RECENT SCIENTIFIC RESEARCH IN PAPER CONSERVATION
DIANNE VAN DER REYDEN
2 PAPER PROPERTIES
Burgess and Binnie (1990) advise that “The evaluation of scientific results and recommendations in conservation literature is greatly aided by a good understanding of the rationale behind the selection of test materials and methods.” An understanding of the rationale behind research design is enhanced by a knowledge of paper properties and their reaction to relative humidity, temperature, radiation, and pollution. These environmental factors initiate degradation mechanisms of hydrolysis, oxidation, and crosslinking (Table 1). These degradation mechanisms can be detected by various tests (Table 2), which measure the chemical, physical, and optical properties of paper. The following summary presents a few basic terms and concepts relevant to these properties. (Optical properties are a type of physical property and can be used to monitor chemical change. They are covered separately here for purposes of organization.)
TABLE 1 SOME ILLUSTRATIVE EXAMPLES OF COMMON CELLULOE REACTIONS
TABLE 2 TESTS USED TO MEASURE THE EFFECT OF CERTAIN REACTIONS ON SOME CHEMICAL, PHYSICAL, AND OPTICAL PROPERTIES OF PAPER
Table. 1. SOME ILLUSTRATIVE EXAMPLES OF COMMON CELLULOSE REACTIONS
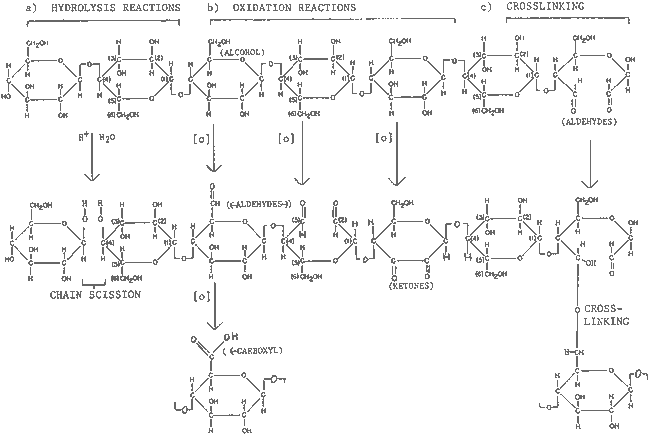 |
Table. 2. TESTS USED TO MEASURE THE EFFECT OF CERTAIN REACTIONS ON SOME CHEMICAL, PHYSICAL, AND OPTICAL PROPERTIES OF PAPER
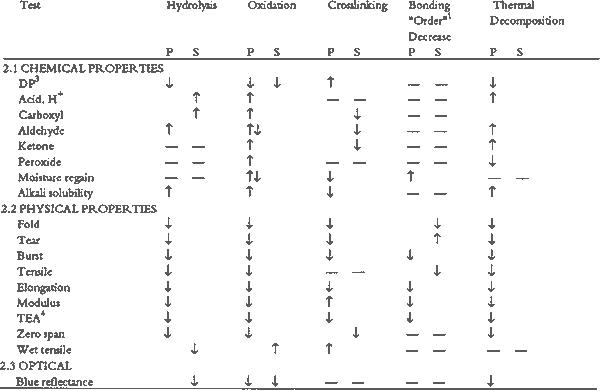 |
2.1 CHEMICAL PROPERTIES
Chemical properties of a paper sheet are derived primarily from variables of furnish or pulp components and from formation or manufacturing processes (Dwan 1987). Furnish variables include the fiber type; pulp stock; refining techniques such as beating or bleaching; and composition including fillers, sizes, coatings, and colorants (Priest 1989a). Formation variables include basis weight, calendaring, and manufacture variables such as speed, drainage, drying, and fiber bonding. Each variable in turn has distinct properties. For example, the pulp stock from which a paper is made has specific properties, including crystallinity, fiber bonding, viscosity, and drying, which affect the nature of the final sheet. Refining techniques such as beating affect fibrillation, swelling, hydration, and water retention. On the molecular level, cellulose, the primary and most stable component of paper fibers (fig. 1), has properties imposed by its structure (fig. 2), which creates amorphous and crystalline regions in fibrils (Smith 1991). The amorphous regions are random, flexible, and water accessible (fig. 3), while the crystalline regions are ordered, rigid, inert, and relatively impermeable to water (Kremer and Tabb 1990). Examples of chemical properties of paper (table 2) and their respective test methods (Waterhouse 1988; Wilson and Parks 1979) are listed below.
Fig. 2.
Detail of fibril, showing crystalline and amorphous regions and proposed aging mechanism (Reprinted with permission from Inaba and Sugisita 1991, after Koura and Krause 1987)
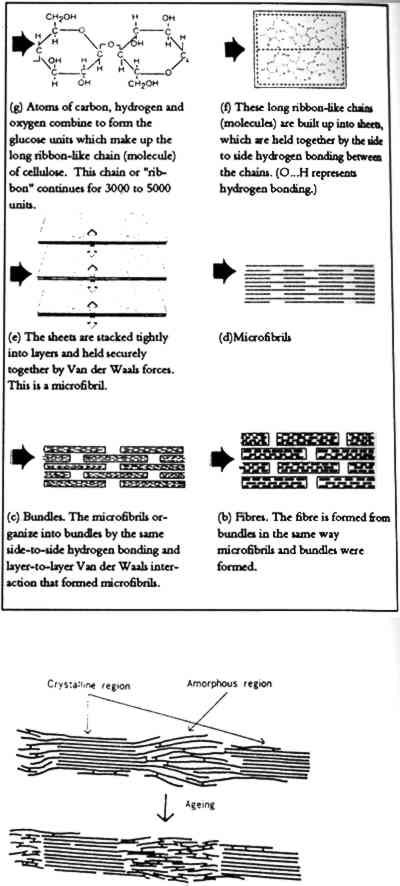 |
Fig. 3.
Detal of cellulosic microfibril amorphous region showing water adsorption (Reprinted with permission from Kremer and Tabb 1990)
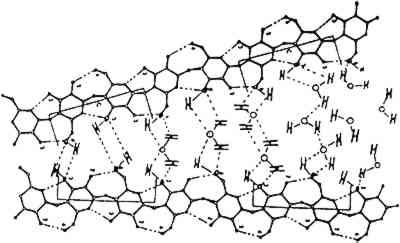 |
- Degree of polymerization (DP) of the cellulose chain, decreased by hydrolysis, oxidation, and chain scission and increased by cross-linking (table 1), can be determined by viscosity measurements or gel permeation chromatography, which also indicates molecular weight distribution.
- Acid content can be measured by alkaline reserve titration, odometric total acid, and surface and cold extraction pH (Burgess and Binnie 1990).
- Production of functional groups such as carboxyl and carbonyl groups, in particular aldehydes and ketones (table 1), through hydrolysis and oxidation, alters the molecular structure. Content can be measured by several methods including titration and iodometric techniques.
- Peroxide formation, which could occur from oxidation is difficult to measure, but one technique suggested in the conservation literature is measurement by the Russell effect (Daniels 1988).
- Moisture content and regain can be measured by gravimetric methods.
- Alkali solubility includes measurements of carbonyl content by hot–1% alkali solubility test, copper number, and the borohydride reducing method. Ester and ether content can be measured by Raman spectroscopy, nuclear magnetic resonance, and ultrasound.
It is important to note that it is the noncellulosic components in some papers that are the most reactive, as in the case of lignin-containing papers. Ironically, this very instability makes such papers the most difficult to monitor and measure chemically.
2.2 PHYSICAL PROPERTIES
Physical properties of paper sheets are determined by basis weight, thickness, density, two-sidedness, smoothness, permeability, handle, rigidity, roughness, and porosity, which in turn affect sheet properties of softness, hardness, compressibility, dimensional stability, curl, and strength (Casey 1980; Bolam 1962). Attention tends to focus on strength measurements since “strength properties can … serve as indications of the permanence of paper, even when the nature of the chemical changes responsible for the deterioration remains unknown … [and] can also be used for evaluating the effectiveness of treatments for aging” (Caulfield and Gunderson 1988). The following are some strength, or mechanical, properties and examples of respective test methods.
- Fold endurance, hampered by a wide standard deviation owing to the limited area tested, can be measured with the MIT folding endurance tester. This method produces results most consistent with the handling of books.
- Tear resistance, including edge tear resistance or internal tear resistance (similar to the kind of tearing occurring to a nicked book page), can be measured using the Elmendorf tear test.
- Burst strength, one of the most common tests in the paper industry but not much used in paper conservation, is measured by the Mullen test.
- Tensile strength is often determined by standard span tensile strength testing, which measures interfiber bonding and total network strength, and is expressed by stress-strain plots, tensile energy absorption, and Young's modulus (fig. 4). Fiber strength, internal bonding, or fiber orientation may be measured by zero-span, z-direction (through-the-thickness), and wet tensile tests.
Fig. 4.
Example of a stress-strain plot of the machine direction (MD) tensile strength of unwashed (I) and washed (II) Whatman paper (Ca(OH)2:deionized water 1:1), showing A) stress or load to breadk; B) strain, elongation, or stretch to break; C) tensile energy absorption area (TEA) or total area under the curve, representing work expended to break, and characteristic of durability (e.g., the washed paper [II] may be more durable than the unwashed paper [I] since its total area under the curve [CII] encompasses most of and exceeds [CI]); and D) Young's modulus, or initial straight line of the slope characteristic of elastic materials and indicative of stiffness. (Modified from van der Reyden et al. 1988)
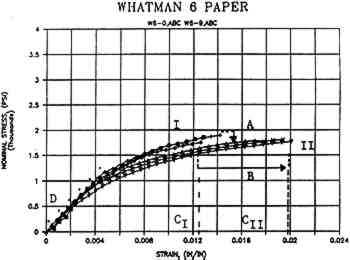 |
2.3 OPTICAL PROPERTIES
As mentioned, optical properties are physical properties which conservators often monitor as an indication of chemical changes. Optical properties of paper include color, brightness, whiteness, reflectance, gloss, opacity, transparency or transmittance of light, and fluorescence (Mark 1983). Measurements are made with reflectometers, spectrophotometers, colorimeters, and goniometers. Findings may be reported in various modes including percent reflectance (figs. 6a, 8a), CieL∗a∗b∗ (fig. 9e) and Kubelka-Munk scattering and absorption coefficients (Berndt 1989; Burgess and Binnie 1991).
Fig. 6a.
Whiteness (reflectance) of rag paper (1), chemical woodpulp paper (2) and chemical/mechanical woodpulp (3); Control sample (C), Washed sample (W).
 |
Fig. 6b.
Tensile strength expressed as breaking length: chemical woodpulp (2) and mechanical woodpulp papers (3–5); Control sample (C), Washed sample (W).
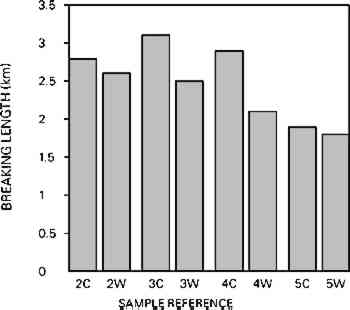 |
Fig. 6c.
Fold endurance of chemical woodpulp paper (2) and mechanical woodpulp paper (3–5); Control sample (C), Washed sample (W).
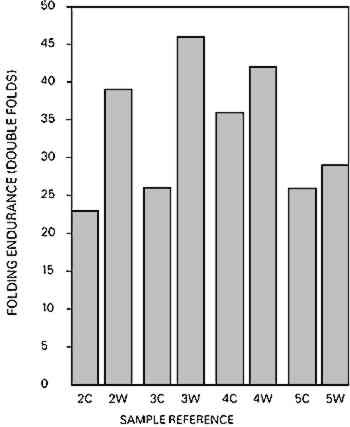 |
Fig. 8a.
Bleaching of thermally-aged filter paper
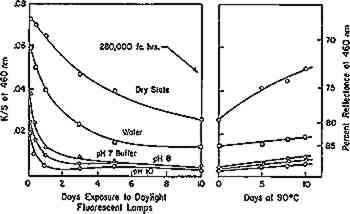 |
Fig. 8b.
Relationship between DP and pH of solution during exposure of aged filter paper to DFL for six days
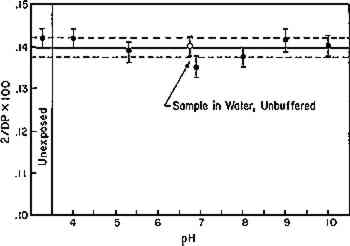 |
Fig. 9.
Effect of aqueous light bleaching on tensile properties (MD) of unsized and sized paper, before and after aging. Figs. 9a, 9b. Treated unsized paper underwent an increased in elongation as compared to unwashed control both before and after aging. Figs. 9c, 9d. Sized, naturally aged artists' paper underwent a decrease in load to break in washed samples as compared to control as a result of breakdown in sizing, and particularly in awueously light-bleached samples, which had the highest solution temperature (40�C+), which may have thermally degraded sizing even further. Key: Controls: █Untreated, dry, in dask; ▴ Washed Ca(OH)2, dry, in dark; ▵ Washed Ca(OH)2, dry in light; ○ Washed Ca(OH)2, Immersed Ca(OH)2, in dark. Experimental Sample: ○ Washed Ca(OH)2, Immersed Ca(OH)2, in light. (Schaeffer et al. n.d.)
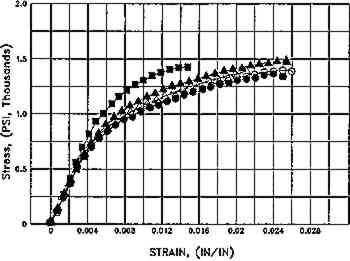 |
Fig. 9a.
Whatman 1, Unaged. 2 hours in Weather-Ometer
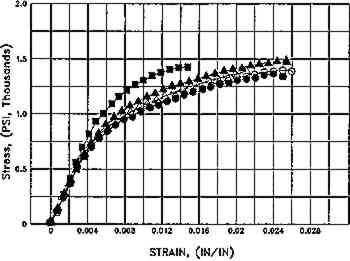 |
Fig. 9b.
Whatman 1, Aged. 2 hours in Weather-Ometer
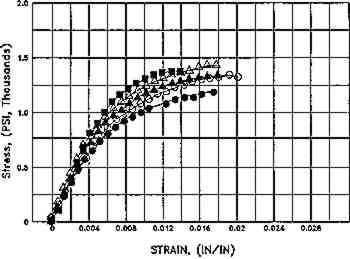 |
Fig. 9c.
Whatman 56, Unaged. 2 hours in Weather-Ometer
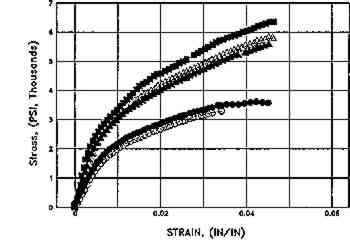 |
Fig. 9d.
Whatman 56, Aged. 2 hours in Weather-Ometer
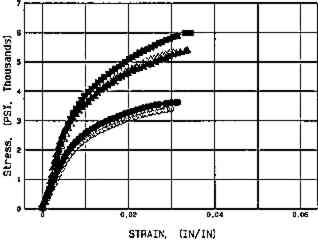 |
Fig. 9e.
Whatman 56, Unaged and Aged. 2 hours in Weather-Ometer. Effect of aqueous light bleaching on color stability of a gelatin-sized paper before and after aging: b∗ values of CieL∗a∗b∗ (increase in b∗ indicates increased yellowing)
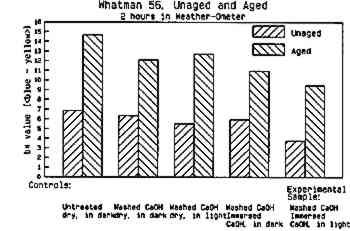 |
Paper properties are interdependent. Interpretation of test data is complicated by the fact that measurements represent “a combination of such factors as flexibility, bonding strength, and fiber strength … which are dependent on type of fibers, length and thickness of fibers, imperfections in fibers, flexibility, pattern of fiber network, number of bonds, strength of the individual bonds, the weight of the paper, the apparent density, the moisture content, and many other factors” (Casey 1980). Properties are in turn affected individually and collectively by environmental parameters such as temperature, humidity, and light; by aging; and by conservation treatments.
|