THE EFFECTS OF RELATIVE HUMIDITY ON SOME PHYSICAL PROPERTIES OF MODERN VELLUM:
ERIC F. HANSEN, STEVE N. LEE, & HARRY SOBEL
2 BACKGROUND
There are three primary concerns in the preservation of objects containing the protein collagen. The first is the immediate or short-term effects of the environment, primarily associated with temperature and relative humidity, on the physical properties such as strength, flexibility, permeability, and dimensional changes. The second is the long-term effects of the environment, associated with temperature, relative humidity, oxygen concentration, chemical pollutants, and radiant energy (light), in causing “aging,” or chemical modifications of the original material. Also important is how the environment allows biodeterioration by microorganisms and fungi of such a rich nutrient source.
The most commonly encountered recommendations in the conservation literature for the storage and display of parchment are in the region of 50% RH to 65% RH. However, recommendations vary and include unspecified levels such as “complete dryness” for the display of parchment and vellum: “The emphasis must be toward complete dryness with no excessive changes in temperature and humidity” (Bloodworth and Parkinson 1988, 65). A recent review of the deterioration of skin in museum collections concludes, “Understanding of the complexities in preserved whole skin does not yet allow for adequate specification of ‘ideal’ conditions that will prevent deterioration” (Horie 1990, 109). The discussion of an “ideal” condition of relative humidity presented in this article is a general one relating to a broad class of objects containing collagen as a principal component (such as parchment documents) and for which the conservation aim is the long-term preservation of the collagen. It is presented with the understanding that there may also be other aims (relating either to aesthetic considerations or some use-related function of a skin artifact) or other considerations (including the environmental requirements of other component materials of a composite object or the effects of additives) that must be considered in arriving at an “optimum” concentration of atmospheric moisture.
2.1 SOME PROPERTIES OF PARCHMENT
Parchment and leather are both processed from the skin of animals; “vellum” is a designation for parchment made from the skin of young or unborn calfs. The history, techniques of manufacture, and properties of parchment have been extensively reviewed by Reed (1975). In general, animal skins for both parchment and leather are dehaired through soaking in baths of various compositions and scraping of hair from the surface. At this stage, parchment manufacture differs from leather manufacture. The unique properties of parchment are due to drying the wet skin under tension; the tension causes an alignment of the three-dimensional fiber network of the skin in a configuration more parallel to the surface of the skin. In contrast, the properties of leather are due to joining of the fiber network by chemical links introduced through tanning agents. The “leathered” skin excludes water from the fibers and is firmer and stronger as a result of tanning.
Parchment, then, has a more aligned structure than leather, which has a complex, highly randomized weave structure. Differences resulting from the two different processes, as noted by Reed (1975) are that:
- Collagen fibers from parchment have a lower shrinkage temperature than collagen fibers from leather. (Collagen fibers exhibit a sudden shrinkage in length when heated. Fibers in leather have had chemical cross-links introduced which raise the temperature at which the fibers shrink (Haines 1987).)
- Parchment soaks up water rapidly and in greater amounts than leather.
- Wet parchment has poorer resistance to micro-organisms than leather but greater resistance if kept dry.
2.2 EFFECT OF ATMOSPHERIC OXYGEN AND WATER ON COLLAGEN
Because parchment is processed from the skin of animals, its major constituent is insoluble collagen (which constitutes about 95% of defatted, dehaired skin, the percentage depending upon the age and sex of the animal). Therefore, the chemistry and structural stability of collagen at different levels of water content and resulting from different levels of relative humidity need to be considered.
As collagen ages, in vivo or in vitro, atmospheric oxygen plays a role in its chemical modification (Sobel and Hansen 1989), Oxidation will change the molecular structure of the proteins, causing cross-linking or chemical modifications of several amino acids over time. Changes in parchment resulting from oxidation might also be influenced by the amount of residual fats and the type and amount of additives (such as softening agents) in historic parchment.
Water affects collagen in several different ways. It plays a direct role in the chemical modification of collagen through hydrolysis, solvation of free radicals, hydrogen bond stability, and the rate of gelatin formation.
Collagen is composed of three similar strands of a protein containing in greatest amount glycine, alanine, proline, and hydroxyproline. The spatial configurations of repeated triple amino acid sequences involving glycine, alanine, and hydroxyproline or proline are responsible for the helical conformation between the strands. A triple strand of the protein is entwined in an alpha helix termed tropocollagen, shown in figure 1. Water molecules are intimately connected with the hydrogen bonding holding the triple helix together.
Fig. 1.
Triple helix of collagen (cross-linked to another molecule from peptides at end of molecule). (Reprinted with permission from Scott 1986)
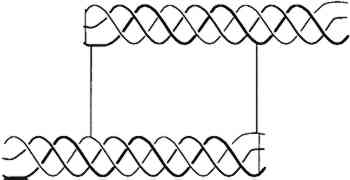 |
It is well known (Bull 1944; Dole and McLaren 1947) that water relates to collagen in four different processes, depending upon the vapor pressure (relative humidity), as shown in table 1. A definite amount of water is necessary for the molecular stability of the tropocollagen structure; this role for water is demonstrable in the region from <1% to 25% RH. From approximately 25% to 60% RH the water is sorbed on hydrophilic sites in the proteins; above 60 % water is sorbed on polymolecular layers; and near saturation, free water exists.
TABLE 1 TYPES OF BOUND WATER ASSOCIATED WITH COLLAGEN AT DIFFERENT RELATIVE HUMIDITIES
The tensile properties of collagen are a function of moisture content. Water sorption is also intimately involved in dimensional changes (swelling) and the permeability of the material.
2.3 GELATINIZATION OF COLLAGEN
In addition to chain-breaking of a macro-molecule by hydrolysis resulting in a decrease in molecular weight, another mode of protein degradation can occur through denaturation. In the case of collagen, this denaturation occurs by interaction with water and is designated gelatinization (fig 2). By heating collagen in water, the water molecules can gain sufficient energy to compete for the hydrogen bonds maintaining the triple helix configuration.
Fig. 2.
Denaturation of collagen
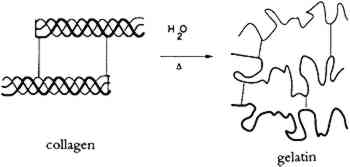 |
The rate of denaturation depends to some extent upon the intactness of the collagen fibrils that make up the fibers in skin. The tropocollagen molecules are cross-linked, end to end, and bundled together to form fibrils in the arrangement shown in figure 3. In skin, these fibrils form fibers that, in a three-dimensional array, are the primary component of skin. When the fibrillar structure is disturbed, as when the fibers are mechanically broken, collagen is more susceptible to attack by bacteria (Rebricova and Solovyova 1987).
Fig. 3.
Collagen molecules line up to form a fibril in “quarter-staggered” array. (Reprinted with permission from Scott 1986)
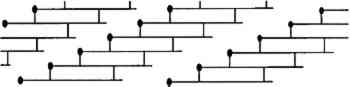 |
Bowes and Raistrick (1967) studied the effect of relative humidity and pH upon the extent of hydrolytic breakdown of collagen and glutaraldehyde-tanned collagen by determining the release of N-terminal residues. At 40�C for 8 weeks, hydrolysis increased for both collagen and tanned collagen with increasing humidity (in the range from 40% to 100%) and decreasing pH (in the range from 5 to 2.5). They concluded that when hydrolytic breakdown becomes extensive, denaturation follows and in turn accelerates further hydrolytic action.
Measuring the effect of temperature on the deterioration of collagen is hampered because temperatures at or above the shrinkage temperature may give unreliable results (Bowes and Raistrick 1964). Of particular interest is whether physically or chemically deteriorated collagen will deteriorate at a faster rate, but this has not been demonstrated and is a matter for further study. What is known is that denaturation of disrupted collagen is promoted by less extreme conditions than those that initiate denaturation in intact collagen. The physical and chemical integrity of collagen can be indicated by the hydrothermal stability, as shown by a decrease in the shrinkage temperature for deteriorated fibrils (Young 1990).
|