AN EXAMINATION OF THE PATINA AND CORROSION MORPHOLOGY OF SOME ROMAN BRONZES
DAVID A. SCOTT
4 PROCESSES OF CORROSION
Geilmann's paper (1956) was the first full account of the corrosion of bronze artifacts in which the principal remaining component is stannic oxide. Geilmann employed wet chemical analysis to investigate the elemental or element oxide concentrations of 12 bronzes, examining in turn the analysis of completely corroded objects, samples in an advanced state of deterioration, samples of patina, dissolution of copper from the patina, fixation of metallic constituents that had been dissolved from the patina or bronze, and the process of corrosion of bronzes in the absence of chloride ions. In his examination of objects in an advanced state of corrosion, Geilmann analyzed an urn from a grave site dating to the second or third century A.D. and a sword blade of the Middle Bronze Age dating to about 1000 B.C. from Germany. The sword blade retained a marker layer, or original surface, even when only hydrated tin oxide remained. The urn, on the other hand, had a thin, dark green patina and an almost white tin oxide layer underneath this green surface, which retained the shape of the object. A representative set of these analyses is given in table 6. Geilmann deduced that the absolute tin content of these bronzes was unchanged during corrosion and that no arsenic or antimony were lost either. As a result of interactions with soil minerals, iron and aluminum levels were increased in the patina layer. Geilmann's data, while showing that some objects retain a copper-rich patina, also revealed that others have copper leached from the surface zone until only hydrated tin oxide remains; this hydrated tin oxide layer is then stable to further attack by soil groundwaters. Geilmann states:
The formation of these patinas in burial is ascribed to oxygen and carbon dioxide carried by groundwaters. The salts present in soil solutions can influence the formation of the patina and the nature of the corrosion, but on the whole are of minor importance. The dissolution of copper and other divalent ions is solely due to the free carbon dioxide in the water and the progress of this dissolution is dependent on the concentration of carbon dioxide in solution. If this concentration is high, as for example in humus or porous sandy soils, then the patina is formed rapidly and the continuing dissolution of copper results in an end product of pure tin oxide, whereas in other soils, such as clay, bronzes of the same age may only be covered in a thin patina. In humus-rich sandy soils with a low lime content, there is no inhibiting effect on the decomposition of the patina to create a tin oxide surface, while in lime containing soils, where the soil solutions may contain calcium bicarbonate, this decomposition is inhibited.
Experimental dissolution studies by Geilmann showed that copper would be lost from a synthetic laboratory mixture of copper carbonate and tin oxide. In addition, salts of trivalent iron and aluminum may also contribute to tin oxide enrichment if they are present in the burial environment, and the copper carbonates are preferentially dissolved while iron or aluminum hydroxide is precipitated, accounting for the increase in these metallic ions in the patina. Analyses of soil samples taken from the earth surrounding the object from burial showed the fixation of most metals from the bronze with the exception of arsenic, antimony, and tin. Geilmann proposed the formation of cuprite as the first corrosion product and the corrosion of both the copper and the tin content, followed by dissolution of copper in the oxidation zone of the burial:
If this proceeds, the copper is dissolved by carbon dioxide containing waters, so that finally pure tin oxide remains which combines with various compounds from the soil…. The degree of this decomposition depends on the ratio of oxygen and aggressive carbon dioxide in the groundwaters. If oxygen is missing the decomposition will be slow, even in the presence of high amounts of carbon dioxide…. If there is a lot of oxygen, but little carbon dioxide, then the metal is often oxidized and covered with a carbonate patina.
These corrosion processes, Geilmann convincingly argues, occur in the absence of chloride ions, and his analytical results support this interpretation. In discussing the corrosion of Chinese bronzes, which may also have tin oxide–enriched patinas, Chase (1993) draws attention to the work of Robbiola (1990). Robbiola proposed that the dissolution of copper depends kinetically on the mass transport of copper from the alloy by events that are either under cationic control or anionic control. This model results in a precipitation-dissolution mechanism, after which various other reactions become possible. Anionic control, for example, in the presence of chloride ions produces greater volume change and less coherent corrosion layers. Cationic processes result in the diffusion of metallic components becoming the rate-determining step. This latter process generally results in compact corrosion layers that may show pseudomorphosis.
Several papers have proposed schemes for the explanation of corrosion structures and possible mechanisms (Fink and Polushkin 1936; Cushing 1965; Graedel 1987). Structures may be classified on the basis of their morphological or descriptive characteristics or on the basis of their presumed mode of origin. Both of these approaches involve some compromise. Descriptive classifications such as those used by Fink and Polushkin provide little information about the genesis of structures. On the other hand, genetic classifications may be misleading; some structures could easily form by more than one process or by a mixture of processes that would be genetically distinct. With an awareness of these difficulties, a general conclusion can be made as a result of the present study that there are at least two distinct forms of warty corrosion:
- pustules of corrosion associated with cuprous chloride or the copper trihydroxychlorides as a result of the subsequent transformation of the cuprous chloride. This cuprous chloride is often at the interface between the patina layer and the base of the pustule (Tennent and Antonio 1981; Scott 1990).
- pustules of corrosion with cuprite and malachite with no chloride ion content. These pustules may be associated with tin oxide–enriched patinas in which the corrosion process has proceeded in soils that do not contain significant amounts of chloride ions. The preservation of metallic components, such as alpha + delta eutectoid phase, may also be seen.
A model of pitting corrosion applicable to the former case was developed by Lucey (1972). The original study was initiated to investigate the corrosion failure of copper boilers in England. Lucey proposed that the cuprite that develops at the surface functions as a bipolar electrode, allowing chloride ions to diffuse through the cuprite layer where a film of cuprous chloride develops close to the metal surface. On the outer surface of the cuprite, diffusing copper reacts with carbonate species to build up a mound of corrosion products.
The cuprous chloride that forms in this case is metastable and can react further to produce paratacamite or atacamite with disruption of the patina or of the corrosion pustule, especially if the surface is cleaned and the cuprous chloride is exposed to the atmosphere. In many archaeological examples, cuprous chloride may also be present in the pustule itself along with cuprite, often covered with a cap of malachite, azurite, or one of the copper trihydroxy-chlorides (fig. 29).
Fig. 29.
Pitting corrosion in copper (Source: Lucey 1972). The marker layer is the original surface.
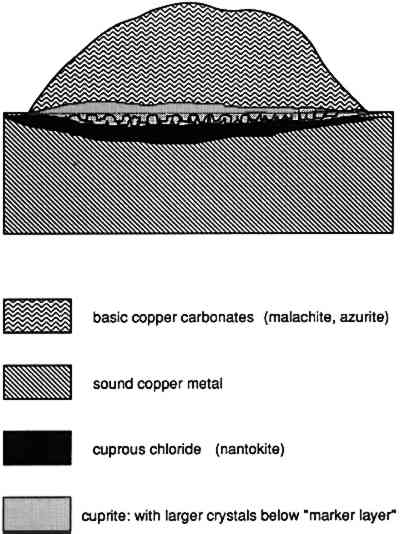 |
In the case of the bronzes examined here, the corrosion pustule appears to originate in the formation of cuprite septa, which pass across the tin oxide patina, allowing the diffusion of copper to occur at particular locations. The tin oxide layer that develops is probably hydrated, and at varying times may be liable to dehydrate to some extent during burial. As a result of the cracking of this layer that would accompany partial dehydration, further loss of copper could occur as septa of cuprite move through the tin oxide patina and relay copper ions to the exterior. These cracks can be seen in figure 25, passing through the tin oxide layer at the bottom of the photomicrograph. The expansion of the corroded matrix of the original bronze is shown by the existence of the lead carbonates and particularly by the relict fragments of alpha + delta eutectoid phase toward the outer surface of the pustule. This localization of extruded corrosion products argues for the continued corrosion of the bronze surface as part of the enrichment in tin as copper is preferentially lost. The preferential loss of copper and the corresponding precipitation contiguous to the tin oxide patina can be seen in figure 11, where isolated and sometimes coalescing dark green corrosion preserves an interdendritic pseudomorphosis. To account for this formation, exterior to the metal itself, the continued corrosion of the substrate would be necessary, with loss of copper through the dendritic pseudomorph of the tin oxide patina and the corresponding precipitation of copper exactly in the location where it passed through the patina layer. In contrast to many of the corrosion processes that occur in iron, copper corrosion products may exemplify epitaxy. In epitaxial processes, there is often a direct morphological correspondence between the substrate on which the corrosion or phase develops and the structure or orientation of the corrosion itself. The same linear extension of copper ions may be responsible for the formation of the fibrous malachite crystals seen in many areas on the surfaces of these objects, although part of the corrosion present on the surface and on the edge of the pustules is also fibrous, and local growth conditions in this case may be the deciding factor rather than the mode of copper loss from the object itself.
The microstructure of the pustule is not simple, and it is difficult to reconstruct every aspect of the growth of this kind of corrosion, as can be gauged from figures 20–27. The presence of euhedral crystals of cuprite (fig. 24) suggests a slow growth process during the transformation of metallic ions into corrosion products, allowing these crystals to form in a background of malachite. The lead that is present in the alloy as discrete, small lead globules has been carried outward into the pustule. There it, too, has become carbonated and converted to cerussite, with considerable expansion in volume, which accounts for the relatively large size (10–35 μm across) of these lead-rich zones in the corrosion. The tin-rich region of the eutectoid is preferentially preserved, while the copper-rich region becomes converted to corrosion products, principally cuprite. A simplified diagrammatic view of the pustule is shown in figure 30, and a summary of the analytical data that were obtained by EPMA for the pustule is given in table 4.
Fig. 30.
Diagram of corrosion pustule, Togati bronze
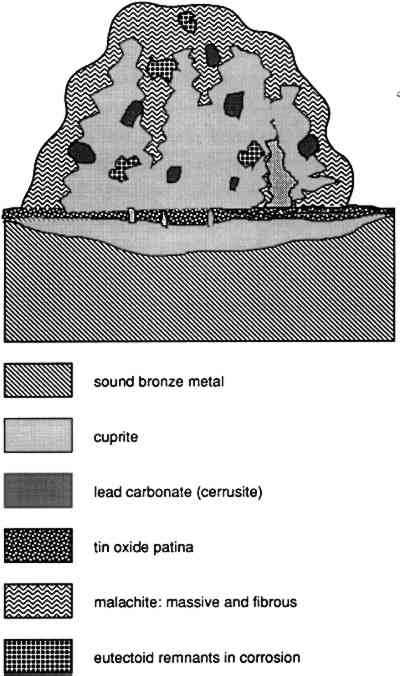 |
Some of the salient features are the low levels of chlorine detected and the presence of some phosphorus—and, by inference, phosphates—in the tin oxide analyses shown in points 16, 17, and 18 of table 4. Point 11 gives an analytical result for the relict eutectoid phase and shows that this material is uncorroded, with a tin concentration found by analysis very close to the theoretical amount of tin in this component of the original alloy, as derived from the equilibrium diagram (Cu31Sn8 contains about 32.6% tin). The localized concentrations of lead carbonate in the structure of the pustule arise from the conversion of individual lead globules in the original metallic matrix. These lead carbonates are not broken up in the process of corrosion but remain as localized patches from the dispersal of the original lead globules through the tin oxide patina. The areas of enrichment in tin oxides give analytical totals in the 60–75% range, which is quite typical; the remainder of the total is oxygen that cannot be analyzed with the electron microprobe used in the study. The data of table 4 show that both cuprite and malachite are fairly pure with no significant impurities. The presence of some residual fragments of the original tin oxide patina in the corrosion pustule were analytically determined and can be seen as veined patches in figure 25.
|