CONTROLS ON STONE TEMPERATURES AND THE BENEFITS OF INTERDISCIPLINARY EXCHANGE
J. P. MCGREEVY, P. A. WARKE, & B. J. SMITH
3 3. LABORATORY STUDIES
3.1 3.1 BACKGROUNG
The laboratory studies described in the following sections both parallel and extend those conducted in the field by examining, under controlled conditions, the effects of surface color change (staining) on stone temperature regimes (Warke et al. 1996) and the effects of direct and indirect heating on different stone types (Warke and Smith 1998). The prompt for examining the former was an interest in the soiling of stone surfaces that are common on buildings and monuments in polluted urban environments and that are cited as being both a cause and an effect of weathering (Smith and Magee 1990). With regard to the latter, it is invariably the case in laboratory-based durability tests (Ross and Butlin 1989) that stone samples are heated and cooled using oven-based (i.e., indirect) methods whereby the temperature of samples is determined solely by the temperature of the surrounding air. This form of temperature control neglects the potential influence of albedo, with the result that all samples are likely to experience the same temperature regime and thus not experience the surface/subsurface temperature differences that may be produced under natural conditions. It was felt, therefore, that the extent to which this approach failed to discriminate among different stone types would be worthy of quantification.
3.2 3.2 METHODS
3.2.1 3.2.1 Experimental Design
The equipment used to examine the effect of staining on stone surface temperature characteristics was specifically designed and constructed to simulate direct heating of stone samples as well as repeated short-term temperature fluctuations associated with, for example, passing cloud cover (fig. 4). It comprises a large drum 75 cm in diameter and 100 cm deep. Three ports located at regular intervals around the circumference of the drum allow access to the interior, and three infrared lamps fixed over the drum provide the heat source. Interruptions to this heat source are achieved by a rotating semi-circular blade driven by a “stepping motor” that can be set to revolve at speeds ranging from 1 rev/8 hours to 16 rev/hour. When, for example, the blade is set to rotate at 2 rev/hour, any stone samples beneath it will experience two shade episodes, each of 15 minutes duration, in every hour. When all three lamps are on, they create within the drum a relatively stable air temperature of 40�C, henceforth referred to as “warm air” conditions that are typical of Mediterranean summer regimes.
Fig. 4.
Diagram of a detail of circular drum used in simulation experiments
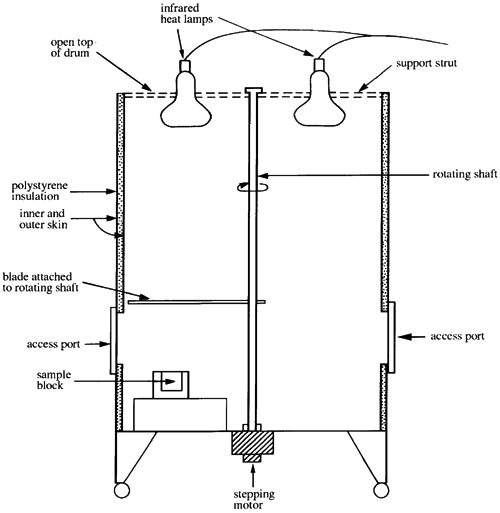 |
Direct and indirect heating effects were investigated using an environmental chamber (Design Environmental 990–20H Chamber) modified by the addition of an infrared lamp positioned approximately 40 cm above the stone samples. As with the drum, short-term temperature fluctuations were also examined, the effect being achieved within the chamber by using a timing control switch to turn the lamp on and off at 15 minute intervals.
Temperatures were measured at 1 minute intervals, and in all experiments bead thermistors and a 12 bit Grant Squirrel data logger were used.
3.2.2 3.2.2 Materials
Stone samples used in the laboratory investigations were prepared as described in section 2.2.1 and depicted in figure 1. The four types selected for the Death Valley field study (Antrim basalt, Mourne granite, Dunhouse sandstone, and Portland limestone) were used in the study of direct and indirect heating effects, while, to investigate the influence of surface soiling on temperature regimes Pentellic marble, Baumberger sandstone, Dunhouse sandstone, and Portland limestone were used. Soiling of test specimens was simulated by fixing a thin dusting of either coal or oil fly ash onto stone surfaces using an aerosol adhesive, producing, respectively, light gray (Munsell notation 10YR 7/2) and black (10YR 2/1) coloration.
3.3 3.3 LABORATORY RESULTS
3.3.1 3.3.1 Soiling as an Influence on Temperature Characteristics
Data presented in figure 5 and table 2 show the differences among the four stone types used in the soiling experiment under warm air conditions. When clean, all four samples present similarly light-colored (high albedo) surfaces, but differences in thermal response characteristics indicate the underlying influence of thermal conductivity. Baumberger sandstone and Portland limestone reached the highest surface temperatures, with the former displaying the greatest surface temperature decrease during each 15 minute period of shade. This finding may reflect a low thermal conductivity and, possibly, poor heat storage in surface layers from where it is rapidly lost once incident radiation is interrupted. Simultaneous surface and subsurface temperature measurements tend to support this relationship, as Baumberger sandstone exhibited the greatest surface/subsurface differences, while Dunhouse sandstone and Pentellic marble showed comparable surface characteristics under these warm air conditions.
Fig. 5.
Surface and subsurface temperature data from insulated clean and soiled stone samples exposed to heating in the circular drum under warm air conditions (Warke et al. 1996) �John Wiley and Sons Limited. Reproduced with permission
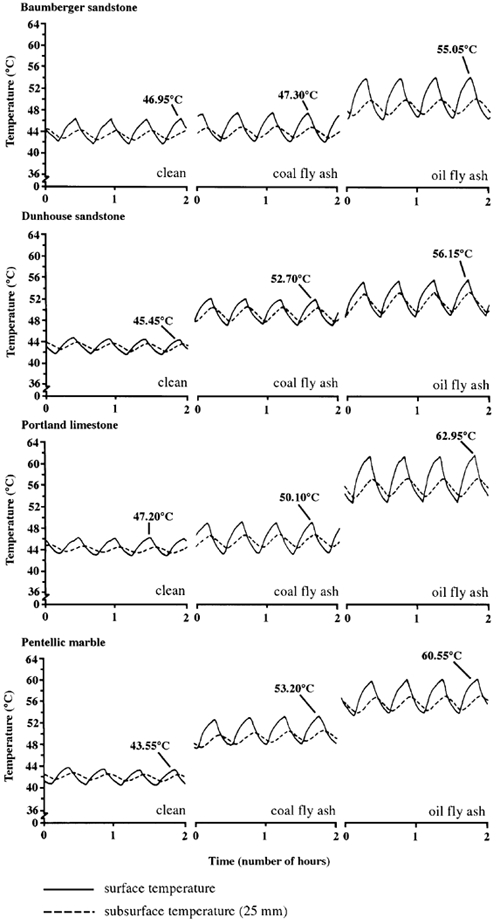 |
Table 2. Rates of Surface Temperature Change for Clean and Soiled Surfaces under Warm Air Conditions
BAUMBERGER SANDSTONE |
| Surface Temp. (�C) | TC after 1st 5 mins (�C) | Rate of TC (�C/mins) | TC after 2d 5 mins (�C) | Rate of TC (�C/min) | TC after 3d 5 mins (�C) | Rate of TC (�C/min) | Total TC (�C) | Avg. TC per 15 min cycle (�C/min) |
Clean surface | 46.95 | 2.55 | 0.51 | 1.35 | 0.27 | 1.15 | 0.23 | 5.05 | 0.34 |
Warm air, coal fly ash | 47.30 | 2.60 | 0.52 | 1.70 | 0.34 | 0.90 | 0.18 | 5.20 | 0.35 |
Warm air, oil fly ash | 55.05 | 3.55 | 0.71 | 2.70 | 0.54 | 1.30 | 0.26 | 7.55 | 0.50 |
DUNHOUSE SANDSTONE |
Clean surface | 45.5 | 1.55 | 0.31 | 1.00 | 0.20 | 0.60 | 0.12 | 3.15 | 0.21 |
Warm air, coal fly | 52.70 | 2.65 | 0.53 | 1.40 | 0.28 | 0.90 | 0.18 | 4.95 | 0.33 |
Warm air, oil fly ash | 56.15 | 8.85 | 0.77 | 1.65 | 0.33 | 0.10 | 0.22 | 6.60 | 0.44 |
PORTLAND LIMESTONE |
Clean surface | 47.20 | 1.15 | 0.23 | 1.10 | 0.22 | 0.85 | 0.17 | 3.10 | 0.21 |
Warm air, coal fly | 50.10 | 3/05 | 0.61 | 1.70 | 0.34 | 0.70 | 0.14 | 5.45 | 0.36 |
Warm air, oil fly ash | 62.95 | 4.85 | 0.97 | 2.35 | 0.47 | 3.15 | 0.63 | 10.35 | 0.69 |
PENTELLIC MARBLE |
Clean surface | 43.55 | 1.35 | 0.27 | 1.10 | 0.22 | 0.65 | 0.13 | 3.10 | 0.21 |
Warm air, coal fly | 53.20 | 2.75 | 0.55 | 1.30 | 0.26 | 2.00 | 0.40 | 6.05 | 0.40 |
Warm air, oil fly ash | 60.55 | 3.60 | 0.72 | 2.45 | 0.49 | 1.85 | 0.37 | 7.90 | 0.53 |
TC = temperature change
|
Surface soiling with both coal and oil fly ash increased the surface temperatures of all four stone types, particularly Portland limestone and Pentellic marble. Portland limestone treated with oil fly ash exhibited surface temperatures more than 15�C higher, increasing during each 15 minute period from 0.21�C min−1 to 0.69�C min−1. In all cases, surface soiling, especially with oil fly ash, increased the magnitude of surface temperature change during each 15 minute period. When shaded, surface temperature of Portland limestone decreased most rapidly, followed by Baumberger sandstone, Dunhouse sandstone, and Pentellic marble. In all cases, approximately half the total temperature decrease occurred during the first 5 minutes of shade. Surface soiling increased radiant heat absorption, raising temperatures well above ambient air temperature such that, when the heat source was interrupted, heat was rapidly lost to the cooler surrounding air.
From the above results and observations, it would appear that soiling affects surface temperatures of light-colored stone in several ways. First, and most obviously, it raises surface temperatures. Second, under conditions of direct heating, it increases the rate of temperature decrease when the heat source is interrupted, especially when thermal conductivity is low. Finally, through a combination of these effects, it enhances the characteristic asymmetrical pattern of surface heating and cooling. Increased rates of heat loss/gain must, in turn, increase the magnitude of thermal stresses developed between individual anisotropic mineral grains and between the surface and subsurface parts of the stone.
An examination of temperature changes with depth reveals that Baumberger sandstone exhibited the largest surface/subsurface temperature differences and the highest average temperature gradient with depth (table 3). This finding suggests that it does not readily conduct heat from surface to subsurface layers. Portland limestone displayed the next steepest gradient, while the data from Dunhouse sandstone and Pentellic marble show reduced surface/subsurface temperature gradients, suggesting more transference of heat from surface to substrate.
Table 3. Rate of Temperature Change with Depth on Clean and Soiled Surfaces under Warm Air Conditions
BAUMBERGER SANDSTONE |
| Max Surf. Temp. (�C) | Simultaneous Subsurf. Temp. (2.5 cm) | Diff. (�C) | Rate of Decrease (�C/cm) | Min. Surf. Temp. (�C) | Temp. Range per Cycle (�C) |
Clean surface | 46.95 | 44.15 | 2.80 | 1.12 | 41.90 | 5.05 |
Warm air, coal fly | 47.30 | 44.35 | 2.95 | 1.18 | 42.10 | 5.20 |
Warm air, oil fly ash | 55.05 | 50.30 | 4.75 | 1.90 | 47.50 | 7.55 |
DUNHOUSE SANDSTONE |
Clean surface | 45.45 | 44.35 | 1.10 | 0.44 | 42.30 | 3.15 |
Warm air, coal fly ash | 52.70 | 50.95 | 1.75 | 0.70 | 47.75 | 4.95 |
Warm air, oil fly ash | 56.15 | 54.05 | 2.10 | 0.84 | 49.55 | 6.60 |
PORTLAND LIMESTONE |
Clean surface | 47.20 | 45.25 | 1.95 | 0.78 | 44.10 | 3.10 |
Warm air, coal fly | 50.10 | 47.55 | 2.55 | 1.02 | 44.65 | 5.45 |
Warm air, oil fly ash | 62.55 | 58.20 | 4.75 | 1.90 | 52.60 | 10.35 |
PENTELLIC MARBLE |
Clean surface | 43.55 | 42.25 | 1.30 | 0.52 | 40.45 | 3.10 |
Warm air, coal fly ash | 53.20 | 50.35 | 2.85 | 1.14 | 47.15 | 6.05 |
Warm air, oil fly ash | 60.55 | 57.00 | 3.55 | 1.42 | 52.65 | 7.90 |
Because soiling, especially with oil fly ash, raises surface temperatures, it also increases temperature gradients within the specimens, particularly in Baumberger sandstone and Portland limestone. Soiling (oil fly ash) increased temperature gradients within these stone types by 70% and 140%, respectively.
The evidence suggests, therefore, that surface soiling of light-colored stone with a low thermal conductivity raises surface temperatures and increases surface/subsurface temperature gradients. Inevitably, these increases must raise thermal stress between surface and subsurface layers and affect microenvironmental conditions at the stone-air interface, increasing the complexity of interactions between weathering activity and stone response in this zone, particularly where soiling patterns are complex and soiled and unsoiled surfaces are adjacent.
3.3.2 3.3.2 Direct and Indirect Heating as an Influence on Temperature Regimes
Comparison of the field data described earlier (see fig. 3) with the results of oven-based (i.e., indirect) heating of specimens of the same four stone types in an environmental chamber (fig. 6) is informative. It is clear that the variations evident under natural conditions of exposure are not replicated by a heating method that results in the pattern of temperature change of the specimens mirroring that of the surrounding air—a drawback given the potential for variation as a result of thermal properties, especially albedo. The relative differences among the stone types encountered under natural conditions can, however, be replicated by using a direct infrared heat source (fig. 7). In this way, the effects of differing thermal properties become evident, with basalt attaining the highest surface and subsurface temperatures and Portland limestone the lowest, despite exposure to the same conditions.
Fig. 6.
Surface and subsurface temperature characteristics of four lithologies exposed to indirect (air) heating in an environmental chamber under simulated hot desert conditions. Reprinted from Warke and Smith 1998, with permission from Elsevier Science.
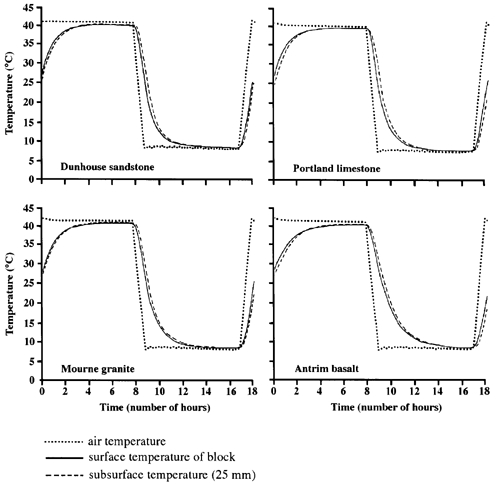 |
Fig. 7.
Surface and subsurface temperature characteristics of four lithologies exposed to direct (infrared) and indirect (air) heating in an environmental chamber under simulated hot desert conditions. Reprinted from Warke and Smith 1998, with permission from Elsevier Science.
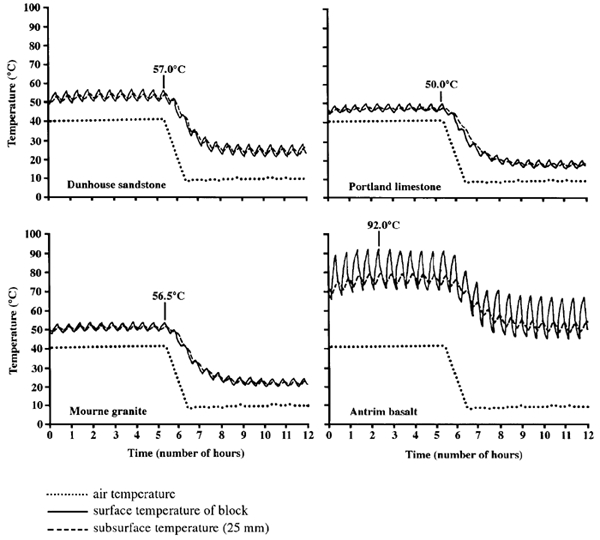 |
|