OBSERVATIONS ON CYCLODODECANE AS A TEMPORARY CONSOLIDANT FOR STONE
REN�E STEIN, JOCELYN KIMMEL, MICHELE MARINCOLA, & FRIEDERIKE KLEMM
5 5. EVALUATION OF PURITY AND EXTENT OF SUBLIMATION VIA FTIR AND GC-MS
Due to its ability to sublime at room temperature, cyclododecane will gradually depart from a substrate, and it is not reported to contain any additives that might remain in or on the substrate after sublimation. Purity of the material was examined by analyzing samples from a single can of the consolidant using both Fourier transform infrared spectrometry (FTIR) and gas chromatography-mass spectrometry (GC-MS). As noted above, no residual traces of cyclododecane or of any impurities or additives were visible on the treated glass slides after sublimation. Nor were any residues indicated by the weights of treated stone samples after sublimation of the consolidant. GC-MS was employed as a sensitive technique for the qualitative detection of residues that would be too small to be detected visually or by weight. GC-MS provides highly sensitive yet indirect analysis that enables detection of components on the order of parts per billion. More direct examination of the samples themselves for possible evidence of residues of any sort could be accomplished with cryo-SEM, as utilized by Riedl and Hilbert (1998), or by diffuse reflectance micro-FTIR. The former technique was not available for the present study, and the latter is not as sensitive as GC-MS.
A sample of cyclododecane was pressed between a pair of diamond cells and scanned 50 times at a resolution of 4 wavenumbers, using a Bio-Rad FTS 40 spectrometer with UMA 500 microscope. The infrared spectrum of cyclododecane (fig. 5) illustrates a hydrocarbon without carbonyl functionality. Straight-chain paraffins, which might be expected as impurities or additives in cyclododecane, are not reflected in the infrared spectrum. Peaks characteristic of paraffins, around 1150–1130 cm−1 and 1090–1055 cm−1, are absent from the spectrum of cyclododecane.
Fig. 5.
Infrared spectrum of cyclododecane
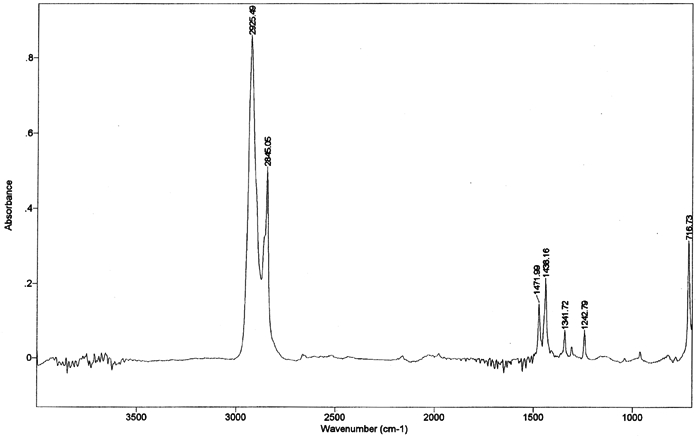 |
Cyclododecane was then dissolved in ether, and a sample volume of 2 μl was analyzed on a Hewlett Packard GC-MS system, which consists of a 5980 capillary gas chromatograph with split/splitless injection port and 5989B mass selective detector. A Supelco SPB-1 bonded dimethylsiloxane column (30 m, 0.25 mm diameter, 0.25 μm film thickness) was used with helium as the carrier gas at a flow rate of approximately 1 ml/min and a linear velocity of 39.0 cm/sec. The injection port temperature was 250�C, and injections were carried out in split mode with a split ratio of approximately 1:10. The temperature was held for one minute at 45�C, then ramped at 5�/minute to 70�C and then at 30�/minute to 280�C, and held at the final temperature for 27 minutes to give a total run of 40 minutes. MS analysis was carried out in scan mode with a transfer line temperature of 200�C and temperature of the quadrapole ion source at 100�C. This analysis of cyclododecane via GC-MS further demonstrates the material's purity with a single elution of the consolidant at approximately 11 minutes (fig. 6).
Fig. 6.
Analysis of cyclododecane purity via gas chromatography-mass spectrometry
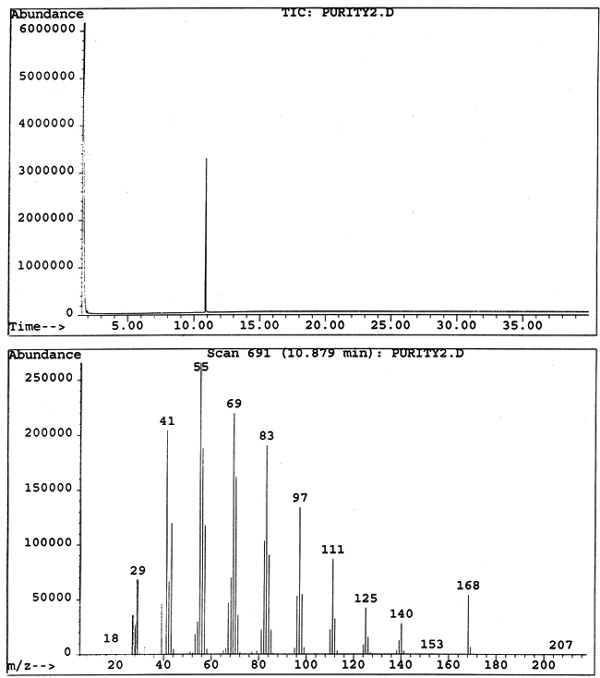 |
Testing for residues in selected stone types was then carried out. The limestone and sandstone cores were treated with melted cyclododecane and with saturated consolidant solutions as described in the preceding section. The samples were weighed before treatment and periodically during sublimation. Once all samples had returned to their untreated weights, approximately four weeks after treatment, consolidant loss was presumed complete. Because GC-MS analysis was in part intended to confirm complete sublimation as indicated by weight, it was necessary to prevent any further sublimation after the four-week weighing period. Several days were required to prepare and analyze each of the 30 samples; therefore, after four weeks the samples were placed in two layers of zip-close polyethylene bags, significantly inhibiting further sublimation as demonstrated in section 2 above. Prior to analysis, each stone sample was crushed with a metal mortar and pestle and transferred to a glass jar with a plastic lid. Then 4 ml of reagent-grade hexanes were added to each jar. The closed jars were gently agitated, and the stone dust was allowed to settle. Because cyclododecane is an unbranched cyclic molecule and unlikely to bond strongly with the substrate, traces of cyclododecane should have been easily extracted from the crushed samples by flushing with hexanes. Five drops of the hexanes solution were transferred with a disposable plastic pipette into 2 ml glass vials with plastic screw-tops. Approximately 1 ml of high-performance liquid chromatography-grade ether was added to each vial, and the vials were gently agitated.
Then 1 μl of the ether solution was injected onto the column for GC-MS analysis. Instrumental parameters were as described above, with the exception of the temperature program, which was modified to shorten the time required for each run of analysis. The temperature program for analysis of samples from the treated stones was as follows: the starting temperature of 35�C was held for 1.5 minutes, then ramped at 5�/minute to 70�C and then 30�/minute to 250�C, and held at the final temperature for 1 minute to give a total run of 15 minutes. The sensitivity for this analytical protocol was evaluated on the basis of increasingly dilute solutions of a known concentration of cyclododecane in hexanes and ether. A 1 μl sample of the solution standard containing 1 � 10−8 grams of consolidant yielded an ion abundance of less than 50; thus, it is evident that cyclododecane can be detected well below 1 ppb.
Those samples for which GC-MS analysis revealed any trace of cyclododecane were allowed to continue subliming. The jars were opened to evaporate off the hexanes and ether, and the crushed samples were spread onto aluminum foil sheets to promote air contact. These crushed samples were exposed to room temperature and humidity for five additional days and then were returned to the glass jars. The samples were retreated with hexanes and ether and then reanalyzed as described above. Following this extended sublimation period, no further traces of consolidant were detected in any samples via GC-MS.
Table 2 shows the results of the GC-MS analysis of the stone samples. After four weeks of sublimation, very small amounts of cyclododecane (ion abundances ranging from <25 to <350) were detected in less than half of the treated samples. The detection of these trace quantities of consolidant confirms the viability of the experimental protocol. Although no consolidant was visible on the stone surfaces and the samples had returned to their untreated weights (recorded to 0.00001 g), traces of cyclododecane remained in 6 out of 12 limestone samples and in 5 out of 12 sandstone samples. Those samples treated with the hexanes system most consistently show small amounts of cyclododecane still present on the stone (5 out of 6 samples). Saturated at approximately 140%, the hexanes solution introduces more cyclododecane per applied volume than the other solvent solutions. Comparatively, the Shellsol OMS solution introduces the least amount of consolidant, and fewer of these samples have retained any detectable trace of cyclododecane after four weeks (only 2 out of 6 samples).
Table 2. GC–MS Results after Sublimation of Cyclododecane from Stone Samples
System | Limestone | Sandstone |
| Ion Abundance | Sample # | Ion Abundance | Sample # |
| after 4 wks | +5 days | | after 4 wks | +5 days | |
Untreated | none | none | 1 | none | none | 1 |
| none | none | 2 | none | none | 2 |
| none | none | 3 | none | none | 3 |
Xylenes | none | none | 4 | <350 | none | 4 |
| <50 | none | 5 | none | none | 5 |
| <50 | none | 6 | none | none | 6 |
Hexanes | <200 | none | 7 | <100 | none | 10 |
| none | none | 8 | <50 | none | 11 |
| <350 | none | 9 | <25 | none | 12 |
Shellsol 71 | none | none | 10 | none | none | 7 |
| <50 | none | 11 | none | none | 8 |
| <25 | none | 12 | none | none | 9 |
Melt | none | none | 13 | none | none | 13 |
| none | none | 14 | none | none | 14 |
| none | none | 15 | <50 | none | 15 |
The samples treated with melted cyclododecane received the greatest amount of consolidant, yet 5 out of 6 of these samples show no detectable trace of cyclododecane after four weeks of sublimation. Comparing the results for samples treated with melted cyclododecane with those for the solvent solutions suggests that penetration affects sublimation time. Very little if any penetration occurred with the melted cyclododecane, whereas solvent solutions carried the consolidant well into the substrate. Riedl and Hilbert (1998) instead found that melted applications to heated plaster samples took longer to sublime than saturated solution deliveries of cyclododecane in petroleum ether to unheated samples. Both the quantity of cyclododecane introduced by the melted application and the penetration achieved by heating the plaster samples probably contributed to the longer sublimation times required for the plaster samples. This discrepancy between the sublimation rates of melted cyclododecane on the stone samples and on the plaster samples treated by Riedl and Hilbert further suggests that penetration impacts sublimation time.
The present analyses using GC-MS therefore indicate that complete sublimation from within stone pores may actually require more time than can be gauged visually or by weight loss, especially if delivered as a saturated solution. It is, of course, pertinent to subsequent treatment plans to know when all cyclododecane has left the pore structure of the substrate. Correlation between sublimation time and depth of penetration, as determined by delivery method or by substrate porosity, requires further evaluation. For the series of experiments described above, the same volume of consolidant solution was applied to each sample. By instead establishing experimental parameters to introduce a constant quantity in grams of cyclododecane, rather than a controlled volume of consolidant solution, it may be possible to more accurately assess the sublimation rate for particular substrates and for individual delivery methods.
|