AN EXAMINATION OF THE PATINA AND CORROSION MORPHOLOGY OF SOME ROMAN BRONZES
DAVID A. SCOTT
3 ANALYTICAL STUDIES
3.1 MICROSTRUCTURE OF THE METAL
No metallographic samples could be taken from the bronzes without noticeable damage. Nevertheless a decision was made to remove a sample from the back of the Roma in the vicinity of the casting core hole, specifically to study the unusual hexagonal network structure. The sample was taken with a diamond-tipped core drill, 1.5 mm in internal diameter, with periodic cooling and lubrication with a metal-cutting fluid. The core drill sample was removed from the surface by dislodging with a scalpel and mounted and polished in the usual way. Toward the top of the metallographic section (fig. 19), an area of eutectoid is seen isolated within the corrosion layer, showing that the copper-rich alpha phase of the bronze is preferentially corroded with preservation of eutectoid relicts. The micro-structural study clearly shows that the tin-enriched surface has not been formed by the corrosion of a deliberately tinned surface layer, and as a result of work to be discussed below it is apparent that tin-enriched patinas can form by natural burial processes. The alpha grains are equiaxial and bordered by eutectoid fringes. This part of the structure is not obviously dendritic, and it has an appearance of an annealed casting, which could well be caused by the casting-on of the legs and feet.
Fig. 19.
Metallographic section of Roma microstructure showing eutectoid preservation and preferential corrosion of α solid solution. Etched with alcoholic FeCl3. 200x
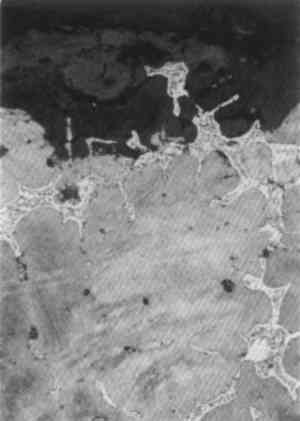 |
The original dendritic and cored microstructure could have been altered by heating during casting on to produce a microstructure more typical for annealed bronzes. Evidence for changes in structure of this type have been seen in other examples where casting-on has been employed, as, for example, in a dagger handle from Luristan, Iran (Scott 1991, app. F, 94). Since casting-on was also used in the Togati and probably the Venus, and since all the bronzes have a similar composition, there is no reason to suppose that their structures are very different from each other.
3.2 COMPOSITIONAL DATA
Detailed analytical results for the composition of the metal are given in tables 1, 2, and 3. These results were obtained by atomic absorption spectrophotometry and by inductively coupled plasma-mass spectrometry. As shown in table 1, the tin content of these objects varies between 6.6–9.5%, lead 1.8–2.7%, and copper 85.7–89.8%. In both the Togati and Nike(tables 2, 3), trace amounts of antimony, arsenic, barium, bismuth, cobalt, gold, iron, nickel, palladium, rhodium, silver, and zinc were detected. Both major and minor components are quite representative of the composition of Roman bronzes of this period (Craddock 1983), and the extraordinarily interesting nature of their surfaces is due entirely to the effects of the burial environment rather than to compositional uniqueness.
TABLE 1 COMPOSITION OF ROMAN BRONZES, DATED A.D. 40–68
TABLE 2 COMPOSITION OF THE BRONZE GOGATI, J. PAUL GETTY MUSEUM
TABLE 3 COMPOSITION OF THE THE BRONZE NIKE, CLEVELAND MUSEUM OF ART
3.3 WARTY CORROSION
A pustule of corrosion from the Togati was removed intact, mounted, and polished so that a cross-sectional view of its structure could be studied and analyzed by electron probe microanalysis (EPMA). In addition, nondestructive x-ray fluorescence (XRF) analyses were carried out on the objects themselves with the Kevex 0750A spectrometer utilizing a barium-strontium secondary target and energy dispersive analysis.
The XRF and XRD analyses of the surfaces of the Togati indicated that a very high proportion of tin remained in the patina, which was about 60–70% tin oxide, a proportion of tin much higher than the tin content of the metal itself.
Darker, mottled areas in this patina fig. 9) were found to have a higher iron content than lighter, contiguous areas. Examination of the clean patina of the Roma showed the same enrichment in tin and depletion of copper. The matte appearance of the patina is therefore partially explained by this loss of copper. Tin-rich patinas have been observed previously in Roman bronzes, in particular, from the classic work of W. von Geilmann (1956), which will be discussed in section 4. He observed tin oxide-rich patinas on bronzes that contain only modest amounts of tin themselves (table 6). Such patinas on these bronzes are reported less commonly than the more often encountered malachite, cuprite, or sulphidic patinas.
TABLE 6 COMPOSITION OF METAL AND CORROSION OF TWO BRONZES FROM THE EUROPEAN BRONZE AGE: GERMANY
The pustule from the Togati, which shows a complex microstructure, was examined by EPMA; analytical data are given in table 4 and back-scattered electron images taken by a scanning electron microscope are shown in figures 20–27. Figure 20 shows the pustule at low magnification. Part of the structure on the right side contains a vacuole, surrounded by malachite crystals, which appear very dark. The lower part of the figure represents the surface adjacent to the tin-rich patina, and the top of the figure represents the outer surface of the pustule. One surprising observation was the existence of metallic remnants of alpha + delta phase eutectoid at the outer extremity of the pustule, a considerable distance from the metallic core of the Togati, and 1 mm above the tin oxide patina. The apparent layering of the pustule, seen under low-power binocular microscopy, does not correspond to any stratification in the corrosion products of the pustule, as is evident from the figures shown here. The spread of lighter particles seen in figure 20 are lead carbonates, while the gray mid-tones are mostly cuprite interspersed with malachite.
TABLE 4 COMPOSITION OF CORROSION PUSTULE FROM THE BRONZE TOGATI
Fig. 20.
Back-scattered electron image of the pustule removed from the Togati. The lower region shows part of the surface of the tin-rich patina. The outer regions are mostly malachite. Complex cuprite growth (lighter gray) and lead carbonate and tin-rich metallic eutectoid relicts (white and pale-colored) can be seen. 45x
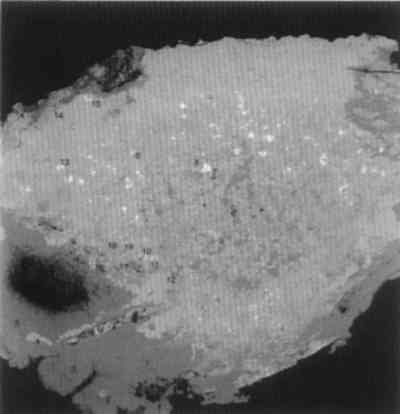 |
Fig. 21.
Back-scattered electron image of the outer surface of the corrosion pustule showing the scalloped and curved profile of the pustule, which is composed of a number of curved fibrous crystals of malachite. The incipiently curved nature of this crystal growth is interesting and is obviously related to the fibrous malachite crystals observed on the surface in free-growing regions. The bar represents 10 μm.
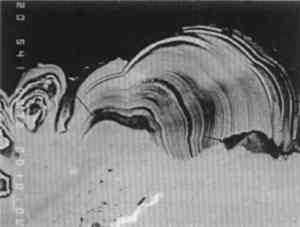 |
Fig. 22.
Back-scattered electron image of part of the vacuole shown in figure 20, right. Acicular malachite crystals can be seen intergrown with some cuprite and a complex mixed assemblage to the right. The bar represents 100 μm.
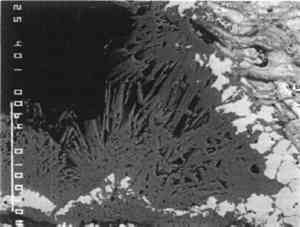 |
Fig. 23.
Back-scattered electron image of the corrosion pustule showing part of the structure from the base of the pustule to the mid-region. This area marks a transition from a more compacted cuprite-rich zone to an area of euhedral cuprite oriented in strands and surrounded principally by malachite. The white phase is lead carbonate, and some α + δ eutectoid regions can also be seen. The bar represents 100 μm.
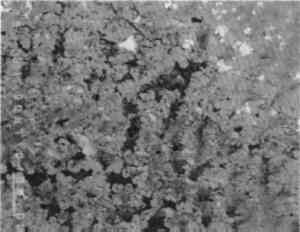 |
Fig. 24.
Back-scattered electron image of euhedral cuprite crystals growing into the corrosion matrix and surrounded principally by malachite. The bar represents 10 μm.
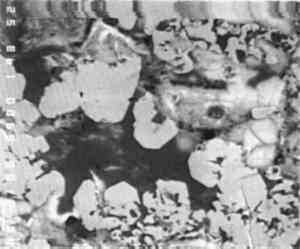 |
Fig. 25.
Back-scattered electron image of the pustule showing part of the base to the mid-region. Note the fissured structure of the tin-enriched layer at the base now interspersed with malachite and cuprite septa. The bright white patches are lead carbonate after original lead globules. Lighter gray particles with interpersed small holes are the remnants of α + δ eutectoid. As the corrosion has developed, part of the rin-rich patina has become detached and “floated” upward into the pustule. The scattered euhedral cuprite crystals can be seen with fine veining in cuprite passing through the malachite. 215x
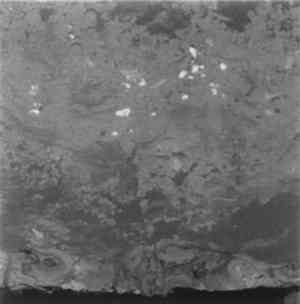 |
Fig. 26.
Back-scattered electron image of a partial side view through the pustule. The bright inclusions represent lead carbonate, and the gray particles are remnants of the α + δ eutectoid phase. The predominantly gray matrix is cuprite, and the darker material toward the right is principally malachite. The bar represents 100 μm.
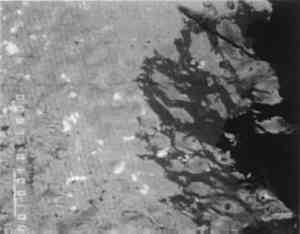 |
Fig. 27.
Bcak-scattered electron image at low magnification showing part of the upper surface of the corrosion pustule and illustrating the presence of some lead carbonates and α + δ eutectoid remnants in a mixed cuprite and malachite matrix. One of the remarkable observations made on this pustule is the presence of α + δ corroded metal remnants at the extreme outer top most surface of the corrosion pustule. The bar represents 100 μm.
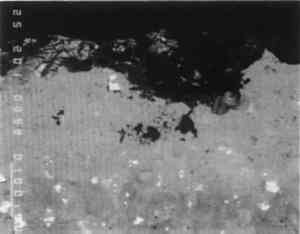 |
Acicular malachite crystals surround the vacuole, shown in figure 20, while the fibrous malachite in the back-scattered electron image in figure 21, toward the edge of the pustule, accounts for some of the apparent layering as each curved set of fibers undulates in and out of the mass. A cluster of acicular malachite crystals in another region of the pustule can be seen in figure 22.
The central part of the pustule is shown in figure 23. It consists of interconnected ribbons of massive and euhedral cuprite passing upward away from the patinated surface. Interspersed in this cuprite are isolated patches of cerussite, islands of alpha + delta eutectoid and malachite, the latter becoming more prevalent toward the outer surfaces of the pustule. The well-crystallized nature of this cuprite can be seen in figure 24. Figure 25 shows an interfacial region between the tin-rich patina and the pustule as it grows from the patinated surface. Views of one of the edge areas of the pustule (fig. 26) and the top of the pustule (fig. 27) show the extent of malachite growth toward the edge. In figure 27, near the upper left, is an isolated remnant of alpha + delta eutectoid, which does not appear as bright as the lead carbonate crystals because the higher atomic number of lead results in enhanced contrast. Significantly, there are no chlorides within the pustule, nor are there any chloride-containing species within the patina layer.
The microstructure of the brick or tile fragments that are embedded in corrosion in all four objects is essentially the same as the sample microstructure taken from the Venus(fig. 28). The fabric of the brick is relatively homogeneous and consists essentially of silica and iron oxides with relatively low amounts of aluminum, sodium, magnesium, potassium, and calcium; these analyses were obtained on brick samples from the Roma and the Venus, and the results are given in table 5.
|