FIBER-SAFE EXTRACTION OF RED MORDANT DYES FROM HAIR FIBERS
ERICA J. TIEDEMANN, & YIQI YANG
2 THE MORDANT-DYE COMPLEX
The natural red dyes used in this study are madder, Relbunium, and cochineal. Madder, a vegetable dye produced by Rubia tinctorum common to Europe and the Middle East, contains mainly alizarin as well as purpurin, pseudopurpurin, munjistin, and rubiadin (Wouters 1985). Relbunium, from the species Relbunium ciliatum, Relbunium hirsutum, Relbunium hypocarpium, is a related vegetable dye native to South America. Schweppe (1986) identified purpurin and pseudopurpurin as components of Relbunium dye, emphasizing that it contains no alizarin. Wouters and Rosario-Chirinos (1992) also identified xanthopurpurin and munjistin in the extract of a Relbunium dyeing. Cochineal, an insect dye produced by the species Dactylopius coccus, also originates from South and Central America. Its main component, carminic acid, is accompanied by flavokermesic acid, kermesic acid, and four unknown components (Wouters and Verhecken 1989a, 1989b). All the above dyes are anthraquinone derivatives. These and the components of other natural dyes are characterized in Thompson's comprehensive work on natural coloring matters (1971). Excepting flavorkermesic acid, which has been characterized more recently (Wouters and Verhecken 1987), Wouters has conveniently provided all of the structures on one page (Wouters 1985).
Because anthraquinone dyes have only moderate affinity for protein fibers, their fastness was often enhanced by mordants. Mordants, which are metal salts that form an insoluble complex with dye molecules, include potassium aluminum sulfate (alum), copper sulfate, potassium dichromate, and ferrous sulfate. The nature of the mordant-dye complex was clarified in a series of articles on the metal complexes of alizarin (Kiel and Heertjes 1963a, 1963b). Figure 1 shows the structure of the calcium-aluminum lake of alizarin adapted from Kiel and Heertjes. The alizarin molecules are capable of forming six-member chelate rings with aluminum ions. Colored lakes formed by the metal ions and dye molecules resist extraction by water and organic solvents, which readily strip similarly structured acid dyes. The sheer size of the complex may account for some of its insolubility. It is also likely that the large complexes are physically trapped within the fiber.
Fig. 1.
The chemical structures of alizarin (a) and the calcium-aluminum lake of alizarin (b) (adapted from Kiel and Heertjes 1963a)
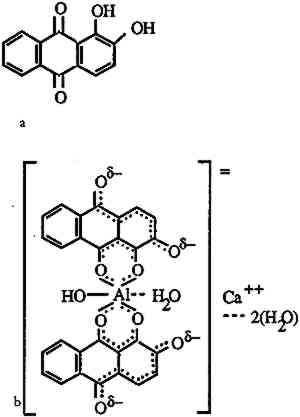 |
The conventional extraction method, using strong acid, probably functions by replacing hydrogen ions for the metal ion, thus releasing the metal ion from the complex. Single dye molecules should then be free to go into solution. With their slight acidic character, however, these particular dyes should not be soluble in strongly acidic solution. By destroying the fiber, then, the acid liberates dye molecules despite their poor solubility.
It has been shown that some of the dye molecules are also unstable at such low pH. Although carminic acid, alizarin, rubiadin, and purpurin are stable in 3 N hydrochloric acid solution in a boiling water bath, pseudopurpurin and munjistin decarboxylate to purpurin and xanthopurpurin even without heating (Wouters 1985).
The proposed extraction method uses EDTA to break up the mordant-dye complex. Like the dye molecules, EDTA complexes with the metal ion. EDTA, however, will react with metal ions in a 1:1 ratio because it supplies six pairs of electrons (West and Sykes 1958). Because it is a stronger chelating agent than the hydroxylated anthraquinone dyes, EDTA complexes preferentially with the metal ion. With the metal ions masked by the EDTA, dye molecules are free to go into solution.
During preliminary testing, however, it was quickly discovered that EDTA alone in water would not extract the dye. A mixture of methanol and water was the next option because methanol has been used consistently in the acid extractions and to dissolve dried samples of the dyes. This mixture, too, gave unsatisfactory results. DMF, a polar aprotic solvent often used to extract acid dyes, mixed in a 1:1 ratio with the EDTA solution did extract the dye.
Clearly, the dyes are soluble in water because that is the solvent used for extraction from the natural source as well as for dyeing. As mentioned above, they are also soluble in methanol. Nevertheless, despite the binding of the mordant to EDTA, the dyes will not move appreciably from the fiber into water alone. As demonstrated by the successful dyeing, the dyes have affinity for the fiber in water.
Although polar interactions are important to the solubility of the dye in water as well as the attraction between dye and fiber, hydrophobic interaction is the main force involved in the dye's affinity for the fiber. The dye's large aromatic system is responsible for this kind of attraction. Due to hydrophobic interaction, the dye-fiber attraction is greater than the dye-water attraction despite the dye's solubility in water. With the help of EDTA, the dye's solubility increases in water/DMF (1/1 v/v) because the dye-DMF attraction is greater than the dye-fiber attraction.
It should be noted that EDTA solubility decreases drastically in the water/DMF solution. A concentration of 0.1% EDTA was effective for the dye extractions and precluded precipitation.
Altogether, the organic acid and the solvent should have little effect on protein fibers (Trottman 1975).
|