CONTROLS ON STONE TEMPERATURES AND THE BENEFITS OF INTERDISCIPLINARY EXCHANGE
J. P. MCGREEVY, P. A. WARKE, & B. J. SMITH
2 2. FIELD STUDIES
2.1 2.1 BACKGROUND
Over the past 20 years, researchers based at the School of Geography, the Queen's University of Belfast, have conducted various field studies aimed at gathering stone temperature data from relatively extreme climatic regions: Morocco (Smith 1977; Kerr et al. 1984); Iceland (Douglas et al. 1983); Karakoram Himalayas (Whalley et al. 1984); Tenerife (Jenkins and Smith 1990); and Death Valley, California (Warke 1994; Warke and Smith 1998).
The general purpose of these studies, which complement work carried out by other geomorphologists (see reviews by Smith 1994 and Goudie and Viles 1997), has been to improve understanding of the temperature conditions under which stone weathering occurs. Notwithstanding the intricacies of microclimate (Geiger 1965), it is clear that there are problems in using standard meteorological data on air temperatures, often recorded at some distance from sites of interest, to infer causes of decay. Specifically, the data collected have been used to guide the design of laboratory simulation experiments and to examine the extent to which albedo and thermal conductivity, in particular, influence the temperature regimes experienced by different stone types. It is these specific aims and the information arising from them that may be of relevance to those interested in the deterioration and conservation of monumental stonework.
2.2 2.2 METHODS
2.2.1 2.2.1 Materials
A number of stone types were used in the field studies and laboratory investigations (table 1). These were chosen to represent a range of physical and mineralogical characteristics that allow the investigation of rock property controls on surface and near-surface temperatures. In particular, the rocks display a variety of colors (albedo) ranging from very dark gray basalt to white chalk and marble, with reddish brown and pale brown sandstones, gray granite, and grayish white limestone lying between these extremes.
Table 1. Some Genearl Characteristics of Stone Types Used for Data Collection
Stone Type | Munsell Color Notation (dry) and Color Description | Albedo(%) | Porosity(%) | Average Ultrasound Pulse Velocity (m sec−1 | Thermal Conductivitya (Wm−1 K−1) | General Description |
Antrim basalt | 2.5Y N3/0(very dark gray) | 12 | 1.83 | 6,155 | 0.96 | tertiary olivine-rich, fine-grained basalt with some microfracture development |
Mourne granite | 5YR 6/1 (gray) | 18 | 1.85 | 4,425 | 1.65 | tertiary quartzose, coarse-grained granite with tightly interlocked crystal structure |
Baumberger sandstone | 10YR 7/2 (7/3) (light gray, very pale brown) | NA | 21.40 | 3,004 | N/A | Cretaceous fine-grained clacareous sandstone |
Dunhouse sandstone | 10YR 7/2 (7/3) (light gray, very pale brown) | NA | 18.10b | 2,460 | N/A | carboniferous noncalcareous fine- to medium-grained sandstone with interstitial kaolinite deposits |
Scrabo sandstone(c) | 2.5YR 5/4 (dull reddish brown, but color can be extremely variable) | 12 | 14.80–21.24 (extremely variable) | 2,274 | 1.05 | Triassic noncalcareous, fine to medium-grained sandstone with bedding planes, clay inclusions and lenses (smectites) and variable packing of constituent grains |
Portland limestone | 2.5YR 8/1 (grayish white) White | NA | 17.90 | 3,978 | 1.53 | Jurassic oolitic limestone with bimodal pore size distributions |
Pentellic marble | White (no suitable Munsell notaion) | NA | 0.18 | 5,097 | N/A | Jurassic dense white marble |
Antrim chalk | 5YR 8/1(white) | 25 | 8.27 | 5,192 | 1.72 | Cretaceous compact chalk with tightly interlocking blocky micritic crystals |
NA = not available
a aAlthough specific thermal conductivity values were not obtainable for all eight rock types, it was possible to assign relative thermal conductivity positions on the basis of simultaneous surface-subsurface temperature differences arising from exposure to direct heating under laboratory conditions with the thermal conductivity of Antrim basalt < Scrabo sandstone < Baumberger sandstone < Portland limestone < Mourne granite < Pentellic marble ≤ Dunhouse sandstone < Antrim chalk. This ordering corresponds resonably closely with known thermal conductivity values.
b bLeary 1986
(c) cValues given may not be representative of Scrabo sandstone in general because of its structural and mineralogical variability.
|
2.2.2 2.2.2 Temperature Measurements and Conditions of Exposure
For two reasons, the data presented focus on hot climates (Morocco and Death Valley, California): first, because of the interest in stone deterioration on buildings and monuments located in regions that, in terms of temperatures, are climatically similar (for example, southern Europe, North Africa, the Middle East, Central America), and second, because they relate to the laboratory studies described later.
Comparative investigation of the responses of different stone types to heating and cooling under natural exposure conditions is often constrained by little or no variation in geology at study sites, by the fact that valid comparisons rely on stone surfaces being similarly orientated and inclined, and by the practical difficulties of drilling into stone to allow subsurface temperature measurements. To overcome these obstacles, we have resorted to the use of precut and predrilled blocks, normally 5–7 cm cubes of a range of stone types embedded in expanded polystyrene. The polystyrene jackets promote unidirectional heating and cooling that is characteristic of larger surfaces in natural situations. Because our interests are related primarily to surface and near-surface weathering phenomena, depths of temperature measurement were not great, varying between 2.5 cm and 6.0 cm (fig. 1).
Fig. 1.
Detail of insulated blocks and position of thermistors used in collection of temperature data from Death Valley and laboratory studies, and Southeast Morocco
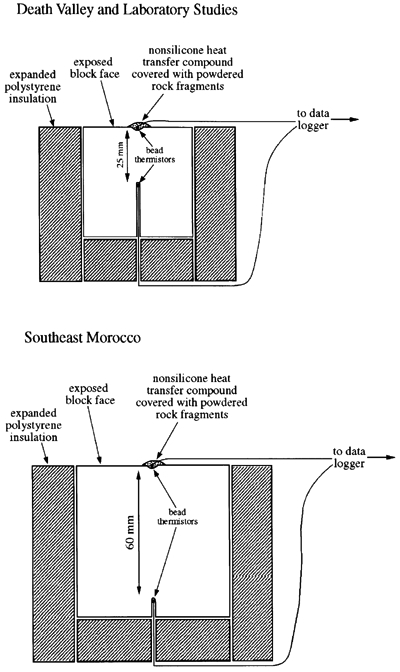 |
Temperatures were measured using various instruments, usually Grant Instrument Squirrel data loggers and bead thermistors. For surface temperature measurements, shallow holes (2–3 mm) were drilled on the exposed sample surface. A bead thermistor was then placed in the hollow and fixed to the stone surface using a nonsilicone heat conducting compound (Heat Sink Compound). The surface of this was then well covered with powdered fragments from the same stone type in order to maintain a uniform surface albedo and prevent any disparity in surface heating. Subsurface temperatures were measured by drilling up from the base of the block to a point 6 cm from the surface in the case of the samples used in Southeast Morocco and 2.5 cm for the blocks used in Death Valley and the laboratory studies.
2.3 2.3 FIELD RESULTS
2.3.1 2.3.1 Southeast Morocco
Figure. 2 depicts surface and subsurface temperatures experienced by blocks of Antrim chalk (white limestone), Mourne granite, and Scrabo sandstone exposed at a site in Southeast Morocco. There are distinct differences between the maximum surface temperatures for each stone type and also between the differences in surface and subsurface maxima. The values for the latter are 0.9�, 1.6�, and 3.0�C for chalk, granite, and sandstone, respectively. These are not, however, the maximum internal temperature differences that occur during the heating phase, which are 3.5�, 4.3�, and 5.4�C, respectively.
Fig. 2.
Surface and subsurface (6 cm depth) temperature measurements for Antrim chalk, Mourne granite, and Scarbo sandstone blocks exposed to natural hot desert conditions in Southeast Morocco
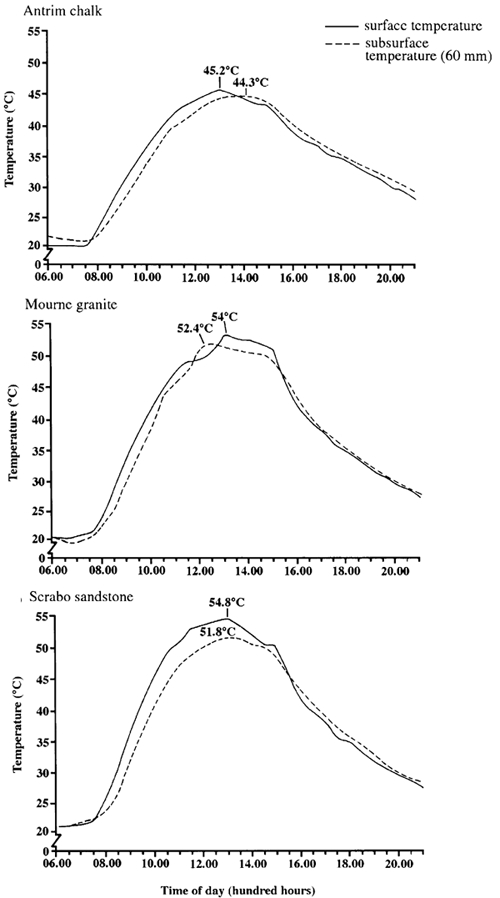 |
Primarily these data can be used to illustrate the control exerted by thermal conductivity (see table 1) on subsurface heating, whereby the stone with the highest thermal conductivity value (chalk) experiences the lowest internal temperature gradient, reflecting enhanced heat transference to depth in comparison to the other stone types. This control is further reflected by the granite, which has a lower maximum surface temperature than Scrabo sandstone but a higher temperature at depth. Absolute surface temperature, however, appears to be controlled more by a combination of surface albedo (coloration) and thermal conductivity. Intuitively, it would be expected that favorable combinations of these would enhance surface heating. Thus a combination of a dark surface and low thermal conductivity should be more conducive to the creation of high surface temperatures than the presence of only one of these characteristics. This speculation is borne out by the data: Scrabo sandstone, with both the lowest albedo and thermal conductivity, attained the highest surface temperature while chalk, with the highest albedo and thermal conductivity, reached a much lower maximum surface temperature. Granite, with intermediate values for albedo and thermal conductivity, exhibited a maximum surface temperature between these two extremes.
2.3.2 2.3.2 Death Valley
The Mourne granite from the Moroccan study was combined with three other stone types—Antrim basalt, Portland limestone, and Dunhouse sandstone—in a similar investigation at a site in Death Valley, California. Again, considerable differences among the lithologies are evident (fig. 3). The effect of dark surface coloration combined with low thermal conductivity is dramatically illustrated by the surface temperatures of the basalt sample (maximum 72.65�C). The contrast with the other stone types, particularly Portland limestone with its light-colored surface and much higher thermal conductivity, is marked. As with the Moroccan data, internal temperature gradients reflect the influence of thermal conductivity, the one varying inversely with the other.
Fig. 3.
Surface and subsurface temperature characteristics of four lithologies exposed to natural hot desert conditions in Death Valley National Monument, California, August 3, 1992. Reprinted from Warke and Smith 1998, with permission from Elsevier Science.
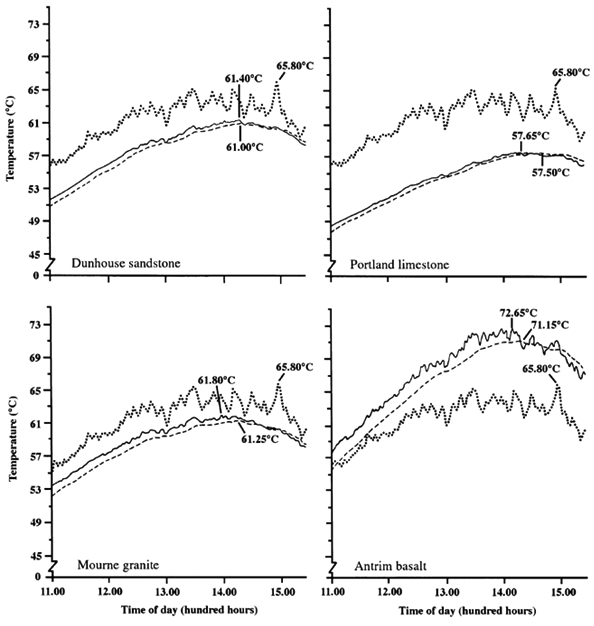 |
|