of swelling, the latter property being defined by the time to half maximal swelling, t1/2. Table 2 contains values for various indicators of solvent polarity and serves also as the key to figures 10–11.
There are many possible indicators of solvent polarity, either physical properties such as dipole moment (μ) and relative permittivity/dielectric constant (∊), or empirical parameters (Phenix 1998b). Most of the empirical parameters are derived from solvatochromic measurements. The Reichardt normalized polarity value ETN is now widely accepted as one of the more viable empirical indicators of solvent polarity (Marcus 1999). One of its principal shortcomings, however, is its apparent tendency to overestimate the polarity of any compound with an –OH group in it. The other main established solvatochromic indicator is the π* parameter of Kamlet et al. (1983). This indicator measures a blend of polarity and polarizability and was established with the intention of providing a quantitative empirical measure of the nonspecific part of van der Waals interactions, that is, contributions other than hydrogen bonding, which is covered by two other parameters, α (hydrogen bonding acidity) and β (hydrogen bonding basicity) (Laurence et al. 1994; Marcus 1999). While there is some general correlation between ETN and π*, there are some notable anomalies in the values for certain solvents, most of which are attributed to different responses to solvent polarizability effects.
Comparison of the whole body of swelling data for the two paint types demonstrates that the lightaged sample, 17, generally swells more slowly and to a lower degree than 16, its unexposed equivalent. This pattern is found with virtually all solvents and is presumably due to several factors: increased paint density in the light-exposed samples, lower organic content, and, possibly, higher cross-link density. The possibility cannot be ruled out, also, that the presence of a mobile, soluble phase within the organic binder actively enhances solvent penetration in the paint binder network. Correlation of the t1/2 values for paints 16 and 17 indicates that, in general, the rate of swelling of paint 16 is of the order of twice that of 17 (fig. 9). A number of swelling curves show a marked decline in the sample area after an initial swelling peak. It is believed that this finding indicates an appreciable degree of leaching connected with swelling, as described by Stolow (1963). The absence of a distinct peak in the swelling curve does not, however, necessarily mean that leaching is not occurring to a significant extent.
It is immediately apparent from the data in figures 2–8 that, as would be expected, solvents vary significantly both in the rate at which they cause swelling and in their ultimate swelling power. It appears, however, that the relationships of swelling power to solvent polarity and molecular size are rather more complex than indicated by previous analyses. Plotting the data on maximum values of swelling for paint film 16 as a function of solubility parameter ∂, as in figure 10, gives a pattern that has superficial similarities with the findings of Stolow (1976). Solvents of low-solubility parameter, such as
Fig. 2.
Representative swelling curves of paint films 16 (a) and 17 (b) in hydrocarbon solvents
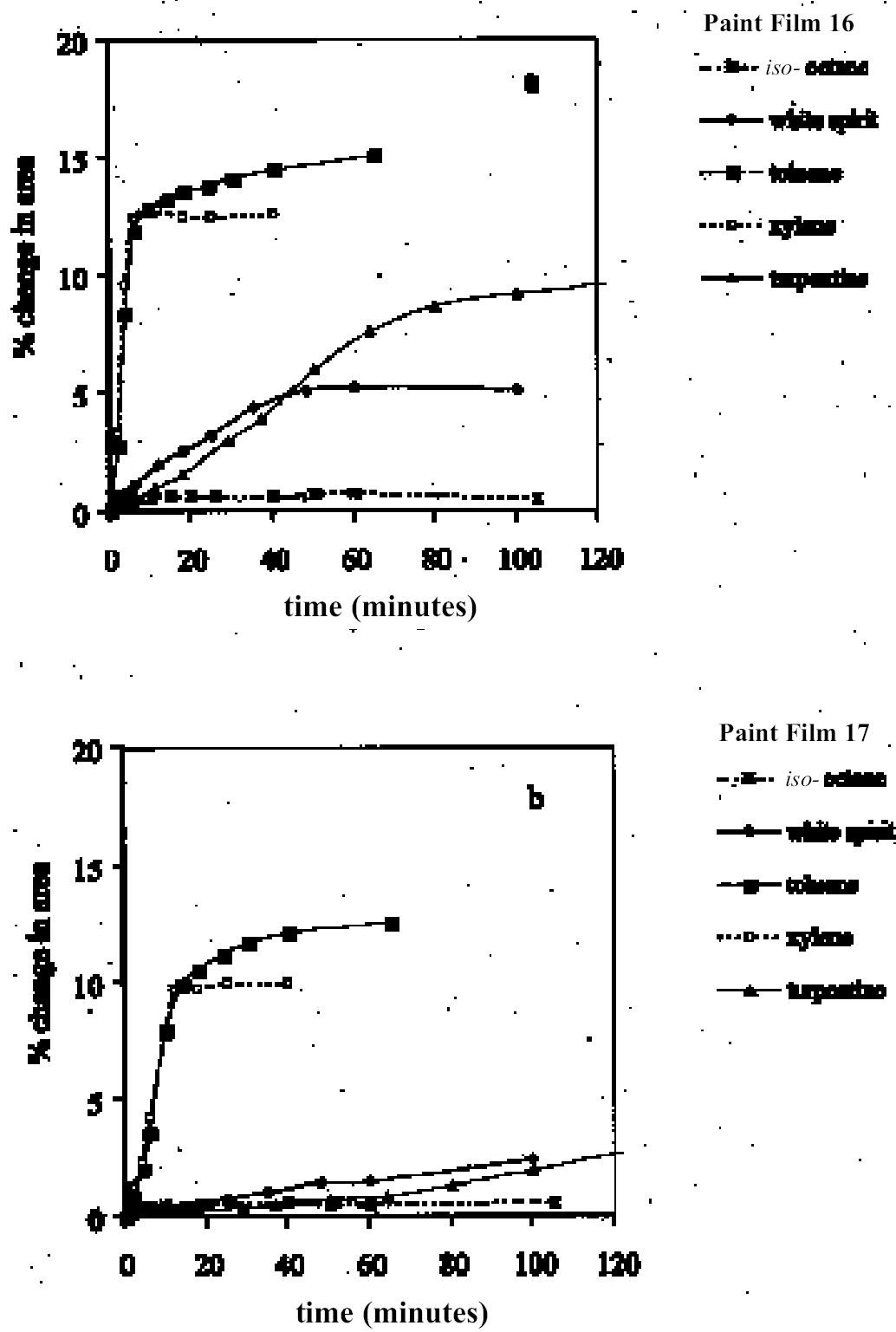 |
Fig. 3.
Representative swelling curves of paint films 16 (a) and 17 (b) in ether and ether-alcohol solvents
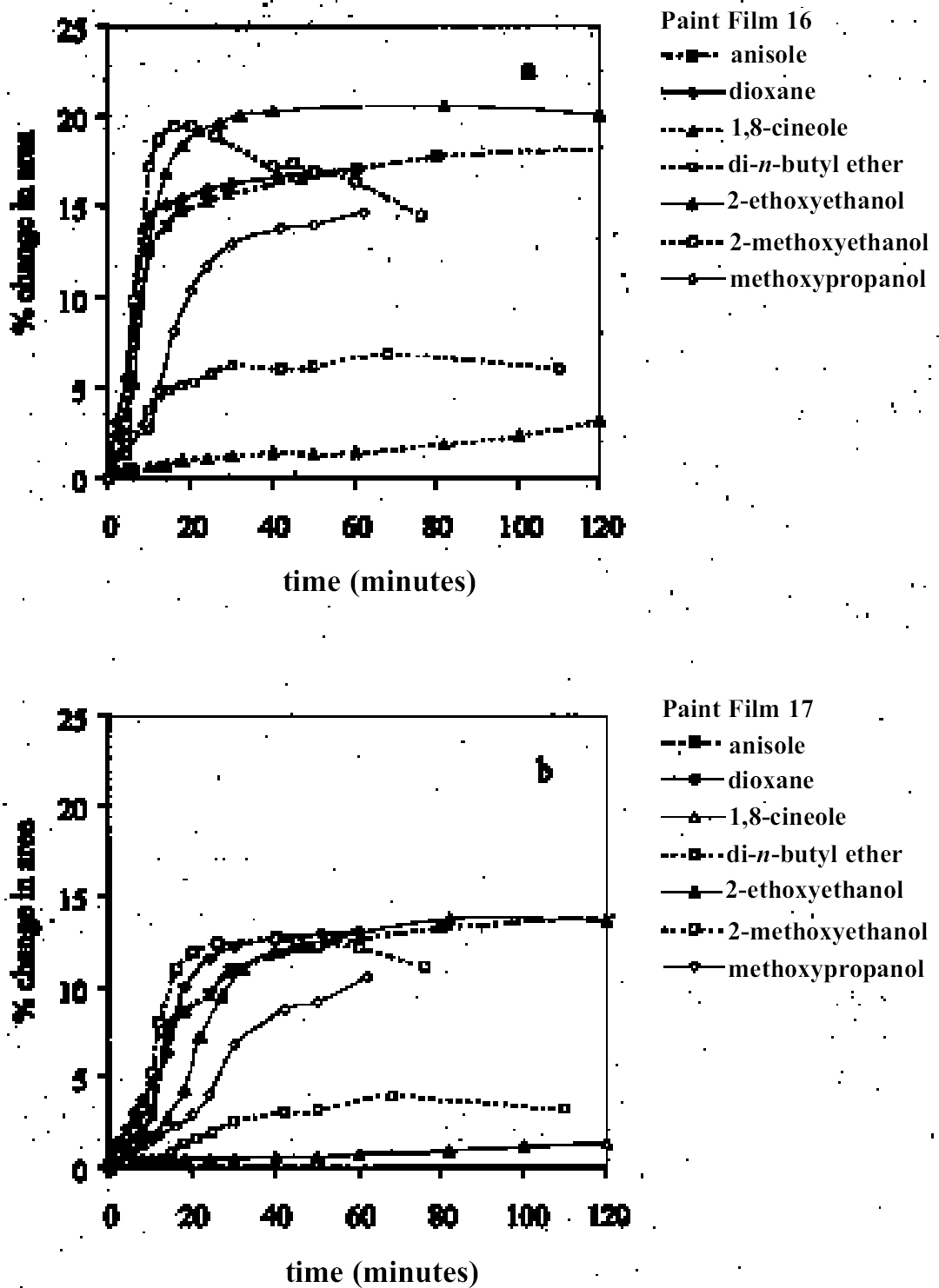 |
Fig. 4.
Representative swelling curves of paint films 16 (a) and 17 (b) in halogenated solvents
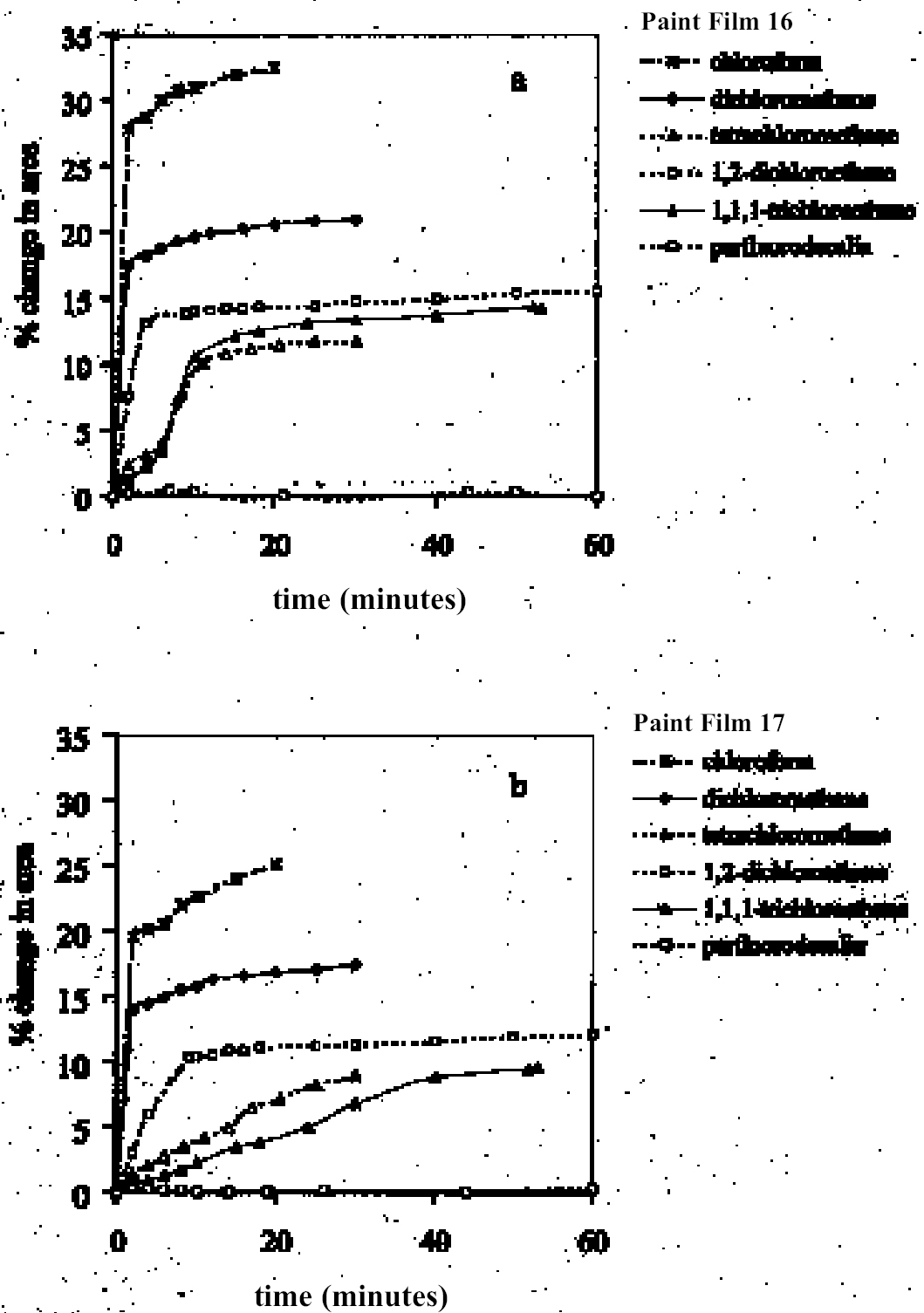 |
Fig. 5.
Representative swelling curves of paint films 16 (a) and 17 (b) in esters and related solvents
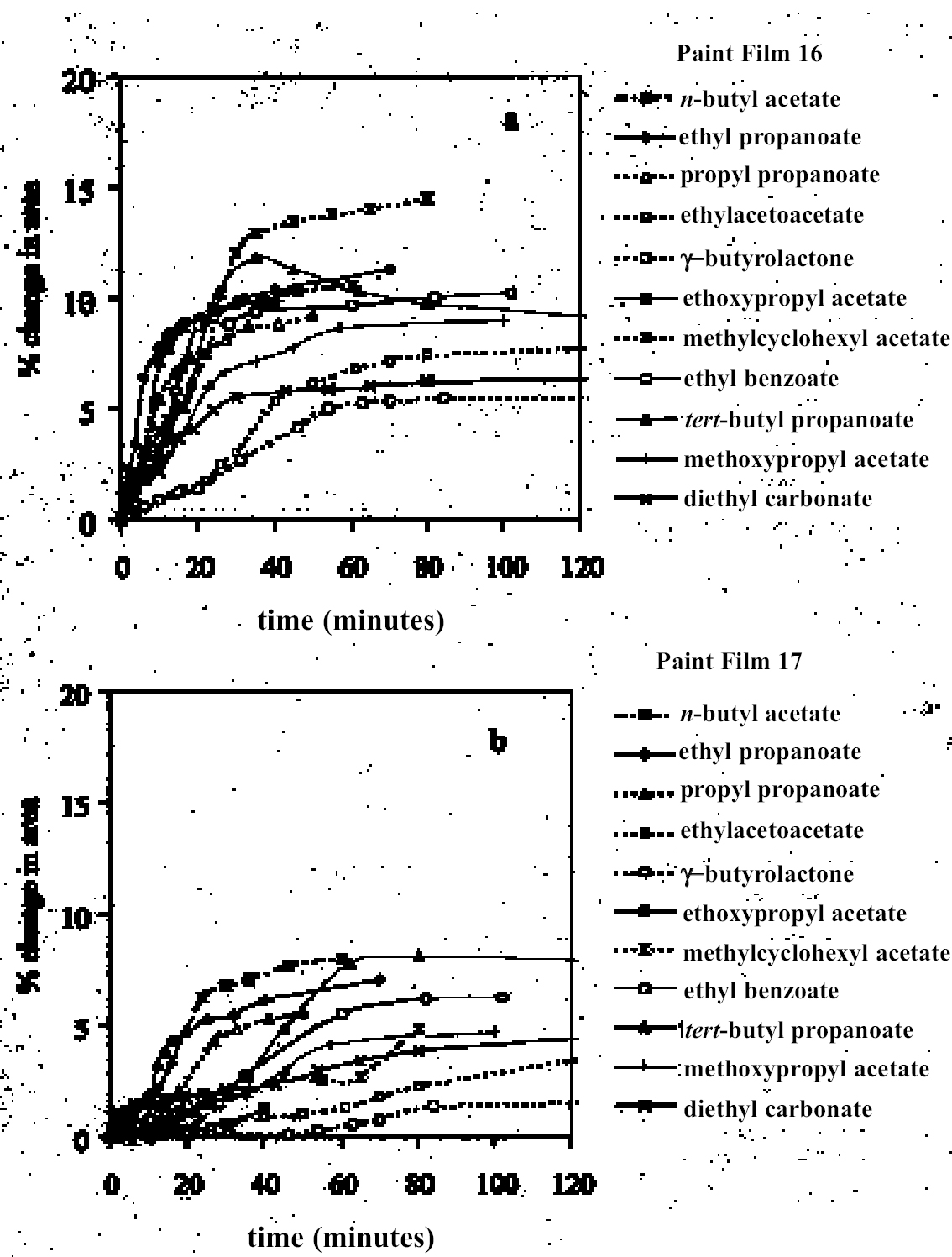 |
Table .
Compilation of Swelling Data for Paints Films 16 and 17
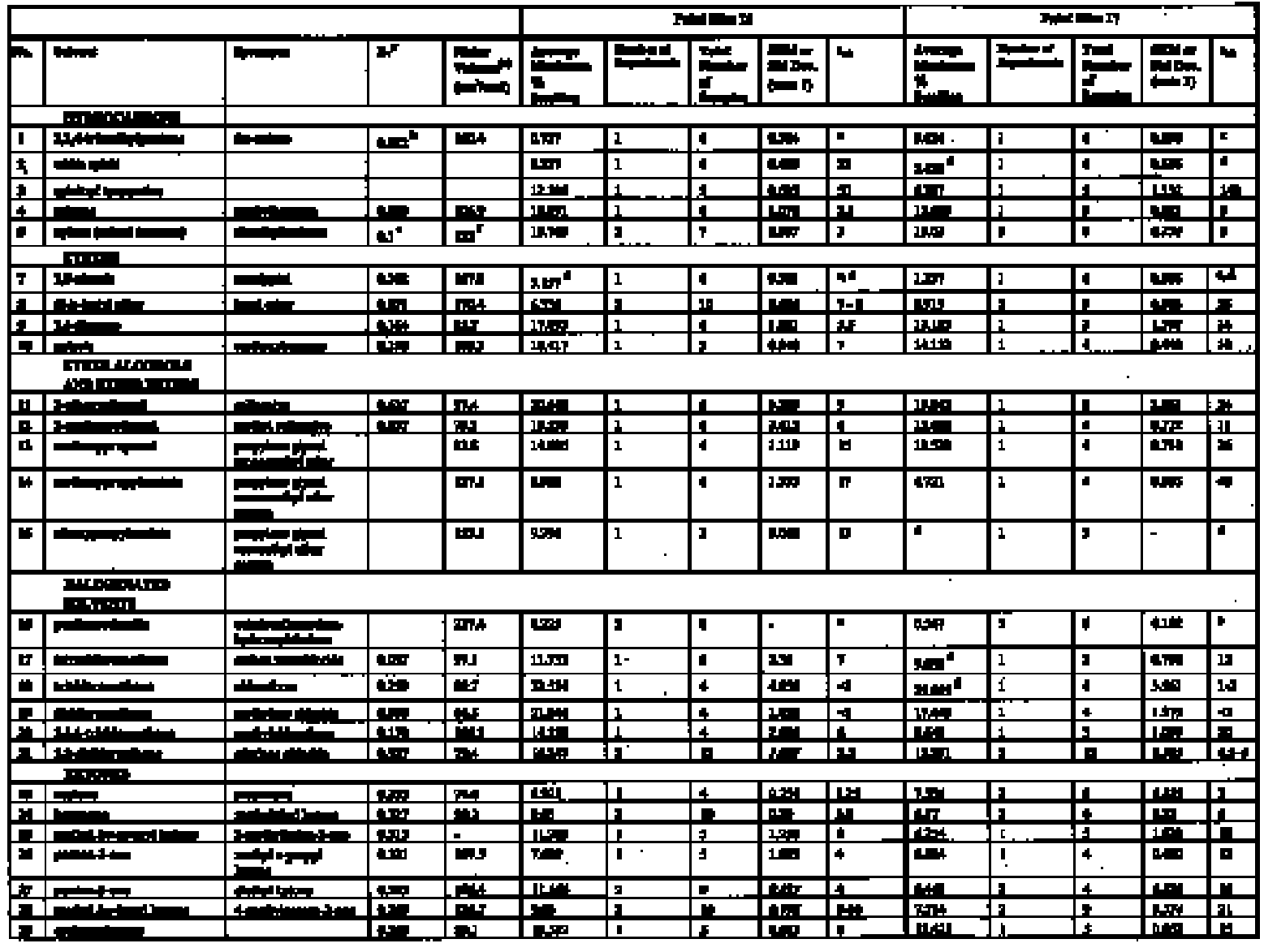 |
Table .
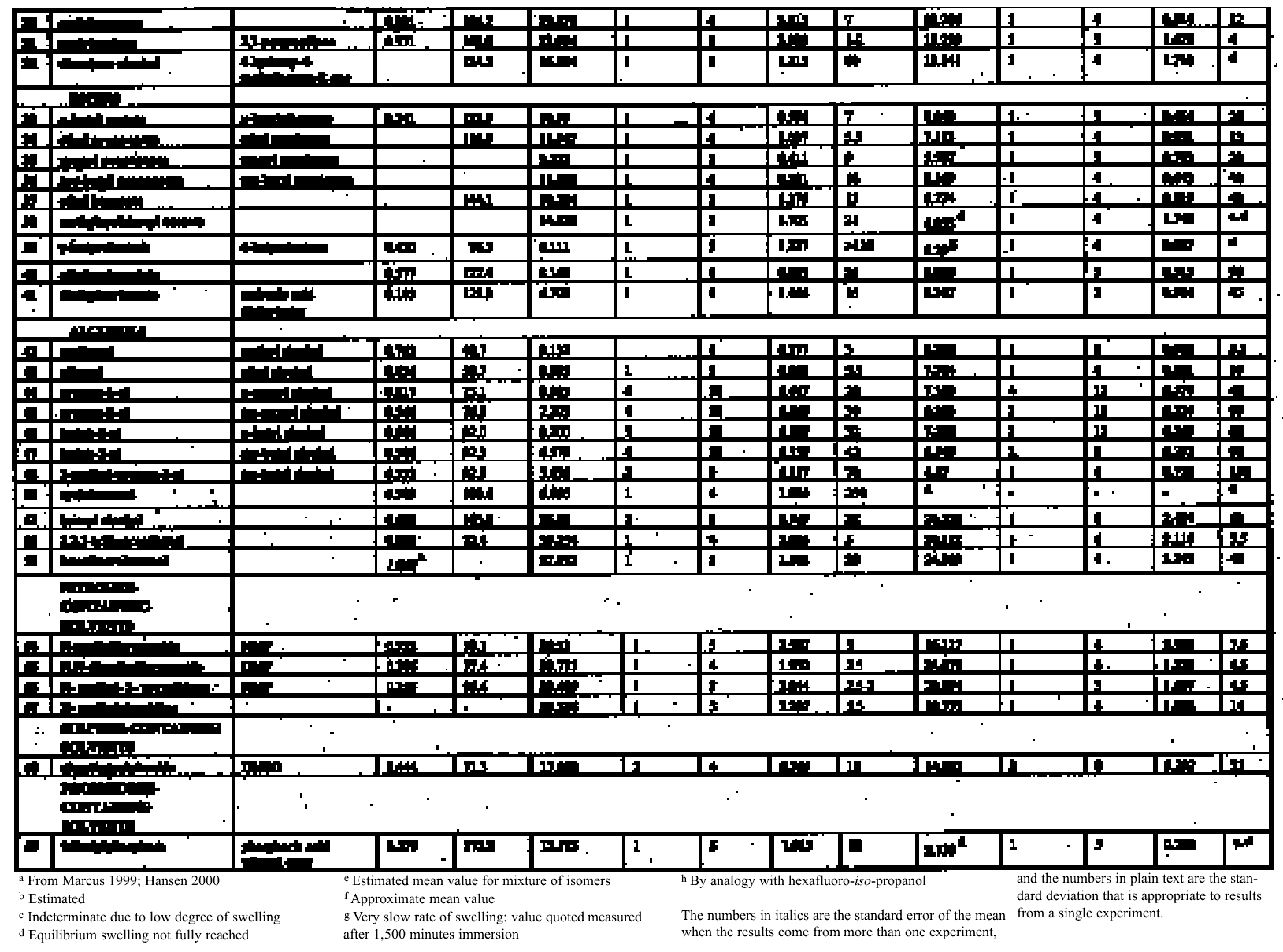 |
Fig. 6.
Representative swelling curves of paint films 16 (a) and 17 (b) in ketone solvents
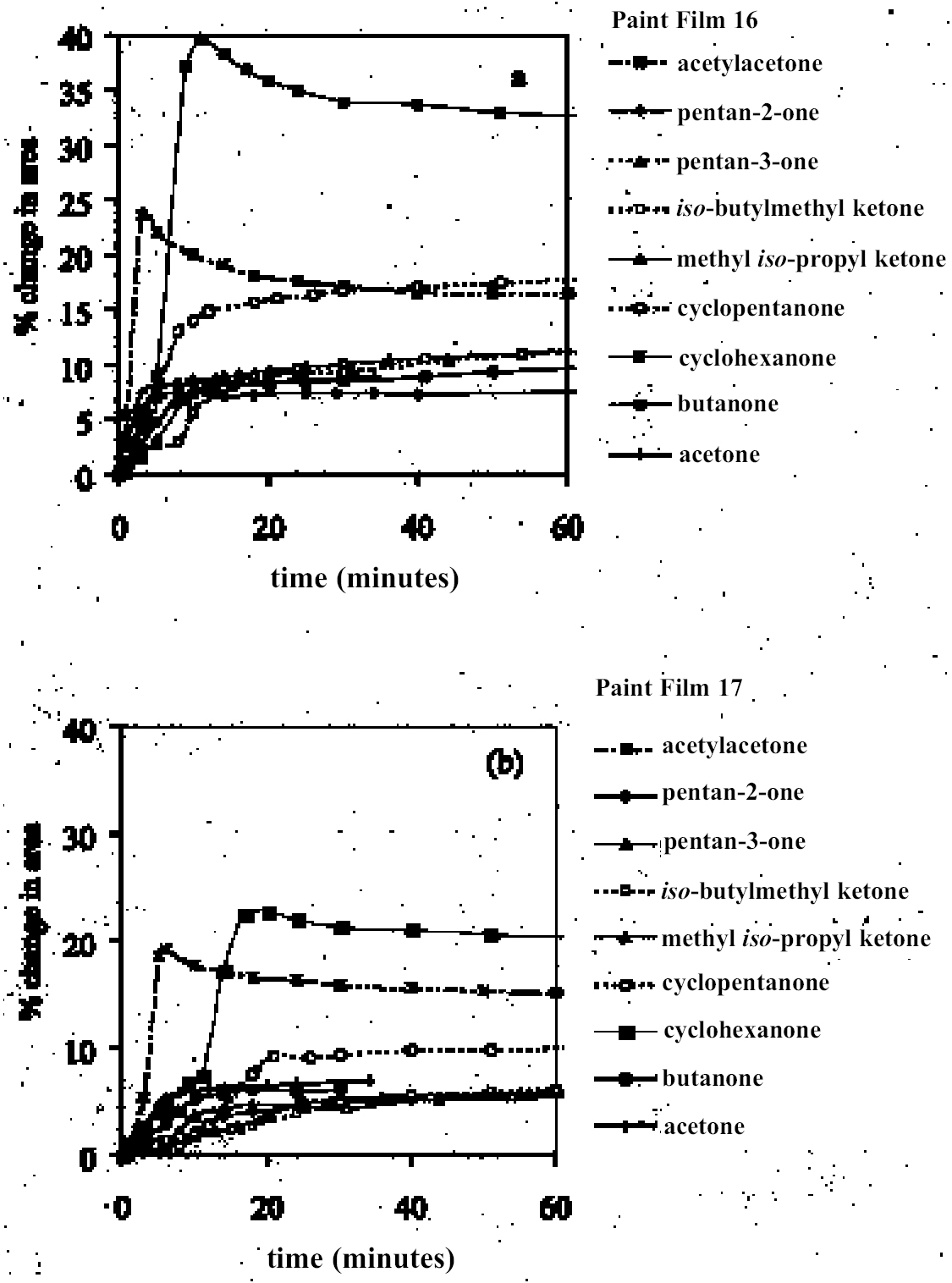 |
Fig. 7.
Representative swelling curves of paint films 16 (a) and 17 (b) in alcohols and related solvents
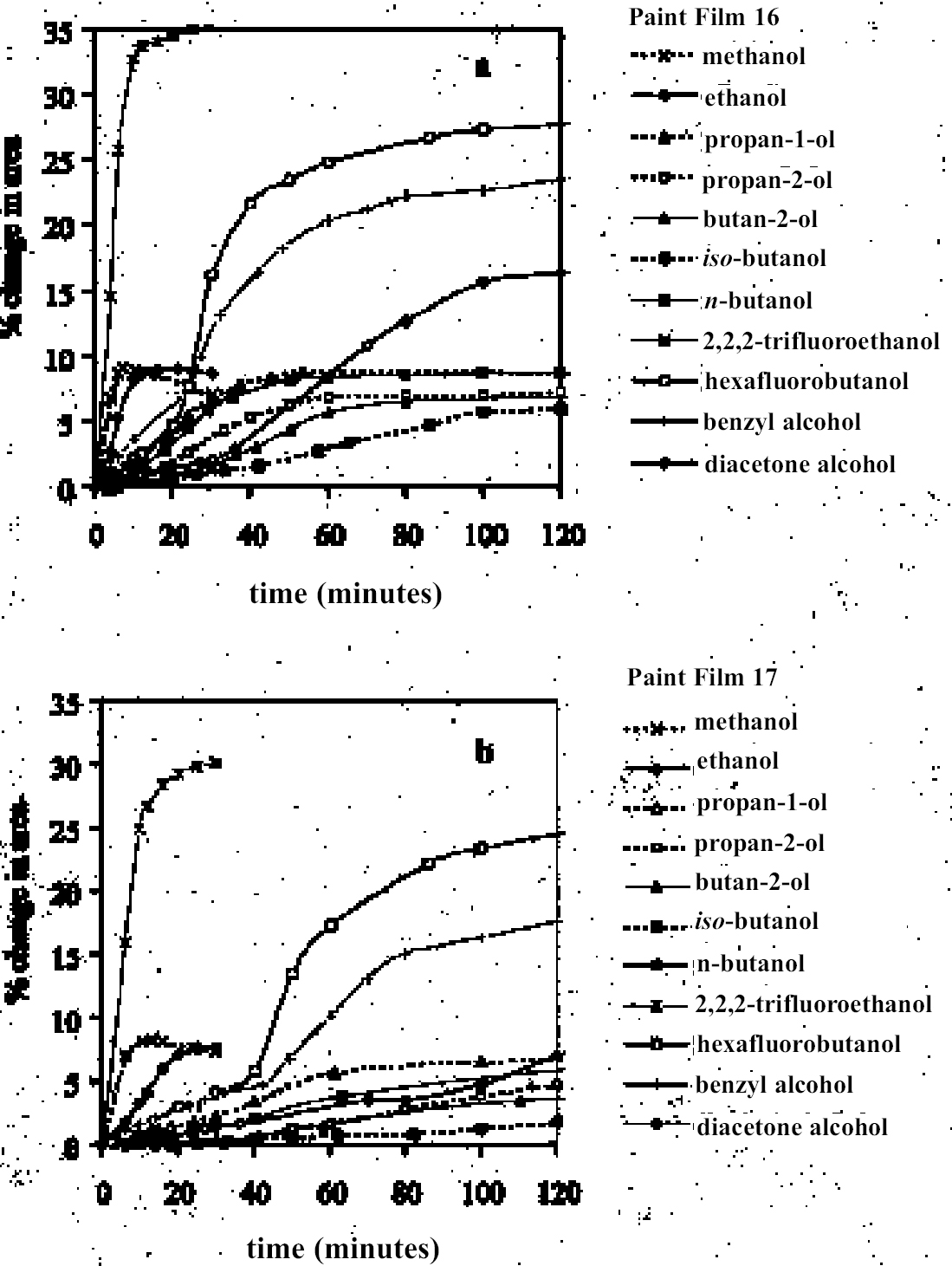 |
Fig. 8.
Representative swelling curves of paint films 16 (a) and 17 (b) in nitrogen-, sulphur-, and phosphorus-containing solvents
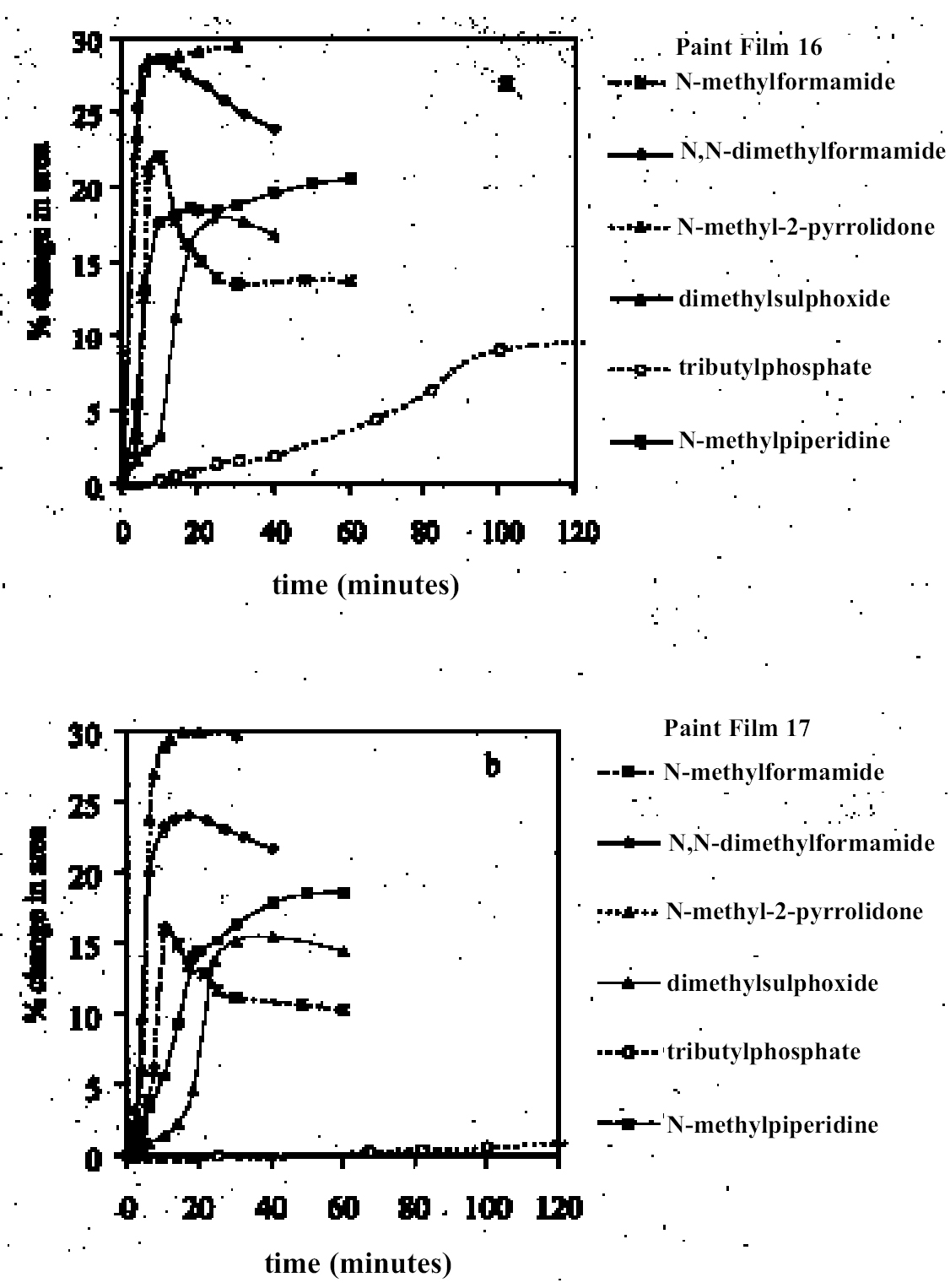 |
the aliphatic hydrocarbons, are low swellers, and a distinct peak is discernible consisting of solvents that cause especially high levels of swelling. It should be noted, however, that this peak extends generally over a much broader range of ∂ values (ca. 19–24 MPa1/2) than indicated previously, covering solvents like chloroform and cyclohexanone as identified by Stolow (1976), but now including solvents that are strongly polar in character, such as the amides and fluorinated alcohols. However, the aliphatic alcohols, plus some miscellaneous solvents, form a distinct group of polar solvents that cause, in the order of things, only low—moderate swelling in these paint films. Although, compared to other highly polar solvents the alcohols have low-swelling power on paint films 16 and 17, the lower alcohols (methanol and ethanol) are undoubtedly more active than the aliphatic hydrocarbons, a finding that differs from Stolow's (1976) findings for linseed stand oil films. The respective swelling powers of aliphatic alcohols will be discussed in more detail below. One of the difficulties with the solubility parameter ∂ as an indicator of solvency properties is that a large group of solvents from different chemical classes fall within a very narrow band of ∂ values, a fact demonstrated by the cluster of solvents in the range 17–21 MPa1/2. This cluster includes aromatic hydrocarbons, ethers, esters, ketones, and halogenated solvents. Alternative descriptors of solvent power/polarity may give better discrimination between solvents of different chemical types.
Fig. 9.
Correlation of t1/2 values for paint films 16 and 17
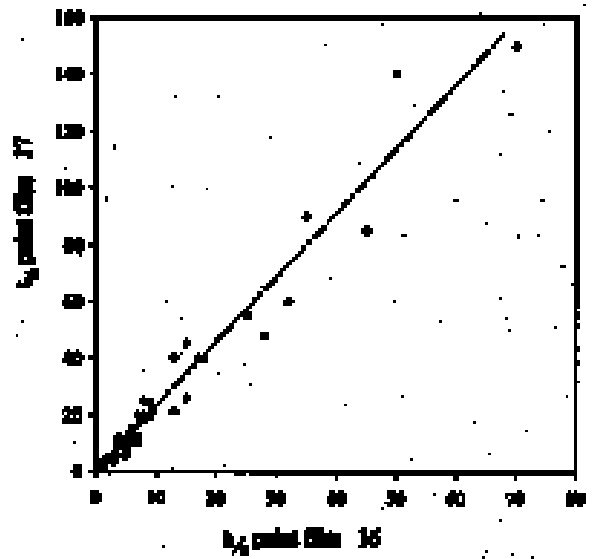 |
Fig. 10.
Maximum swelling of paint film 16 as a function of solvent solubility parameter δ (δ values mostly from Marcus 1999). Solvents falling in the ellipse are aliphatic alcohols and other polar solvents that cause relatively low degrees of swelling.
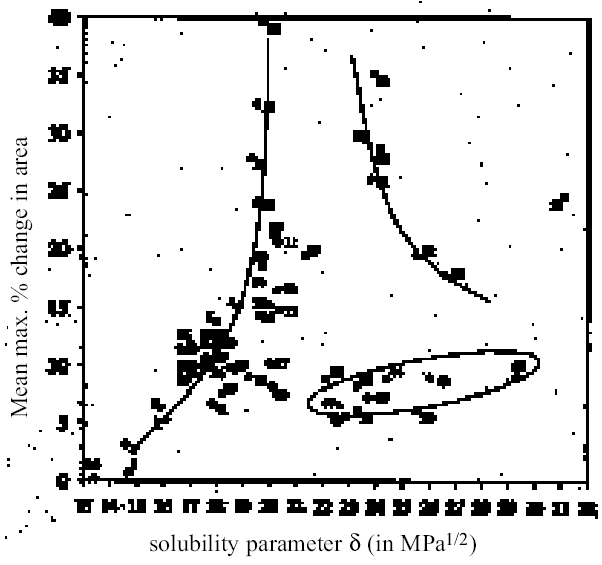 |
Figure 11a shows for paint film 16 the variation in maximum degree of swelling (as represented by % change in area) as a function of solvent polarity, here represented by the Reichardt normalized polarity value ETN (Reichardt 1990). Figure 11b shows the same data for paint film 17: the overall pattern of behavior is similar, although the magnitudes of maximal swelling are generally smaller. The various solvents can be placed into arbitrary categories according to their capacity to induce swelling and to their speed of action (see table 3). It can be seen that high degrees of swelling can be caused by solvents of various chemical classes over a broad range of polarity from ETN values of about 0.3 up to the supersolvents, with the highest possible values of around 1.0. A feature common to the lower polarity solvents from the group that causes high-moderate swelling or greater (tetrachloromethane, xylene, toluene, 1,1,1-trichloromethane, 1,4-dioxane, anisole, dichloromethane) is their high polarizability. Two classes of solvents emerge (figs. 11a, b) that have comparatively low-swelling effects for their polarity: aliphatic alcohols (indicated by trend line) and carbonyl solvents (encircled), the latter group comprising esters, selected ketones, plus miscellaneous solvents such as ethylacetoacetate (a ketoester). Again, the aliphatic alcohols can be seen to constitute a distinct group of polar solvents that cause only low-moderate swelling in the paint films under test.
Fig. 11.
Maximum swelling of paint films 16 (a) and 17 (b) as a function of Reichardt solvent polarity parameter ETN (ETN values mostly from Marcus 1999). Two groups of solvents have comparatively low swelling effects for their polarity: aliphatic alcohols (identified by trend line) and carbonyl solvents (encircled), the latter group comprising esters, selected ketones, plus miscellaneous solvents such as ethylacetoacetate.
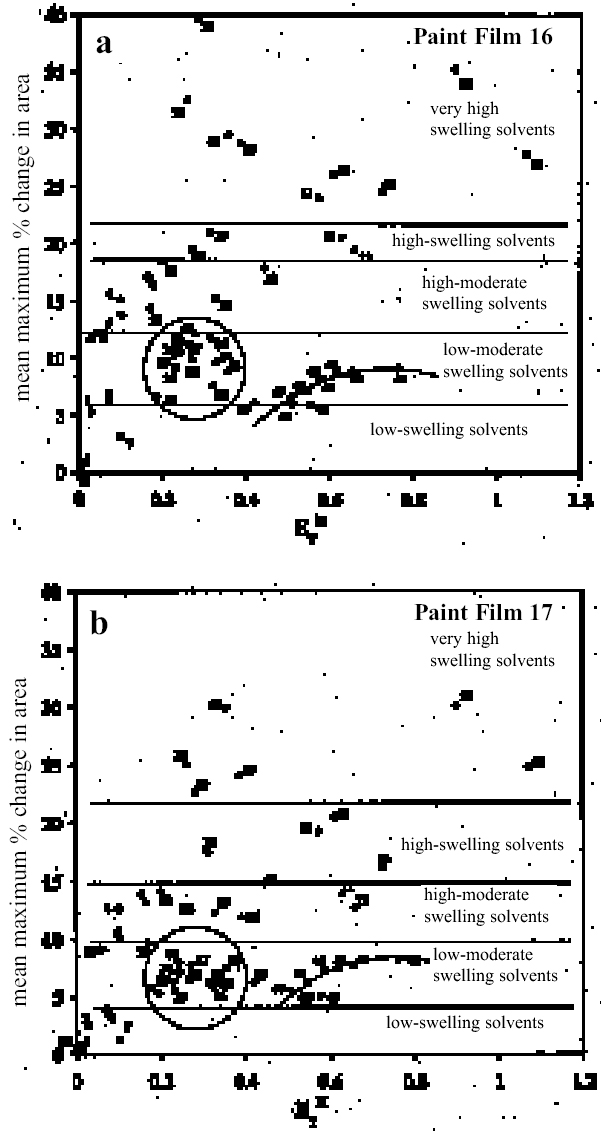 |
4.1.1 Hydrocarbons and Other Nonpolar Solvents
As might be expected from practical experience, the group of low-swelling solvents comprises mostly very nonpolar liquids, such as aliphatic hydrocarbons and related compounds (see figs. 2, 4). Perfluorodecalin, like many of its fully fluorinated relatives, is extremely nonpolar and hydrophobic. It causes barely detectable swelling in the paint films. Similar low swelling is also observed with iso-octane (2,2,4-trimethylpentane). The presence of a small proportion (<20%) of aromatics in aliphatic hydrocarbons, as in the case of white spirit, increases the swelling power of the solvent, especially on paint film 16, almost to the point of elevation to the low—moderate swelling category. For these young-mature paint films, spirit of turpentine is a low-moderate sweller. The cyclic monoterpenoid ether 1,8-cineole (eucalyptol) might also be included in the group of low-swelling, low-polarity solvents. White spirit and spirit of turpentine are rather slow in their swelling action, especially on the light-aged paint film 17: for both these solvents, equilibrium swelling is still not reached after more than 100 minutes.
The aromatic hydrocarbon solvents toluene and xylene were markedly more active than the nonaromatics, causing high-moderate levels of swelling in both paint films 16 and 17, the explanation for this response probably being their greater polarizability and molecular size and shape. For these solvents the difference in magnitude of maximal swelling between the light-aged sample and the unexposed sample is relatively small. On the other hand, the nonpolar, nonaromatic solvents white spirit and spirit of turpentine show significantly lower swelling effects on the light-exposed sample 17, a finding that may reflect a shift to greater polarity caused by aging.
Table .
Classification of Solvents in Terms of Ultimate Swelling Power and Rate of Swelling Action
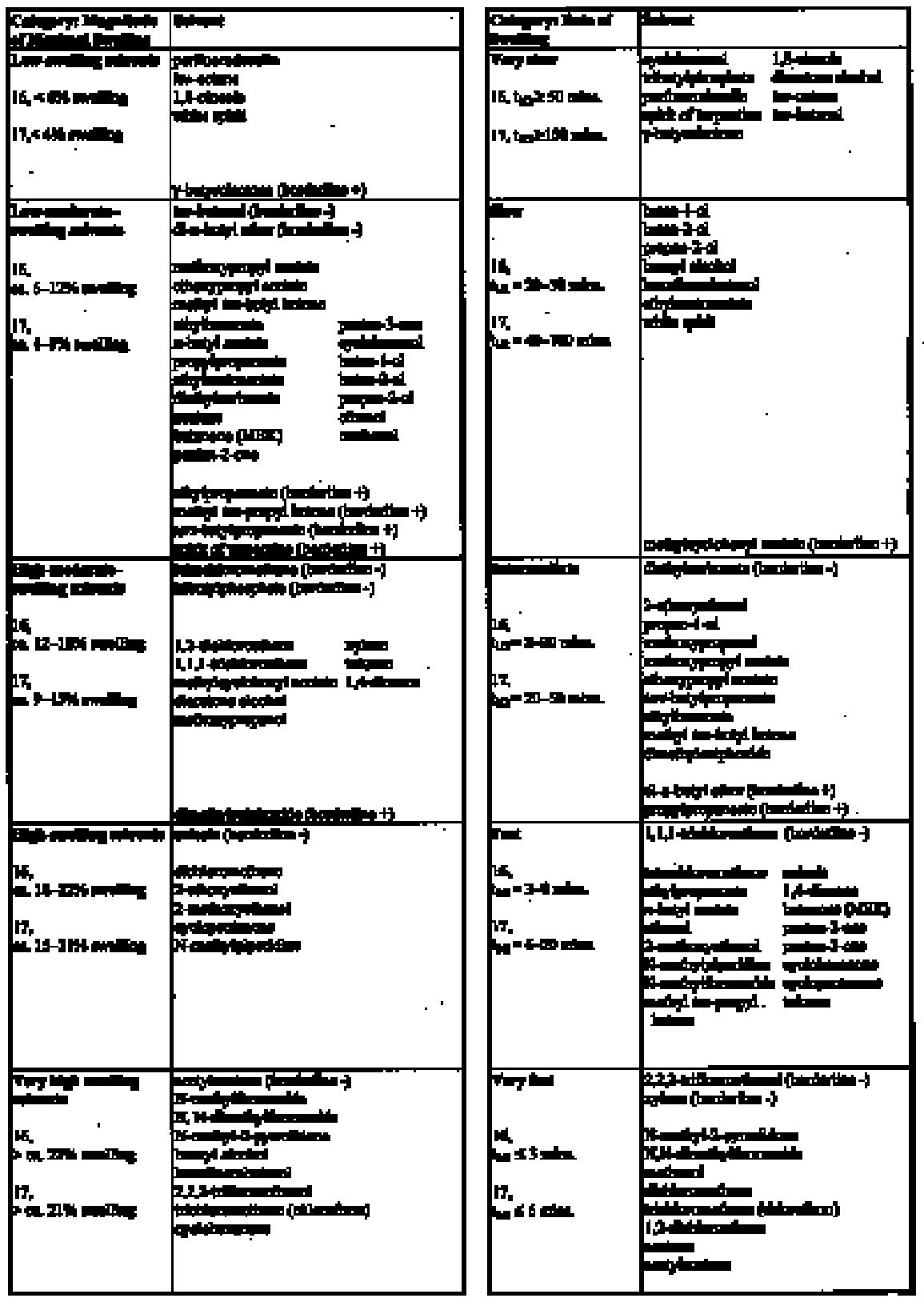 |
4.1.2 Ethers and Ether-Alcohols
The mono-functional compound di-n-butyl ether (see fig. 3) falls toward the low border of the group of low-moderate swellers, most likely as a consequence of both the low polarity of this solvent and its high molecular volume. As mentioned previously, ether solvents containing additional functional groups (aromatic ring, hydroxyl, etc.) that confer greater polarity or polarizability of the molecule cause significantly higher levels of swelling, with the consequence that 1,4-dioxane and methoxypropanol fall in the group of high-moderate swellers, with anisole, 2-ethoxyethanol (cellosolve), and 2-methoxyethanol (methyl cellosolve) among the group of high-swelling solvents. In the context of solvent substitution, it is interesting to note the low-swelling power of methoxypropanol compared to 2-ethoxyethanol, with which it shares the same ether-alcohol functionality and molecular weight. Conversion of methoxypropanol to its corresponding acetate reduces the swelling power of the solvent somewhat: methoxypropyl acetate is among the group of low-moderate swelling solvents, and the same applies to ethoxypropyl acetate (see fig. 5).
4.1.3 Halogenated Solvents
The solvent properties of simple halogenated solvents (see fig. 4) are influenced predominantly by their dipolarity due to the electron-withdrawing behavior of the halogen atom(s). Carbon tetrachloride and chloroform are well known as good solvents for fatty acids and fatty acid esters (Schmid 1973), and it is not surprising that solvents of this class are found among the most active on these young-mature paint films. For the group of chlorinated solvents examined here, swelling power is related, among other things, to polarity and molar volume. As found by Stolow (1976), chloroform (trichloromethane) is one of the most powerful solvents in its swelling action, producing very high levels of swelling, >25% for both paints 16 and 17, followed by dichloromethane. Both these solvents are aprotic dipolar compounds with small molar volumes. The reason for the particularly strong swelling power of chloroform is unclear, but it may be related to its hydrogen bonding—donor behavior in addition to its dispersion force and dipolar interactions. As far as rate of swelling is concerned, all of the halogenated solvents tested here, with the exception of perfluorodecalin, fall into the categories of “fast” or “very fast” acting liquids.
4.1.4 Esters and Related Solvents
As noted earlier, as a class, aliphatic ester solvents (see fig. 5) all generally fall in the category of low-moderate swellers, producing maximum changes of area 6–12% in paint film 16. There are a few exceptions to this general classification: ethyl propanoate, tert-butyl propanoate, and tributyl phosphate (data plotted in fig. 8), all of which are borderline high-moderate swellers for paint film 16, and methyl-cyclohexyl acetate. Increased dispersion force interactions deriving from the greater compactness of the cyclic ester may contribute to the enhanced swelling power of methylcyclohexyl acetate compared to acyclic aliphatic esters. For paint film 17, all the aliphatic esters fall comfortably within the low-moderate swelling category. Indeed, it is useful to note the similarity in swelling power of the various acyclic aliphatic esters and even ethyl benzoate. However, their rates of swelling vary considerably, essentially in relation to molecular size.
Other solvents related to the ester group— diethylcarbonate and ethylacetoacetate—also produce maximal levels of swelling in the low-moderate category; and the cyclic ester γ-butyro-lactone is on the border with the group of low-swelling solvents. These last two solvents are particularly interesting, as they appear to behave similarly to the aliphatic alcohols in that they are quite strongly polar liquids, yet they produce, in the order of things, only low swelling. γ-butyrolactone is a very slow-swelling solvent that is distinctive in that the degree of swelling it causes respectively in paints 16 and 17 is very similar.
4.1.5 Ketones and Related Solvents
Perhaps the most interesting aspect of the results for the aliphatic ketones (see fig. 6) is the diversity of swelling response that these solvents induced in the test paint films; yet this diversity also presents problems in interpretation, for patterns are hard to discern. Most obviously, the cyclic ketones appear to have enhanced swelling powers compared to their acyclic analogs. For the sample not exposed to light, 16, cyclohexanone is the most powerful of all solvents in terms of swelling action (for paint film 16, maximum change in area ca. 40%), a finding that is consistent with Stolow's results for lead white—stand oil films seven years old (Stolow 1963; Feller et al. 1985). Cyclopentanone, despite having smaller molar volume and similar polarity, does not match the very high swelling power of cyclohexanone, and it may be that specific stereochemical factors and dispersion force interactions are contributing to the potency of the latter solvent on these young-mature oil films. Cyclopentanone is still a high sweller, though, compared to analogous acyclic C5 ketones (pentan-2-one, pentan-3-one, methyl iso-propyl ketone), which are all low-moderate swellers. On the light-exposed sample 17, the swelling powers of cyclopentanone and cyclohexanone are both diminished compared to the high-swelling, high-polarity solvents such as the amides and fluorinated alcohols.
All of the acyclic ketones (acetone, butanone, pentan-2-one, etc.) produce roughly similar degrees of maximal swelling (8–12% change in area for paint film 16). The classification of acetone in terms of swelling power should be noted. This solvent is often referred to as a “strong” solvent for oil paint, and, while this label may legitimately apply to its capacity to extract soluble compounds, as far as swelling is concerned acetone is firmly in the “low-moderate” category.
As happens in a number of cases with other solvent types, the introduction of additional functional groups changes (usually by increasing) the swelling properties of ketone solvents. Accordingly, acetylacetone is on the border between the groups of high-and very high swelling solvents. Acetylacetone and acetone are among the fastest-acting solvents, with t1/2 values of less than two minutes and four minutes for paint films 16 and 17, respectively. The cyclic ketones swell at a faster rate than analogous acyclic compounds, probably by virtue of their smaller molar volumes.
4.1.6 Alcohols and Related Solvents
As mentioned previously, it can be seen from both figures 10 and 11 that the simple aliphatic alcohols, including cyclohexanol, form a distinct group of solvents characterized by high polarity and low-moderate swelling power (see fig. 7). Looking at the data for paint film 16 in more detail, it can be seen that the capacity to induce swelling increases with increasing polarity (fig. 12a) and with decreasing molecular size/molar volume. The finding that, for these plain linseed oil samples, the smaller, more polar alcohols (methanol, ethanol) are capable of producing greater degrees of swelling than the linear three-and four-carbon alcohols is at variance with the results of Stolow (1976) for linseed stand oil films. This finding
Fig. 12.
Maximum swelling of paint film 16 as a function of Reichardt solvent polarity parameter ETN (a) and solvent molar volume (b)
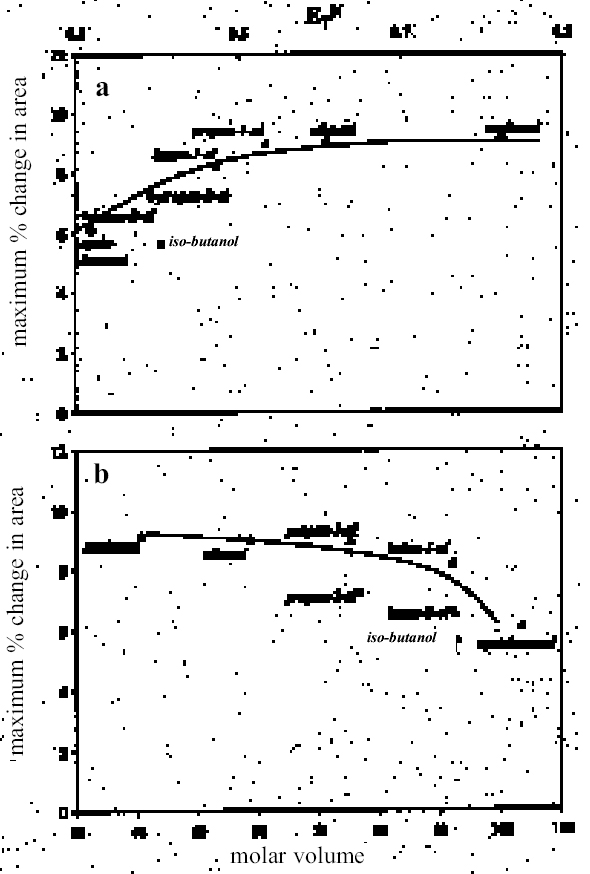 |
tends to support the view that plain linseed oil paints are more sensitive to polar solvents than previously suggested. That the smaller molecules in the homologous series cause greater degrees of swelling (fig. 12b) is consistent with conventional wisdom in the fields of paint and polymer technology. However, an observation that is consistent with the findings of Stolow (1976) is that the branched chain alcohols (propan-2-ol, butanol-2-ol, iso-butanol) all produced lower degrees of swelling than their corresponding n-isomers. Indeed, the maximum degree of swelling caused by iso-butanol on these paint films places this solvent almost in the category of low swellers.
As in the case of ketones, alcohol solvents with additional functional groups have different (again, mostly greater) swelling properties than the simple alcohols. The keto-alcohol, diacetone alcohol, is a high-moderate sweller, albeit very slow in its action. Benzyl alcohol—widely used now as an additive to aqueous cleaning formulations to enhance the activity on drying oil—containing coatings—is, not surprisingly, in the class of high-swelling solvents. The greatest degrees of swelling among the alcohols are caused by the two fluorinated alcohols, hexafluorobutanol and 2,2,2-trifluoroethanol. These are among the most polar organic compounds that are known and fall into the group of most active solvents on oil paint, comparable with chloroform, cyclo-hexanone, and dimethylformamide.
4.1.7 Nitrogen-, Sulphur-and Phosphorus-Containing Solvents
Compounds from this group (see fig. 8) are also widely recognized as some of the most powerful organic solvents that exist, and, in essence, this char-acteristic derives from their ability to engage in almost the full range of intermolecular interactions with solute molecules. The amides, for example, three of which were examined here (N-methylformamide, N, N-dimethylformamide, and N-methyl-2-pyrroli-done), are all strongly dipolar solvents, as is dimethylsulphoxide: all are characterized by high dipole moments, dielectric constant, and polarity parameters. The very high value of ∂ for N-methylformamide (NMF, solvent 54) reflects the very high specific cohesiveness of this liquid, which arises in part from its strong intermolecular association. Like water, N-methylformamide is classed in the group of hydrogen-bonded strongly associated solvents (HBSA) (Reichardt 1990).
It is not surprising, therefore, that these solvents cause high levels of swelling in the paint films under test, a finding that is consistent with empirical wisdom from conservation practice. Dimethylfor-mamide (DMF), after all, is resorted to for extreme problems such as the regeneration of paint films severely blanched by exposure to water. With paint type 16 the amides all produce roughly similar degrees of maximal swelling (25–30% change in area, “very high” category), with the cyclic compound N-methylpyrrolidone (NMP) being the most active despite having the largest molar volume. The high degrees of contraction observed in the samples during immersion in NMF and DMF possibly indicate substantial leaching of soluble material from the swollen paint. For paint film 17, the swelling power of N-methylformamide is apparently diminished in comparison to DMF and, especially, NMP. This last solvent has the most powerful effect on the light-aged paint film of all solvents tested. All three amides are fast in their swelling action, with t1/2 values for paints 16 and 17 of ≤ 5 minutes and ≤ 7.5 minutes, respectively. Unusually, the rate of swelling of NMF is slower than the larger molecule DMF.
The cyclic amine N-methylpiperidine was included in the range of test solvents essentially for comparison with the cyclic amide NMP: they have similar structures save that N-methylpiperidine has an additional carbon atom in the ring and lacks the carbonyl group. While still a member of the high-swelling group of solvents, N-methylpiperidine has somewhat lower swelling power than NMP, possibly due to diminished hydrogen-bonding interactions. Dimethylsulphoxide (DMSO), rather surprisingly, does not match the nitrogen-containing solvents for swelling power, being on the border between the high-moderate and high swelling groups. The rate of swelling in DMSO is also low by comparison with the amides, especially for the light-aged paint film 17. Tributylphosphate is one of the slowest acting of all the solvents tested, a characteristic that is almost certainly due to its large molecular size. If allowed to proceed to equilibrium swelling, it is one of the more active in the group of ester solvents and is borderline between the low-and high-moderate swelling categories.
4.2 SWELLING BY BINARY SOLVENT MIXTURES CONTAINING ETHANOL
Binary mixtures of a polar solvent, such as ethanol, and an apolar solvent, such as white spirit or iso-octane, are commonly used in varnish removal from paintings. One of the most important findings of Stolow's research was that such mixtures could induce a degree of swelling that was significantly greater than that of either of the pure solvents used alone (Stolow 1963; Feller 1976). Since binary solvent mixtures containing ethanol are such an important element of the conservator's range of cleaning tools, the swelling responses of paint films 16 and 17 were measured for a range of mixtures across the whole spectrum of polarity, from 100% white spirit to 100% ethanol and beyond. Details of the various solvent mixtures tested are given in table 4. The grade of white spirit used was Stoddard solvent (BDH Merck Ltd.). Following the approach of Feller (1976), the solvent power of the various mixtures is described by the Teas fractional dispersion parameter fd. Figure 13 shows the swelling curves for paint films 16 and 17 immersed in selected mixtures from the range tested. Selected early data points have been excluded to aid clarity of graphic presentation. It can be seen immediately that mixtures of the two solvents produce considerably higher degrees of maximum swelling than either of the pure solvents. Interestingly, for the binary mixtures of ethanol and white spirit containing 10% or more ethanol, the initial rate of swelling (up to ca. t = 8–10 minutes) is effectively the same and comparable to that for pure ethanol. This result might suggest that the rate of swelling, and probably also the rate of penetration, caused by a binary solvent mixture is governed by the diffusion of the faster component.
Figure 14 shows, for both paint films 16 and 17, the maximum values of swelling caused by binary solvent mixtures containing ethanol in relation to their Teas fractional dispersion parameters, fd. Some of the mixtures containing larger proportions of white spirit produce surprisingly high levels of swelling (>20% for paint film 16, >18% for paint film 17) putting them almost into the category of high swellers. The high sensitivity of both paints, especially 16, to solvent mixtures containing as low as 10% ethanol is perhaps an indication of their relatively youthful nature. The greatest degrees of swelling are caused by solvent mixtures in the range fd 77–85: this peak may have some correspondence with the single peak observed by Stolow for lead white—stand oil films (Stolow 1963, fig. 4) However, there remains a broad plateau representing mixtures of higher polarity that cause swelling to levels comparable with the high-moderate category described previously. Pure ethanol and a 10% water-ethanol mixture, by comparison, are low-moderate swellers, and pure white spirit is a low sweller. This pattern suggests that, while young-mature oil paint films may show a particular sensitivity to a narrow window of relatively apolar solvent mixtures, they are also capable of being swollen to a significant degree by solvent mixtures covering a much broader polarity range. As observed with virtually all pure solvents, the light-aged paint film 17 swells to a lower degree than its unexposed analog, but the general pattern of behavior is similar, although for paint 17 there are signs of reduced sensitivity to very apolar solvents, white spirit and white spirit: ethanol 19:1.
It was noted by Michalski (1990) that mixtures of ethanol and castor oil might provide a useful means of skirting round the peak swelling region of oil paint, by virtue of the distinctive solubility parameters (fd: 56, fp: 11, fh: 33) of the oil. Only one such mixture has been tested to date, castor oil: ethanol 1:1, and while this mixture appears to produce slightly lower maximal degrees of swelling than ethanol—white spirit mixtures of comparable polarity, the difference is only slight. It remains to explore the swelling power of mixtures of ethanol and castor oil in the more significant region of lower polarity.
Fig. 13.
Representative swelling curves of paint films 16 (a) and 17 (b) in binary solvent mixtures containing ethanol
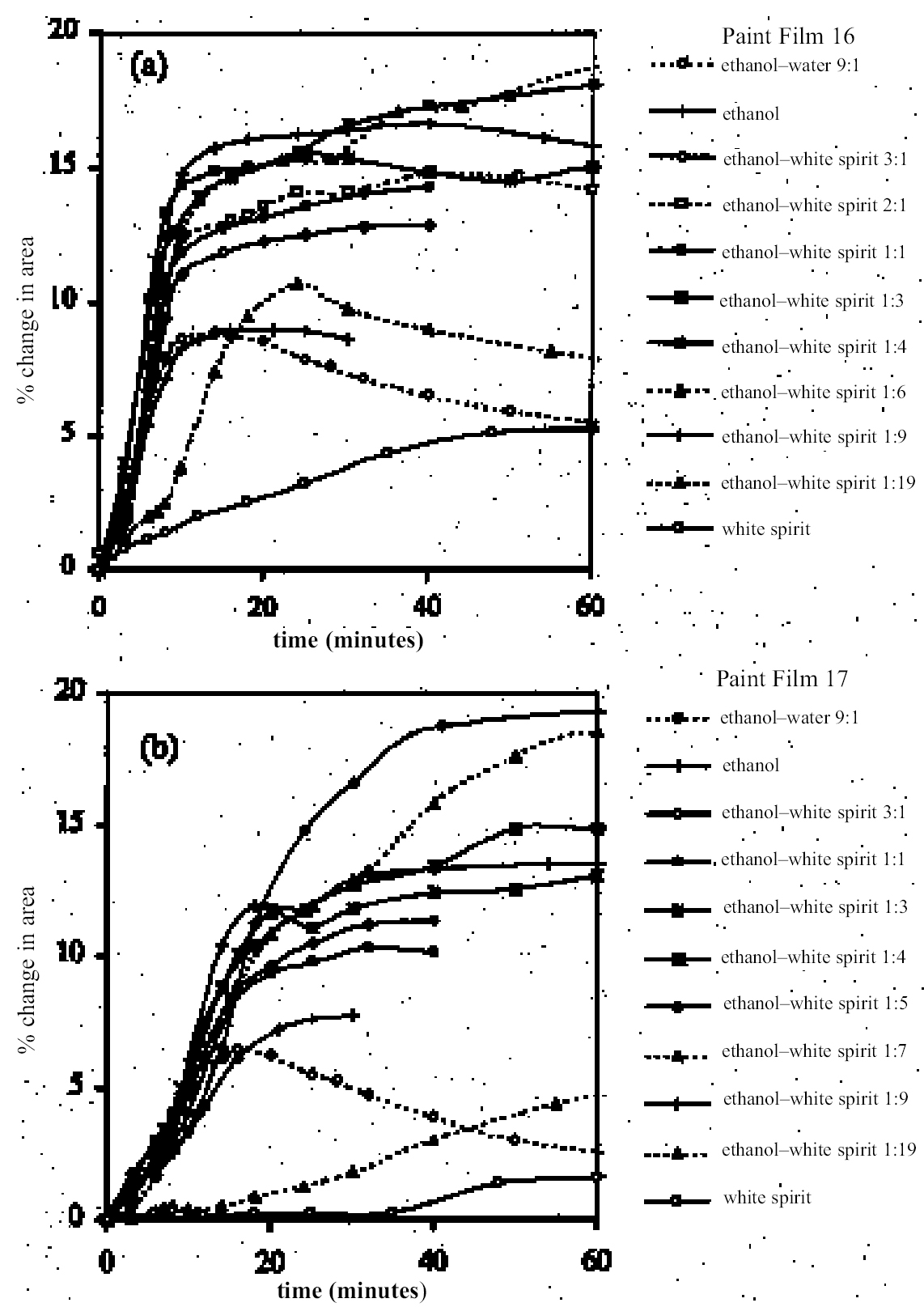 |
Table .
Compilation of Data for Swelling of Paint Films 16 and 17 in Binary Solvent Mixtures Containing Ethanol
 |
5 SUMMARY
It has been shown that the low-power microscopy—image analysis technique can be used successfully for measuring the comparative swelling powers of organic liquids on young-mature oil paint films such as the ones tested here. The results obtained in this study indicate a more complex relationship between swelling power and individual solvent characteristics, especially polarity, than is reflected by previous studies. Several types of polar solvent are capable of generating high degree of swelling in the paints tested. Although the lower alcohols produced relatively low degrees of swelling in comparison with other solvents having high values of Reichardt polarity ETN and accordingly were classified as low-moderate swellers, methanol and ethanol in particular have been shown to be appreciably more active on these test paints than on the lead
Fig. 14.
Maximum swelling of paint films 16 and 17 as a function of Teas fractional dispersion force solubility parameter, fd
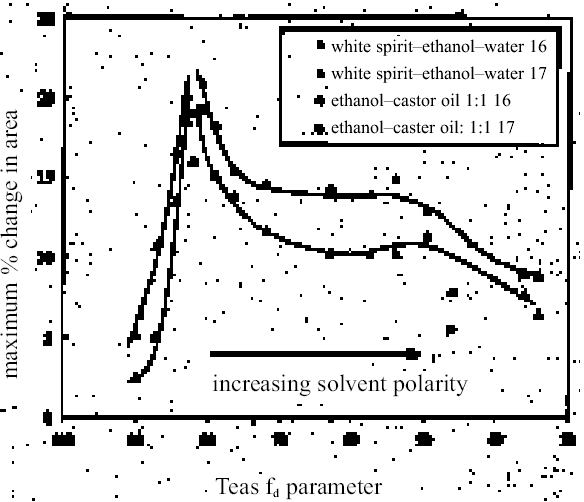 |
white—stand oil films of Stolow (1955). Indeed, there is some correspondence in the present data with Stolow's results on linseed oil films other than stand oil. This correspondence is indicated, for example, by the broad shoulder toward higher polarity in figure 14 that also appears in figure 1.
In the vast majority of cases, the light-aged paint film 17 shows distinctly lower degrees and slower rates of swelling than its unexposed equivalent. It is conceivable that more severe aging would lead to progressive decline in these properties. The markedly lower sensitivity of paint film 17 to some apolar solvents, such as white spirit and di-n-butyl ether, may point to contraction of the swelling region on the apolar side caused by light exposure. Paint film 17 remains similarly sensitive to solvents at the more polar end of the range as its unexposed equivalent, 16.
It can be seen that there is not a very clear relationship between the swelling power of a given solvent and a single descriptor of solvent power such as Reichardt ETN or total solubility parameter, ∂. The plots in figures 10 and 11 show, at best, rather loose patterns of behavior, and this finding applies also with most other polarity indicators. It seems likely that attempting to correlate swelling power against any single indicator of solvent power will lead to similarly unresolved outcomes, as many factors will influence the manner of paint solvent interaction. As well as polarity, other factors that will be significant are molecular size, polarizability, hydrogen-bonding donor and acceptor properties, electron pair donor/acceptor behavior, and acidity/basicity. An approach to data analysis that addresses the potential contribution of many factors, such as multivariate analysis, may ultimately be more fruitful.
The finding of swelling powers almost reaching the “high” category for some of the ethanol—white spirit mixtures in the range of Teas fd 80–85 has significant implications for cleaning practice, particularly for relatively young paintings. On the young-mature paint films tested here, the swelling power of white spirit mixtures increases quite significantly as the proportion of ethanol is increased above about 8–10%. The sharp increase in paint sensitivity in this relatively low polarity territory may give the conservator using the conventional practice of progressive addition of ethanol to white spirit to increase polarity very little room to maneuver in finding a solvent mixture that will effectively remove a varnish yet still be safe for the underlying original paint. It may be that, in such cases of removing a varnish from a relatively apolar, young-mature paint film, working from the other end of the polarity range may be a more effective strategy. Passages in many paintings from the later part of the 19th century and the first half of the 20th century may be of this type.
In terms of practical application of the results presented here, the main thing has been to clarify the activity of the lower alcohols and of other solvents commonly used in conservation, yet previously untested. Thus the powerful action of strongly dipolar solvents such as N, N-dimethylformamide and N-methyl-2-pyrrolidone—used variously to help remove oil-containing varnishes and overpaints— have been confirmed. Similarly, other solvents occasionally resorted to by conservators to remove tough coatings containing oil (e.g., cellosolve, benzyl alcohol, diacetone alcohol, and dichloromethane) have also been shown to be within the “high-moderate,” “high,” and “very high” swelling categories. Although, as mentioned previously, the lower aliphatic alcohols have been found to be stronger swellers for oil paints than perhaps reflected by Stolow's (1976) stand oil data, the simple alcohols (and acetone) are mostly reassuringly low-moderate swellers. Considering also their polarity and their health and safety properties, the value of the alcohols as favored tools in the solvent-cleaning of paintings remains uncompromised by the present findings. Other members of the group of low-swelling, high-polarity solvents have been identified, however, as perhaps warranting further investigation: ethylacetoac-etate and γ-butyrolactone.
It is envisaged that the data presented here, in addition to helping the practical conservator anticipate and control risk in varnish removal, may also serve to guide formulation of agents for the active removal of paint, as in the cases of removing overpaint from paintings or from, say, historic decorated interiors.
6 CONCLUSIONS
This study represents a preliminary exploration of the potential of the microscopy—image analysis method for measuring the relative activity of solvents in affecting the physical properties of paint in the context of cleaning works of art. It is the first study of the swelling of artist's oil paints since Stolow's pioneering work of the 1950s. Some ambiguities that have continued since then have been clarified, but there are many questions remaining. Preleached paint films will undoubtedly behave differently from virgin films. Some formalized schemes for selection of solvents and solvent mixtures for removing coatings of various kinds are yet to be addressed specifically. It is known also that paint films swell in water and that swelling is influenced by pH. Examination of this latter phenomenon should have direct relevance to some of the new water-based cleaning approaches that have been introduced in the last 10–15 years (Wolbers 2000). The intention is that that these issues will be pursued in further studies.
ACKNOWLEDGEMENTS
This work was commenced while I was on secondment from the Courtauld Institute of Art to the MOLART (Molecular Aspects of Aging in Painted Works of Art) project, a Prioriteit research program funded by the Dutch Organization for Scientific Research (NWO) and organized by the Foundation for Fundamental Research on Matter, Institute for Atomic and Molecular Physics (FOM-AMOLF), Amsterdam. I gratefully acknowledge the support of the Courtauld Institute of Art for facilitating this period of secondment. The work could not have been undertaken with-out the equipment acquired with the aid of a grant from the Research Committee of the Courtauld Institute of Art. Special thanks must also go to Tom Bilson of the Courtauld Institute of Art for assistance with the digital imaging and analysis and to Ken Sutherland for his gas chromatography—mass spectroscopy work on the samples that form the subject of this article. I am indebted to Professor Steen Sauerberg, University of Copenhagen, without whom this work would not have seen the light of day.
NOTES
1. The measurements from separate experiments can be considered as a sample from an infinite population of possible measurements; and, likewise, the means of any series of measurements are a sample from the possible means of samples of measurements from the whole population. The distribution of the sample means is called the sampling distribution of the mean: its mean is the same as the mean of the original population. The standard error of the mean (SEM) is equivalent to the standard deviation of the sampling distribution of the mean (Miller and Miller 1993). The standard error of the mean can also be determined from the relationship between the standard deviation of the individual measurements, ς, and the sample size, n, following the equation: SEM = ς / √n, and this method has been used here.
REFERENCES
Banik, G., and G.Krist. 1984. L�sungsmittel in der Restaurierung. Vienna: ICCROM and Verlag der Apfel.
Blank, S., and C.Stavroudis. 1989. Solvents and sensibility. Western Association for Art Conservation Newsletter11(2):2–10.
Browne, F. L.1956. Swelling of oil paints in water. Part 8, Swelling of linseed oil paints in water and organic liquids.Forest Products Journal6:312–18.
Brunt, N. A.1962. The influence of swelling by water vapor on the mechanical properties of a polymer. Kolloid-Zeitschrift und Zeitschrift f�r Polymere185(2):119–25.
Brunt, N. A.1964. Blistering of paint layers as an effect of swelling by water.Journal of the Oil and Colour Chemists' Association47:31–42.
Cremonesi, P.2000. L'uso dei solventi organici nella pulitura di opere policrome. Padua: Il Prato.
Eissler, R. L., and L. H.Princen. 1968. Effect of some pigments on tensile and swelling properties of linseed oil films. Journal of Paint Technology40(518):105–11.
Eissler, R. L., and L. H.Princen. 1970. Swelling of linseed oil films in acid and alkaline environments. Journal of Paint Technology42(542):155–58.
Elm, A. C.1949. Deterioration of dried oil films.Industrial and Engineering Chemistry41:319–24.
Erhardt, D., and J. S.Tsang. 1990. The extractable components of oil paint film. In Cleaning, retouching, coatings,ed. J. S.Mills and P.Smith. London: International Institute for Conservation. 93–97.
Erhardt, D., C. S.Tumosa, and M. F.Mecklenburg. 2000. Natural and accelerated thermal aging of oil paint films. In Tradition and innovation,ed. A.Roy and P.Smith. London: International Institute for Conservation. 65–69.
Feller, R. L.1976. The relative solvent power needed to remove various aged solvent-type coatings. In Conservation and restoration of pictorial art,ed. N.Bromelle and P.Smith. London: International Institute for Conservation and Butterworths. 158–62.
Feller, R. L., N.Stolow, and E. H.Jones. 1971. On picture varnishes and their solvents. Rev. ed. Cleveland: Case Western Reserve University.
Feller, R. L., N.Stolow, and E. H.Jones. 1985. On picture varnishes and their solvents. Rev. and enlarged ed. 1985. Washington D. C.: National Gallery of Art.
Frilette, V. J.1946. Drying oil and oleoresinous varnish films: Increase in acidity on aging. Industrial and Engineering Chemistry38(5):493–96.
Hansen, C. M.2000. Hansen solubility parameters: A user's handbook. Boca Raton, Fla.: Chemical Rubber Company.
Hedley, G.1980. Solubility parameters and varnish removal: A survey. Conservator4:12–18. (Reprinted, with corrections, in Measured opinions [1993], ed. C.Villers. London: United Kingdom Institute for Conservation. 128–34.)
Kamlet, M. J., J.-L. M.Abboud, and R. W.Taft. 1983. Linear solvation energy relationships. Part 23, A comprehensive collection of solvatochromic parameters π*, α, β, and some methods for simplifying the general solvatochromatographic equation.Journal of Organic Chemistry48:2877–87.
Laurence, C., P.Nicolet, M.Tawfik Dalati, J.-L. M.Abboud, and R.Notario. 1994. The empirical treatment of solvent-solute interactions: 15 years of π*.Journal of Physical Chemistry98:5807–16.
Marcus, Y.1999. The Properties of solvents. Chichester, U. K.: John Wiley.
Michalski, S.1990. A physical model of the cleaning of oil paint. In Cleaning, retouching, coatings, ed. J. S.Mills and P.Smith. London: International Institute for Conservation. 85–92.
Miller, J. C., and J. N.Miller. 1993. Statistics for analytical chemistry3d ed.London: Ellis Horwood.
Mills, J. S., and R.White. 1999. The organic chemistry of museum objects. 2d ed.Oxford: Butterworth-Heinemann.
Phenix, A.1998a. The science and technology of the cleaning of pictures: Past, present and future. In Proceedings of 25th anniversary conference, School of Conservation, ed. K.Borchersen. Copenhagen: Royal Danish Academy of Art. 109–19.
Phenix, A.1998b. Solubility parameters and the cleaning of paintings: An update and review. Zeitschrift f�r Kunsttechnologie und Konservierung12(2):387–409.
Phenix, A2002. The swelling of artists'paints in organic solvents. Part 1, A simple method for measuring the inplane swelling of unsupported paint films. Journal of the American Institute for Conservation41:43–60.
Reichardt, C.1990. Solvents and solvent effects in organic chemistry. 2d ed.Cambridge: Wiley-VCH.
Ruhemann, H.1968. The cleaning of paintings. London: Faber and Faber.
Schilling, M. R., D. M.Carson, and H. R.Khanjian. 1999. Gas chromatographic determination of the fatty acid and glycerol content of lipids. Part 4, Evaporation of fatty acids and the formation of ghost images by framed oil paintings. In ICOM Committee for Conservation preprints, ed. J.Bridgland, 12th Triennial Meeting, Lyons, 1999. London: James and James. 1:248–53.
Schilling, M. R., H. R.Khanjian, and D. M.Carson. 1997. Fatty acid and glycerol content of lipids: Effects of aging and solvent extraction on the composition of paints.Techne5:71–78.
Schmid, P.1973. Extraction and purification of lipids. Part 2, Why is chloroform-methanol such a good lipid solvent?Physiological Chemistry and Physics5:141–50.
Stolow, N.1955. Some investigations of the action of solvents on drying oil films. Ph. D. diss., Courtauld Institute of Art, University of London.
Stolow, N.1957. The action of solvents on drying oil films. Part 1.Journal of the Oil and Colour Chemists' Association40:377–402.
Stolow, N.1963. Application of science to cleaning methods: Solvent action studies on pigmented and unpigmented linseed oil films. In Recent advances in conservation, ed. G.Thomson. London: Butterworths. 84–88.
Stolow, N1965. The application of gas chromatography in the investigation of works of art. In Application of science in the examination of works of art. Seminar held at the Museum of Fine Arts. Boston: Museum of Fine Arts. 172–83.
Stolow, N.1976. Solvent action. In Conservation and restoration of pictorial art, ed. N.Bromelle and P.Smith. London: International Institute for Conservation and Butterworths. 153–57.
Stoner, J. H.1994. The impact of research on the lining and cleaning of easel paintings.Journal of the American Institute for Conservation33:131–40.
Sutherland, K.2000. Personal communication. Scientific Research Division, National Gallery of Art, Washington, D.C.
Sutherland, K.2001. Solvent extractable components of oil paint films. Ph. D. diss., University of Amsterdam.
Sutherland, K., and N.Shibayama. 1999. The components of oil paint films extracted by organic solvents. In ICOM Committee for Conservation preprints, ed. J.Bridgland, 12th Triennial Meeting, Lyons, 1999. London: James and James. 1:341–46.
Tumosa, C. S., J.Millard, D.Erhardt, and M.Mecklenburg. 1999. Effects of solvents on the physical properties of paint films. In ICOM Committee for Conservation preprints,ed. J.Bridgland, 12th Triennial Meeting, Lyons, 1999. London: James and James. 1:347–52.
van den Berg, J. D. J., K. J.van den Berg, and J. J.Boon. 1999. Chemical changes in curing and ageing oil paints. In ICOM Committee for Conservation preprints, ed. J.Bridgland, 12th Triennial Meeting, Lyons, 1999. London: James and James. 1:248–53.
White, R., and A.Roy. 1998. GC-MS and SEM studies on the effects of solvent cleaning on old master paintings from the National Gallery, London.Studies in Conservation43:159–76.
Wolbers, R.2000. Cleaning painted surfaces: Aqueous methods. London: Archetype.
AUTHOR INFORMATION
ALAN PHENIX was educated at Leeds University, United Kingdom, receiving a B.Sc. Honors in chemistry and color chemistry combined in 1979. He studied conservation of easel paintings for three years at the Courtauld Institute of Art, University of London, and was awarded the institute's postgraduate diploma in 1984. After an internship at Tate Gallery, London, he worked as a paintings conservator in Australia, returning to Britain in 1989 to take up a teaching and research position at the Hamilton Kerr Institute, University of Cambridge. He was a lecturer in the Department of Conservation and Technology at the Courtauld Institute of Art from 1991 to 2000, during which time he spent 15 months on secondment to the MOLART (Molecular Aspects of Aging in Painted Works of Art) project managed by the Foundation for Fundamental Research on Matter, Institute for Atomic and Molecular Physics (FOM-AMOLF), Amsterdam. During 2001 he was research fellow at the Royal College of Art, London, and he has recently been appointed associate professor at the Institute for Archaeology, Art History and Conservation at the University of Oslo. He is presently coordinator of the ICOM-CC Working Group Paintings 1: Conservation and Restoration of Paintings. Address: Department of Conservation Studies, Institute for Archaeology, Art History and Conservation (IAKK), University of Oslo, Frederiks gt. 3, 0164 Oslo, Norway.
Section Index |