SOME OBSERVATIONS ON THE USE OF ENZYMES IN PAPER CONSERVATION
Pia C. DeSantis
ABSTRACT—A review of the conservation and enzymology literature is offered in order to characterize the use of proteolytic and starch digesting enzymes in paper conservation. Results from experiments designed to test the effects of enzymes on aged papers are presented. A protease new to the conservation field was used in these tests.
1 INTRODUCTION
CONSERVATORS HAVE LONG VALUED enzymes for their role in facilitating the breakdown of tenacious accretions of old paste and glue. This article presents a study of the use of enzymes in paper conservation. A brief discussion of terms as well as the currently accepted theories of enzymatic reaction pathways will be followed by an outline of earlier enzyme studies published by paper conservators. Facts derived from a review of the enzymology literature will then be presented, since they clarify statements in the conservation literature. The article concludes with the results of a series of experiments run on an enzyme new to the conservation field. The purpose of the author, then, is not only to call attention to the importance of the enzymology literature, but also to highlight a new enzyme which may prove useful to conservators in the future.
2 GENERAL TERMINOLOGY
ENZYMES ARE PROTEINS found in organisms and their purpose is to help those organisms use efficiently the materials they consume. As catalysts, enzymes increase the speed of reactions without being consumed themselves. The enzymes of interest to conservators catalyze degradation reactions, mostly hydrolytic reactions, and usually belong to the class known as hydrolases. The preparations which conservators have purchased in the past have been extracted from the cells of bacteria, molds and cattle pancreas.
Like all proteins, enzymes are made up of amino acids linked by peptide bonds between the amino group of one acid and the carboxyl group of the next.1 The chain comprising each enzyme has a unique sequence of amino acids as well as a unique configuration, and their uniqueness makes it possible for hydrolytic enzymes to catalyze the breakdown of only certain molecules.2 These molecules, called substrates,3 fit into the pockets created by the enzyme's twisted chain.4 The ability to enter an enzyme pocket ensures the close interaction of the reacting substrates, thereby lowering the initial energy needed for their reaction. Thus, the degradation of the substrates takes place in a far shorter time than would be necessary without the benefit of the enzyme.5
When in the organism, the enzyme depends on its environment to function properly.6 Temperature and pH shape this environment, and consequently enzyme reactivity and efficiency are temperature and pH dependent.7 Product literature will often refer to temperature and pH optima which supposedly provide the user with conditions for eliciting the enzyme's peak efficiency. However, these terms are not absolute but relative, and their applicability to our purposes will be discussed below.
All enzyme molecules have amino acids as part of their structure, but some may also include sugars, vitamins and a number of other compounds which may be necessary for the enzyme's stability or catalytic efficiency.8 Enzyme molecules which include metals are called metalloenzymes and form an important subgroup to be discussed below.
At present, two types of enzymes have been found useful in paper conservation: first, the amylases, or starch digesting enzymes, which quicken the degradation of old starch paste, and second, the proteases, or protein digesting enzymes, which speed the degradation of old hide glue. The reaction pathways have been summarized by Grattan, St. Hilaire, Burgess and McCawley:9
Fig. .
 |
Water, which must be present for these reactions to take place, acts as the cleaving agent10 and carries the water soluble end products, glucose for the amylase and amino acids for the protease, away from the enzymes' active sites.11
3 ENZYMES IN PAPER CONSERVATION
Enzymes which digest starch and protein have become widely accepted as some of the most useful reagents available to paper conservators. Delicate papers such as gampi which tolerate little manipulation respond well to enzyme treatments, since adhesive accretions may be removed with a water-thin solution applied with a soft brush.12 Similarly, for the removal of adhesives from waterleaf and other absorptive papers, application of enzymes can be a method that is at once the most efficacious and the least disruptive to the paper fibers. Extremely thick accretions which would require inadvisably long or repeated bathing times in traditional washing solutions are also more efficiently removed by enzymes, because they catalyze a degradation reaction of the components of paste and glue. In many cases, this efficiency means a safer treatment for the object.
As indicated above, enzyme preparations can be dissolved in water and applied locally or in overall treatments. Enzymes have been used in cellulosic poultices13 and in mixtures of water and organic solvents,14 although it should be noted that the latter procedure may not be advisable.15 A rinse step is an essential part of an enzyme treatment and adjustments of temperature and pH can increase an enzyme's efficiency. The importance of temperature, pH and the removal and inactivation of enzymes are discussed in the references cited below as well as in later sections of this paper.
Paper conservators have recognized the benefits of enzymes since the late nineteen sixties. In 1969, Paul Banks mentioned collagenase,16 an enzyme which attacks collagen, as a tool for the quick removal of animal glue accretions. In 1970, Otto Wendelb⊘ published a procedure using trypsin,17 another protein digesting enzyme, which enabled him to separate the pages of a water-damaged manuscript tha had been glued into a solid block by the action of its dissolved and subsequently dried sizing. In 1977, Segal, Cooper and Hatton found that Wendelb⊘'s methods weakened the paper and faded the inks of their experimental pieces.18 Thus, they devised new procedures employing a protease derived from the organism Streptomyces griseus (hereafter referred to as S.griseus) and an α amylase derived from the organism Bacillus subtilis (hereafter referred to as B.subtilis).19 Since their experiments revealed that a minimal amount of enzyme can be used if pH and temperature optima are maintained, they stressed the importance of the pH and temperature of an enzyme bath. Because of the emphasis on pH, commercial buffer solutions using sodium phosphate are an important part of their treatment procedure.
By 1980, conservation scientists were consulting the enzymology literature to find experimental methods for categorizing enzyme efficiency.20 Burgess and Charette investigated the effects of Protease Bovine as well as an α amylase derived from Aspergillus oryzae on various artist's media applied to paper.21 Other articles have described the use of enzymes in a variety of applications, but their purpose has been to circulate new treatment procedures.22
Three important areas of inquiry which require further investigation can be identified: first, the nature of the products of enzymatic reactions and whether they harm the artifact during the time of reaction; second, whether these products can be safely and effectively removed from the artifact; and third, whether any residual material from an enzyme treatment will harm the artifact over time. Determination of the products of enzymatic reactions requires equipment and reagents not readily available to most conservation laboratories. However, methods which have become traditional in conservation testing can be used to study any adverse effects sustained by an artifact during treatment as well as to investigate the second and third areas of inquiry. For example, there have been two unpublished series of fold endurance tests run on enzyme treated papers, one by Segal23 and the other by Rogers and Rayer.24 These researchers could not draw firm conclusions from their studies. Unfortunately, the work presented herein cannot be termed conclusive either, but it is hoped that some of the results of research and testing will prove useful.
4 REVIEW OF THE ENZYMOLOGY LITERATURE
WHILE THE STUDIES cited above place appropriate emphasis on the speed, specificity and apparent safety of enzyme treatments, some misapprehensions about enzymes do appear in the conservation literature.
First, the importance of pH and temperature to the efficacy of enzyme reactions is generally overemphasized. Published treatment procedures usually include the prescribed pH and temperature optima for the enzyme employed and state that the enzyme cannot function efficiently unless it is used in an environment controlled by these optima.25 For example, articles discussing the use of the S. griseus protease and the B. subtilis α amylase have always included instructions for the preparation and maintenance of solutions that meet the optima of 37�C and pH 7.5.26
However, for the enzymologist temperature and pH optima are not absolute but relative terms.27 It is important to note that while a so-called temperature optimum may increase the rate of the reaction between enzyme and substrate, it may also increase the rate of the enzyme's inactivation.28 The pH also affects several factors simultaneously because it alters the ionization state, or charge, of the enzyme and substrate.29 The factors affected by pH include the affinity of the substrate for the enzyme, the velocity of the catalyzed reaction, and the stability of the enzyme.30 However, such effects are not important if there is an excess of enzyme or substrate.31 Since an excess of substrate (old glue or starch paste for the conservator) often prompts the decision to use an enzyme, close observance of pH optima is not essential. It should be remembered that enzymes are stable and thus will remain active over a wide range of pH. For example, α amylase from B. subtilis is stable from pH 5–12; protease from S.griseus from pH 5–10.32 Similarly, although each 10�C rise in temperature can quadruple an enzyme's reaction rate, enzymes will function within a broad temperature range and can be used effectively at room temperature.33
The purpose of the above discussion is to stress that enzymes lend themselves to experimentation. There are a number of variables available to the conservator in a system of enzyme, water and starch paste or animal glue. In many cases, a satisfactory working environment for the enzyme can be produced by the pH of the moistened accretion or the pH of the deionized water used to make the enzyme solution. A slightly elevated temperature can be used to offset a pH that is far from the optimum but which the conservator is not at liberty to change.34 Finally, although the velocity of the reaction would doubtless be enhanced by strict maintenance of one or the other if not both optima, satisfactory results can be obtained even when ignoring both conditions.35
The second point to be discussed is the safety of enzyme treatments. The safety of such treatments is dependent upon a number of factors pertaining to (1) the purity of the enzyme, (2) the possibility of removing the enzyme from the paper and (3) the possibility of the irreversible inactivation of any residual enzyme.
The specifications for enzyme purity established by most purchasers will usually be far less stringent than those required by conservators. An unadulterated enzyme would be useless to the majority of a chemical company's customers. Salts, acids, preservatives and fillers such as sawdust must be added to any enzyme which is to be subjected to the industrial environment.36 An enzyme that one buys “off the shelf”, such as digestive aids or contact lens cleaners, has been similarly treated and would not be suitable for our purposes.37 The conservator should also be aware that preparations designed for enzyme research often contain stabilizers.38 Consequently, a careful reading of the product description is essential even when purchasing enzymes from companies specializing in reagent grade materials. One should choose enzymes described as “free from foreign extenders”.Preparations described as “crude” and preparations that already include buffers should be avoided. Mixtures of several enzymes sold as one reagent have been found useful in some situations.39 However, one must be certain that all the components of such mixtures are listed in the product description, since many enzymes have properties that could necessitate changes in one's treatment procedure. For example, the protease from S. griseus is stabilized by calcium against heat inactivation (a property to be discussed below).40
There is a potential danger of an impurity of cellulase, the enzyme that breaks down cellulose, in any enzyme preparation that the conservator might buy, since the microorganisms which produce protease and α amylase also produce cellulase. However, purification techniques have become so sophisticated that the realization of this potentiality is quite improbable. Indeed, methods are currently being developed whereby the DNA of the enzymesynthesizing microorganism is adjusted so that the desired enzyme is the only one produced.41 Even more reassuring is the resistance of cellulose to cellulase attack. Commercial cellulases work well on cellulose derivatives like carboxymethylcellulose, but do not work or work very slowly on native cellulose.42 A pulp of cotton linters must be fine ball milled for an hour to enable cellulase to attack it, and even then complete breakdown of the cellulose takes ten days.43 A pulp containing lignin would require even more vigorous conditions, since lignin blocks an enzyme's accessibility to cellulose.44 Finally, a sample of cellulose will resist enzymatic breakdown even after it has been hydrolyzed by dilute acids,45 so a degraded, acidic paper should not easily be attacked by a chance cellulase impurity.46
There are three other characteristics which the conservator should evaluate before purchasing an enzyme preparation. Enzymes derived from thermophilic bacteria may not be desirable in some cases, since these are unusually heat stable (a property to be discussed below).47 Such bacterial properties are found in Bergey's Manual of Determinative Bacteriology,48 a reference book that is available in any college library. Some scientists also speculate that an enzyme of low molecular weight would be more easily washed out of the paper.49 Although this proposition has yet to be proven, conservators might consider purchasing a low molecular weight reagent, which for enzymes would be about 15,000 atomic mass units,50 rather than one of a high molecular weight, which would be over 50,000 atomic mass units.51 Finally, it is important to consult an enzyme's product description for data describing the rate of the enzyme's hydrolysis reaction. This information appears under headings like “activity” and “unit definition”.If several preparations satisfy the conservation specifications discussed above, one should choose the enzyme which offers the highest number of units for a given weight of reagent (often referred to as “solid”). As a result, one should increase the possibility of removing the enzyme preparation from an artifact during a rinse step, since a more powerful reagent can be used in more minute percentages.
As implied above, the safety of an enzyme treatment not only depends upon the proper choice of enzyme preparation but also upon an effective rinsing step. The conservation literature reveals a prevalent mistrust of the adequacy of a rinse step to effect the complete removal of enzymes from an artifact. Recommendations for the inactivation of any residual reagent thus appear in published treatment procedures. Application of alcohol to areas that have been treated with enzymes and subsequently rinsed has been suggested as a method for the inactivation of any residual enzymes.52 However, if this inactivation step is to be effective, the area treated with enzymes must be flooded with alcohol. Studies made by Cooper, King and Segal led them to propose the following reaction:
Fig. .
 |
53
They found that an excess of enzyme favored enzyme activity.54 It follows that an excess of solvent would be required to favor inactivation. This hypothesis is confirmed by enzymology textbooks.55
The behavior of enzymes in organic solvents is not necessarily due to the creation of an enzyme-solvent complex. Enzymologists have explained the interaction of enzymes and solvents in terms of the ionization of a solvent-enzyme as opposed to a water-enzyme mixture. Ethanol has a lower dielectric constant than water. Since a decrease in dielectric constant increases the attractive force between two opposite charges, ethanol decreases the ionization of proteins and thus promotes their coalescence.56 An enzyme can be seen, then, as opened up and free to interact with the substrate when in water, and closed, and therefore not as accessible to the substrate, when in an organic solvent. The coalescence of such an inactivated enzyme has a practical implication for the conservator: as Segal has noted,57 a coagulated enzyme would be difficult to remove from paper. Consequently, if inactivation is deemed necessary, it must follow the rinsing step.
It is noteworthy that an enzyme inactivated by alcohol could possibly reactivate and again serve as an efficient catalyst if it were returned to a temperature and pH within its stability ranges.58 The likelihood of this possibility is diminished by using a minimal amount of enzyme and by rinsing the object thoroughly before the solvent inactivation step.59
Inactivation is also caused by denaturation, which has been defined as the unfolding of a protein's folded, native structure to an open coil.60 Inactivation by denaturation can be reversed,61 but this possibility is again unlikely if one is careful to reduce the amount of enzyme present by using the minumum required and by thoroughly rinsing the treated area before the denaturation step.62
Immersion in a water bath maintained at 50�C has been prescribed to denature an enzyme that might remain in a treated, rinsed paper,63 but this method is not universally applicable. For example, such a denaturation step would have no effect on the known α amylases (derived from B. subtilis, Bacillus stearothermophilus, Aspergillus oryzae and Aspergillus niger).64 These enzymes are metalloenzymes including calcium in their structure, and researchers have found that the bound calcium enables these enzymes to withstand temperatures up to 100�C.65 Denaturation of the α amylases at temperatures of 100�C or below would require the removal of the protective calcium with a chelating agent like EDTA.66
The protease derived from S. griseus enjoys a similar immunity to elevated temperatures.67 Although calcium is not part of the native structure of the S. griseus protease, this enzyme will take up calcium, magnesium, and a number of other divalent cations from solution and thereby be protected against inactivation by heat.68 The amounts of calcium or magnesium needed for these effects is minute, on the order of 0.0015 grams of these divalent cations per six liters of enzyme solution.69 Conservators should be alerted to this property of the S. griseus protease, since many of us have used some form of calcium or magnesium to buffer solutions of this enzyme to its optimum pH of 7.5. We have thereby unwittingly stabilized this enzyme against changes in its environment. It should be noted that a final deacidification step would also stabilize any residual enzyme.
Many enzymes are not protected by divalent cations against changes in their environment. For example, the protease derived from Aspergillus saitoi (to be presented below) would be inactivated by a bath of 55�C, and if enough calcium or magnesium ions were present to raise the pH to eight, this inactivation would be irreversible.70 It has become apparent to this author that consultation of the enzymology literature is imperative when choosing an enzyme for conservation purposes. Before ordering an enzyme, the conservator should refer to The Enzymes, edited by Paul Boyer.71 This set of fifteen volumes is available in any college library. The spines of each volume identify the categories of enzymes discussed according to the reaction they catalyze, such as “Hydolysis. Glycosides”.The index is well cross-referenced, and one can research enzymes not only by their general name (such as “α amylase”) but by one of the microorganisms which synthesizes them (such as “Bacillus subtilis”). Pertinent chapters present summaries of all the currently known facts about each enzyme's stability ranges, optima, and useful and damning peculiarities.
If not stored in cool, dry conditions, enzyme preparations suitable to conservation use72 will eventually lose their catalytic properties. Unfortunately, it is unclear just how long the enzymes remain in a condition that makes reactivation possible. According to one microbiologist, an enzyme dried on paper could retain the ability to be reactivated for several years.73
An unsophisticated experiment performed by the author suggests that enzymes reactive for longer periods than one might suspect and emphasizes the importance of sufficient rinsing and some type of inactivation step. A piece of paper that had been coated with a thick layer of gelatine and oven aged for three days at 100�C was immersed in a 0.5% solution of the protease derived from Aspergillus saitoi (hereafter referred to as A.saitoi) until the gelatine dissolved. The treated paper was given a cursory rinse with tapwater and remained uncovered for five weeks on an exposed shelf in the Paper Conservation Lab of the National Gallery of Art. The paper was then cut in half. One half received an application of warm gelatine, was folded, and left under pressure to dry. After drying, the paper came apart easily: it had not adhered. The A.saitoi protease is active in the acid pH range and at body temperatures,74 and the warm gelatine, which had a pH of four, apparently created an environment in which the enzyme could reactivate. The author is reasonably certain that this phenomenon was due to residual enzyme, since running the above-described “adhesion test” on a desized, but otherwise untreated paper produced a well-adhered sample.
The remaining half of the enzyme treated paper was cut in two. One piece received a forty-five minute soak in deionized water raised to pH eight with calcium and magnesium. These conditions are supposed to irreversibly inactivate the A.saitoi protease,75 and after being subjected to the “adhesion test”, the paper did indeed adhere to itself. The other piece received a fifteen minute soak in ethanol. This treatment apparently inactivated the enzymes as well, for the paper remained bonded after undergoing the “adhesion test”.
The author recogizes that this experiment is only a crude preliminary study. A 0.5% enzyme solution was used, and initially, the paper was rinsed only briefly. Treatments designed for works of art on paper require very different procedures. The least possible percentage of enzyme is employed and the sheet is rinsed as extensively as the nature of the object permits. Nonetheless, it is significant that in this experiment the enzyme apparently survived five weeks of exposure to dust, light and room temperature. Since unfavorable storage conditions do not easily destroy enzymes, it seems worthwhile to seek enzymes that can be irreversibly inactivated by methods that are safe for art objects.
It should be noted that some scientists present a strong case for omitting the inactivation step from enzyme treatments when the least possible percentage of enzyme is used and when the object can be rinsed extensively. Scientists who hold this view stress the importance of keeping the enzyme stable during the initial rinsing period to eliminate any danger of the enzyme coagulating while still in the paper. To this end, a rinse in any buffers used for treatment should precede rinsing in the wash-water appropriate for a final bath. In addition, solvents should not be applied to a sheet that has been treated with enzymes and has yet to be rinsed.76
The author concurs that careful rinsing must precede any attempts to inactivate an enzyme. Moreover, her research indicates that the minute percentages of enzyme used by conservators should not harm paper, even if the enzyme is not completely removed or inactivated. Nonetheless, until data for a wide variety of papers can be obtained regarding the susceptibility of enzymes to rinsing,77 it can only be advantageous to seek an enzyme which is not more resistant to removal by rinsing than other reagents and for which the inactivation procedure is actually considered beneficial to many works of art on paper. Consultation of Boyer's opus uncovered a protease that is appealing for these reasons.78
Presentation of the protease “Aspergillopeptidase A”.The “new” protease is derived from Aspergillus saitoi (mentioned above). Its properties make it preferable to the S.griseus protease that many of us have used in the past. Since the A.saitoi protease is an acid protease, it is active when the pH of its solution is below seven and is inactivated when the pH is above seven.79 Indeed, unlike the protease from S.griseus, A.saitoi protease is not stabilized by calcium, magnesium or any other divalent cation, and will be completely and irreversibly inactivated if subjected to a bath raised to pH eight using whatever alkaline solution the conservator prefers.80
For glue accretions which are acidic, even mildly acidic at pH 6, the A.saitoi protease can be used without a buffer.81 However, when not employing a buffer, it is suggested that the optimum temperature of 37�C be maintained to derive the full benefit of using the enzyme.
5 EXPERIMENTAL
Experiments were designed to test the long term effects of a strong solution of the protease derived from A.saitoi on a degraded paper.82 Samples of newsprint83 and of a buffered Rives paper84 were aged for three days at 100�C. Five specimen groups were then devised:
- untreated control
- deionized water, 37�C, one hour immersion (pH 5)
- deionized water + enzyme, 0.5%, 37�C, one hour immersion (pH 6); samples not rinsed after enzyme treatment
- same as 3, but rinsed in three twenty minute baths of deionized water buffered with calcium and magnesium (pH 7.8)
- deionized water + acetic acid, 2% (pH 2.5), one hour immersion, rinsed in three twenty minute baths of deionized water buffered with calcium and magnesium (pH 7.8).
It should be noted that these experiments are not meant to simulate conservation treatments but are intended to expose any adverse effects that an enzyme might have on an aged paper. To this end, the enzyme was not rinsed from group 3; and in group 4, specimens were rinsed in baths that elicit the enzyme's inactivation. In a conservation treatment, a rinse in deionized water would follow the use of Aspergillopeptidase A and would precede rinse baths such as those described in group 4.
Specimen group 2 serves as a wash control, since these specimens received a one hour soak in pure deionized water from the same column as that used to make up the solutions in groups 3, 4, and 5.85 Moreover, the bath water temperature was maintained at 37�C so that this bath would be comparable to the enzyme baths. The rinse baths used for specimen groups 3, 4, and 5 came from the same column,86 and the acetic acid bath was kept covered so that it would not decrease in strength. Acetic acid and enzyme treated specimens are compared in this study because some conservators still prefer to use acetic acid rather than enzymes for the removal of old glue accretions.
The temperture optimum of 37�C was maintained during the experiments by the use of hot water and metal containers. Hot tapwater was run into the lab's stainless steel sink, a smaller stainless steel container was placed within it, and this smaller container was filled with boiling water. The heated solutions were poured into enamel trays that had been placed in the smaller container and equipped with thermometers and plate glass covers. The temperature of the heated solutions was checked and the samples were agitated periodically. Additional boiling water was added to the smaller stainless steel container as necessary to maintain the temperature of the heated solutions at 37�C.
Samples were kept in blocks during treatment to facilitate handling, and cut to TAPPI specifications just prior to being placed in the enviornmental chamber.
Each specimen group comprised twenty specimens. After treatment, ten specimens were oven aged to provide post-aged data. Post-aged specimens of Rives were aged for three days at 100�C; newsprint was aged separately for four days at 100�C. (A dry oven was used since a humid aging oven was not available.) Specimens treated with acetic acid were also separated from the other four specimen groups during aging.
Brightness tests were run at 457 nanometers on the Photovolt model 670 Reflection Meter at the Preservation Office of the Library of Congress.
Fold endurance testing followed the specifications for conditioning and the procedures that are presented in TAPPI methods T402 os-70 and T511 su-69. The testing was performed in the controlled environment chamber at the National Gallery of Art on their MIT foldendurance tester model #1, set at 500 grams and sixty double folds per minute. Measurements of pH followed TAPPI method T509 os77 for cold extraction.87
6 RESULTS
Note: All specimens were aged before treatment, or “pre-aged.” After treatment, some specimens were aged once again and will be referred to as “post-aged.”
The protease derived from A.saitoi was used in all experiments.
6.1 BRIGHTNESS READINGS RIVES
Brightness test for Rives specimens which were only pre-aged do not show significant differences between the controls (columns one, two) and A.saitoi protease treated samples (columns three, four). The data in column five indicates that the acetic acid treatment slightly brightened the paper.
Comparison of the post-aged and tested Rives data in column one to that in columns two through four indicates that the specimens benefitted from all wet treatments. The greatest degree of darkening after aging is seen in the untreated control and acetic acid treated specimens.
In reference to the brightness readings for both Rives and newsprint specimens, it should be recalled that a dry oven was used, since humid conditions are reported to induce a more dramatic indication of a possible color change in a treated, aged paper.88 It should also be noted that a 1.0 degree difference in brightness was just perceptible to the author's eye. A difference of 2.0 degrees was noticeable, and over 3.0 degrees discrepancy could be considered an unacceptable difference.
6.2 BRIGHTNESS READINGS NEWSPRINT
According to the brightness tests for newsprint, the acetic acid treatment only slightly affected the pre-aged specimens, while the enzyme treatments maintained their brightness. It is interesting that the post-aged specimens which had received a buffered rinse (columns four and five) do not show as much darkening after aging as the untreated control and the deionized water wash control (columns one and two). It is encouraging that the A.saitoi protease treated specimens (columns three and four) yield the highest readings. (In reference to the data in column three, recall that the pH of the deionized water was about five, and that of the A.saitoi protease solution was about six.)
6.3 FOLD ENDURANCE DATA RIVES
Unfortunately, the standard deviations of the fold endurance date (Table I, Fig. 1) for the tests run on Rives specimens were too great to afford the data any reliability.89 Such results are said to be typical if all specimens are not taken from one roll of paper. Researchers cannot depend on the uniformity of the sheets in a ream, since a ream is cut from a stack of rolls.90 In the case of Rives, the problem is complicated further by the sizing and fillers added to this paper during manufacture.
TABLE I FOLD ENDURANCE RIVES
Fig. 1.
Fold endurance, Rives.
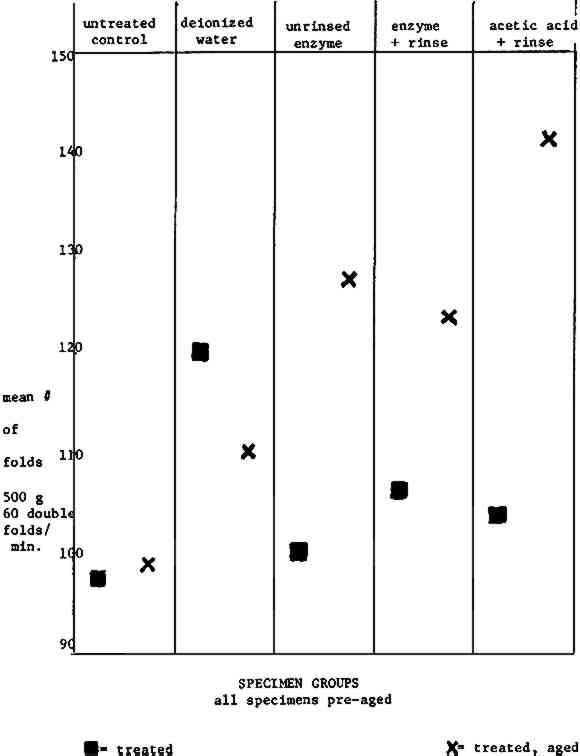 |
The data generally indicate that an aged Rives paper will benefit from a wet treatment (compare column one to columns two through five). The increase in fold endurance for all post-aged specimens except the deionized water wash control is certainly suspect.
6.4 FOLD ENDURANCE DATA NEWSPRINT
The calculated standard deviation shows that the fold endurance data (Table II, Fig. 2) for newsprint specimens are far more reliable than those for Rives specimens.91 It is encouraging that enzyme treated papers yielded higher fold endurance readings than the controls or the acetic treated specimens for both pre-aged and post-aged specimens.
Fig. 2.
Fold endurance, newsprint.
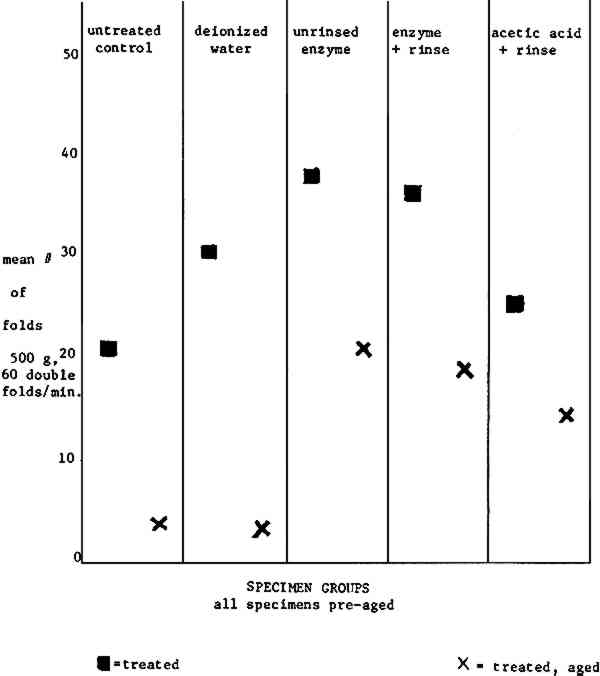 |
TABLE II FOLD ENDURANCE NEWSPRINT
It is noteworthy that the data presented here corroborate those of other studies92 in which a wash water including alkaline material apparently benefitted the papers tested (compare columns one and two to columns three through five).
6.5 PH READINGS
According to the pH results for Rives specimens (Table III, Fig. 3), the A.saitoi treatments devised for this study removed some of the paper's buffering material.93 Specimens subjected to the acetic acid treatment produced an even lower pH reading.
Fig. 3.
pH readings, Rives and newsprint.
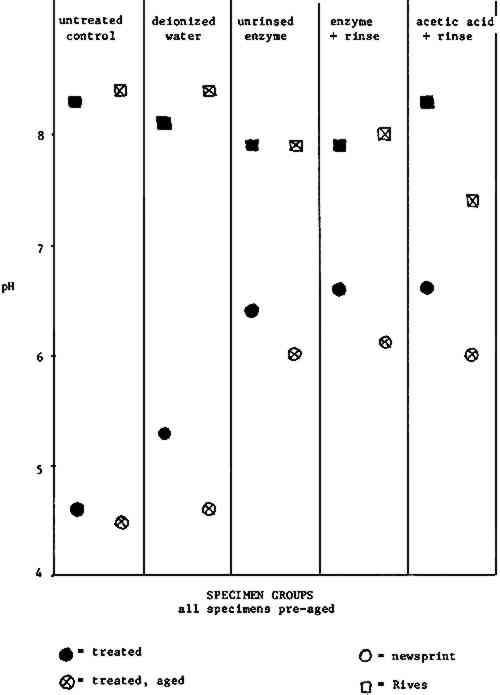 |
Table III PH READINGS NEWSPRINT
The newsprint used for these experiments does not include any buffering material, and in the light of the Rives data, it is interesting that enzyme and acetic acid treatments do not yield substantially different results for newsprint specimens (Table IV, Fig. 3). It is encouraging that newsprint specimens from the unrinsed enzyme group furnish higher pH readings than the untreated control and the deionized water wash control groups.94Table IV PH READINGS NEWSPRINT
7 SUMMARY AND CONCLUSIONS
- It is not absolutely necessary to meticulously maintain an enzyme's prescribed temperature and pH optima. The enzyme can function as an efficient catalyst even if only one of these conditions is met.95
- Before purchasing an enzyme, the conservator should consult Boyer's The Enzymes. These volumes not only provide reliable methods for the inactivation and denaturation of each enzyme, but also include the particular characteristics that would favor the use of one enzyme over another.
- Although the product literature implies that enzymes cannot withstand storage at room temperature, the author found that enzymes remaining in a paper exposed for five weeks to dust, light and room temperature could be reactivated. Clearly, effective rinsing is an important part of a treatment procedure, and after rinsing an inactivation step should be considered.
- Inactivation of an enzyme by alcohol as well as denaturation of an enzyme by heat can be reversed if the enzyme is returned to a moist environment having a pH and temperature within its stability ranges. The complete reactivation, however, of any inactivated or denaturated enzyme that might remain in a treated artifact is unlikely. Nonetheless, one should take precautions to keep the possibility of reactivation to a minimum. Such precautions include using a minimal amount of enzyme and rinsing the object thoroughly before considering a solvent inactivation step.
- The results of the tests run on specimens treated with the A.saitoi protease are encouraging. Enzyme treated specimens of newsprint and Rives fell below the controls in only a few cases, and even then by small amounts. Moreover, when compared with the differences between pre-aged and post-aged controls, the differences between pre-aged and post-aged enzyme treated specimens do not appear significant.
- The A.saitoi protease, which was used in all the tests presented in this article, is a good candidate for use in conservation. The A.saitoi protease is not stabilized by calcium, does not require buffers to work in conditions of pH 6 or lower, and is completely and irreversibly inactivated by solutions brought to pH 8 with any divalent cation the conservator might choose. The minimum time and pH required to achieve irreversible inactivation has yet to be determined, but inactivation can be obtained by a ten minute soak in ethanol as well as by a ten minute soak in deionized water raised to pH 7.8 with calcium or magnesium.96
ACKNOWLEDGEMENTS
I would like to thank Mrs. Antoinette King and the faculty and students of the Conservation Center, Institute of Fine Arts, for their advice and support in the early stages of this study, and Catherine Nicholson, Shelley Fletcher and Nancy Ash of the Paper Lab, National Gallery of Art for their invaluable instruction and ideas for the testing section and final drafts of this paper. I would also like to thank Randall L. Holton for his assistance in editing.
NOTES
1. A. L. Lehninger, Biochemistry, Worth Publishers, Inc., New York, 1972, pp. 55–56.
2. Ibid., p. 62; pp. 169ff. See also S. Blackburn, Enzyme Structure and Function, Marcel Dekker Inc., New York, 1976; C. Walsh, Enzymatic Reaction Mechanisms, W. H. Freeman and Co., San Francisco, 1979; A. Fersht, Enzyme Structure and Mechanism, W. H. Freeman and Co., San Francisco, 1977.
3. See Lehninger, op. cit.(note 1), pp. 169ff. for a good section on general terminology.
4. See Blackburn, op. cit.(note 2), pp. 497f; for excellent diagrams, see Lehninger, op. cit.(note 1), p. 176; also S. Kindel, “Enzymes: the Bioindustrial Revolution”, Technology, November/December 1981, esp. pp. 64, 66–67. I would like to thank Jerri Nelson for calling my attention to this article.
5. See Lehninger, op. cit.(note 1), pp. 169ff.; also Blackburn, op. cit.(note 2), p. 497.
6. See Walsh, op. cit.(note 2); Lehninger, op. cit.(note 1), pp. 169ff.
7. Dixon and Webb, Enzymes, third edition, Academic Press, New York, 1979, p. 11. Also see the sources cited in note 2; for a succinct approach to this enzyme characteristic, see D. Grattan, J. St. Hilaire, H. Burgess and C. McCawley, “The Characterization of Enzymes for use in Paper Conservation”, The Conservation of Library and Archive Materials and the Graphic Arts, The Institute of Paper Conservation, Cambridge, 1980.
8. Lehninger, op. cit.(note 1), pp. 169ff.; Dixon and Webb, op. cit.(note 7); Kirk-Othmer, Encyclopaedia of Chemical Technology, third edition, John Wiley and Sons, New York, 1980, v. 9.
9. Grattan et al, art. cit.(note 7).
10. M. A. Johnson, personal communication, 10/8/83.
11. Walsh, op. cit.(note 2), chapter 3; see also Lehninger, op. cit.(note 1), pp. 169ff.
12. A synthetic bristle brush should be used when employing a protein digesting enzyme.
13. M. Hatton, “Enzymes in a Viscous Medium”, The Paper Conservator, v. 2, 1977.
14. D. Cooper, C. King and J. Segal, “The Use of Enzymes in Partially non-Aqueous Media” in The Conservation of Library and Archive Materials and the Graphic Arts, Institute of Paper Conservation, Cambridge, 1980.
15. M. A. Johnson does not suggest using enzymes in solvents in paper conservation applications since the enzymes will coagulate and thus be difficult to remove from the paper. M. A. Johnson, personal comunication, 10/8/83.
16. P. Banks, “Paper Cleaning”, Restaurator, v. 1, no. 1, 1969, p. 56.
17. O. Wendelb⊘ and B. Fosse, “Protein Surgery: A Restoring Procedure Applied on Paper”, Restaurator, v. 1, no. 4, 1970.
18. J. Segal and D. Cooper, “The Use of Enzymes to Release Adhesives”, The Paper Conservator, v. 2, 1977, pp. 47–50.
19. Ibid.
20. Grattan et al, art. cit.(note 7).
21. H. Burgess and C. Charette, “Aspects of Image Safety in the Use of Enzymes in Paper Conservation” ICOM Committee for Conservation, 6th Triennial Meeting, Ottawa, 1981, 81/14/10.
22. See for example, S. Fletcher and J. Walsh, “The Treatment of Three Prints by Whistler on Fine Japanese Tissue”, Journal of the American Institute of Conservation, v. 18, #2, 1979, pp. 118–126; D. Cooper, C. King and J. Segal, “The Use of Enzymes in Partially non-Aqueous Media”, and W. van Oort and P. Poldervaart, “Prints Off the Ice: The Conservation and Restoration of the Nova Zembla Prints”, both in The Conservation of Library and Archive Materials and the Graphic Arts, Institute of Paper Conservation, Cambridge 1980; Hatton, “Enzymes in a Viscous Medium”, The Paper Conservator, v. 2, 1977; O. Wendelb⊘, “The Freeing of Papyri from Cartonnage,” Restaurator, v. 2, no. 2, pp. 41–52. There has been a comparative examination of enzyme treated papers with untreated samples using the scanning electron microscope: P. Flood and O. Wendelb⊘, “The Enzymatic Extraction of Papyri from Cartonnage: A Control Study by Light- and Scanning Electron Microscopy”, Restaurator, v. 2, 1975, pp. 53–59. The conclusions were very encouraging if not conclusive.
23. Segal and Cooper, art. cit.(note 18), “Acknowledgements”, p. 59; J. Segal, personal communication, 5/14/81.
24. Nina Rayer, personal communication, 4/83.
25. Segal and Cooper, art. cit.(note 18), pp. 47–48.
26. Grattan et al, art. cit.(note 7), pp. 7, 10. It is also noteworthy that the reaction rate of a proteolytic enzyme will begin to decrease after a certain period due to autodigestion (that is, a protease will catalyse its own breakdown since it too is a protein); see for example, Dixon and Webb, op. cit.(note 7), chapter 1. Grattan et al point out as well that the by-products of enzymatic reactions can “poison” the enzyme, and thereby slow the initial reaction rate (art. cit.[note 7], p. 10). It should be noted that the autodigestion reaction should not happen too quickly since most enzymes are resistant to proteolytic attack. Boyer, op. cit. below, (note 32); p. 257.
27. See, for example, Dixon and Webb, op. cit.(note 7), pp. 139, 165; see pp. 226–227 for a concise statement of the conditions necessary for a successful enzymatic reaction.
28. Dixon and Webb, op. cit.(note 7), pp. 165–166.
29. Dixon and Webb, op. cit.(note 7), pp. 139–140; Lehninger, op. cit.(note 1), pp. 133, 158.
30. Ibid., p. 139.
31. Ibid., p. 136. It should be noted that it is not advisable to consider the option of using an excess of enzyme when planning a conservation treatment.
32. P. Boyer, ed., The Enzymes, third edition, Academic Press, New York, 1972; B. subtilis α amylase: v. V, pp. 235ff.; S. griseus protease (a DFP sensitive alkaline protease): v. III, pp. 744ff.
33. See above, note 26.
34. In tests run by the author, it was found that the protease derived from A.saitoi, also called Aspergillopeptidase A, functioned when a temperature of 37�C and a pH of 6 was maintained, although the enzyme's pH optimum is 2.5–3.0. See Boyer, op. cit.(note 32), v. III, pp. 722ff.
35. M. A. Johnson, personal communication, 10/8/83.
36. For a detailed list of additives, see Kirk Othmer, op. cit.(note 8), pp. 173ff.
37. Ibid.; see also trade journal articles such as E. Beckthorn, M. Labbee, L. Under-holfer, “Production and Use of Microbial Enzymes for Food Processing”, Agricultural and Food Chemistry, v. 13, Jan.–Feb. 1965, pp. 30–34. I am grateful to Catherine Nicholson for calling my attention to this article.
38. See, for example, the product descriptions in the Sigma Chemical Company Catalogue.
39. van Oort and Poldervaart, art. cit.(note 22); Burgess and Charette, art. cit.(note 21).
40. Boyer, op. cit.(note 32), v. III, pp. 744ff.
41. Kindel, art. cit.(note 4).
42. Wood and McCrae, “Synergism Between Enzymes Involved in the Solubilization of Native Cellulose”, in Brown and Jurasek, ed., Hydrolysis of Cellulose: Mechanisms of Enzyme and Acid Catalysis, Advances in Chemistry Series #181, American Chemical Society, Washington, D.C., 1979. For an explanation of cellulose behavior under synergistic attack by enzymes, see Cong, Ladisch and Tsao, “Biosynthesis, Purification and Mode of Action of Cellulases of Trichoderma reesi” in the same volume.
43. Millett, Effland and Caulfield, “Influence of Fine Grinding on the Hydrolysis of Cellulosic Materials: Acid versus Enzymatic” in Brown and Jurasek, op. cit.(note 42).
44. Ibid.
45. Vitale and Simeon, ed., Industrial and Clinical Enzymology, Pergamon Press, New York 1980. Any form of celluolose which retains at least some of its native crystalline structure will prohibit enzyme accessibility.
46. Dr. M. D. Appleman, personal communication, 5/4/83, suggests some unsophisticated but effective methods that one could use as an “in-house” check of the degree of purity of an enzyme preparation. To test an amylase, one could boil egg white until coagulated, take a tiny portion and place it in an amylase solution heated to 30–37�C. If the egg white remains unaltered, the amylase should be pure enough for our purposes. For a protease, one would perform the same test, only placing a small amount of boiled starch in a solution of protease heated to 30–37�C. If the egg white or starch were affected by these treatments, it would mean that the enzyme had not been properly purified. One would then have to question whether an impurity of cellulase was present.
47. Dr. Appleman is a Professor (retired) of the Microbiology Dept., USC. Dixon and Webb, op. cit.(note 7), p. 166.
48. Breed, Murray and Smith, Bergey's Manual of Determinative Bacteriology, The Williams and Wilkins Co., Baltimore, 1957.
49. Dr. M.D. Appleman, Professor (retired), Microbiology Department, University of Southern California, personal communication, 5/83. Mike Mahinka of Customer Services, Novo Laboratories had suggested the same idea, personal communication, 9/82. A primitive series of tests were run using the protease from A.saitoi in an attempt to determine whether 10 and 30 minute baths of ethanol and alkaline water could remove the enzyme from cotton. The results were encouraging, but not conclusive, since the protein detecting agent used is sensitive enough to detect the protein in cotton. See the procedure for ninhydrin test, B. Browning, Analysis of Paper, Marcel Dekker, Inc., New York, 1969, pp. 95–96.
50. See, for example, the molecular weights listed by Boyer, op. cit(note 32), v. III, Table VIII, pp. 756–757.
51. Ibid. It is noteworthy that all the α amylases have molecular weights of 40,000 to 60,000 amu. Boyer, op. cit.(note 32), v. V, p. 250.
52. Segal and Cooper, art. cit.(note 18), pp. 46–47.
53. Cooper, King and Segal, art. cit.(note 22), p. 17.
54. Ibid.
55. Dixon and Webb, op. cit.(note 7), p. 11: “Organic solvents, for example, alcohol, inactivate most enzymes at room temperature, except at low concentrations”.
56. Lehninger, op. cit.(note 1), p. 134.
57. Segal and Cooper, art. cit.(note 18).
58. See, for example, the discussion of inactivation of specific enzymes in Boyer, op. cit.(note 32), v. III, chapter 20; v. V, pp. 235ff.; Dixon and Webb, op. cit.(note 7), chapter 1; Cooper, King, Segal, art. cit.(note 22), p. 17. It is also note-worthy that organic solvents are used to separate proteins that need to survive this separation treatment with their properties unaltered: thus, the coagulation that such solvents cause must be reversible. See for example Lehinger, op. cit.(note 1), p. 134. M. A. Johnson, personal communication, 10/8/83, states that this is true only if the solvent is very cold.
59. These precautions should minimize any danger of reactivating residual enzyme since there is evidence that the possibility of such a reactivation is a matter of the percentage of residual enzyme. See Boyer, op. cit.(note 32), p. 254: “Taka-amylase A [A. oryzae α amylase] is one of the proteins that was found to be renatured from a random coil state. The presence of an optimum concentration for the refolding of reduced Taka-amylase A was observed”.
60. Lehninger, op. cit.(note 1), p. 59.
61. Lehinger, op. cit.(note 1), pp. 59–60; Dixon and Webb, op. cit.(note 7), p. 167; Boyer, op. cit.(note 32), v. V, p. 254, with bibliography of articles presenting research that confirms the recoiling of denatured enzymes.
62. M. A. Johnson believes that using a minimal amount of enzyme and rinsing thoroughly probably renders an inactivation step unnecessary. M. A. Johnson, personal communication, 10/8/83.
63. Segal and Cooper, art. cit.(note 18).
64. See Boyer, op. cit.(note 32), v. V, pp. 238–258. This treatment was recommended for the α amylase from B. subtilis (see note 63).
64. Boyer, op. cit.(note 32), v. V, pp. 247–249 (see pp. 253–256 for the resistance of this enzyme to denaturation); see also the sources cited below, note 61. Calcium may also make the enzyme less susceptible to inactivation by alcohol, although the author has not found a direct statement confirming this supposition. However, there is the comment in Boyer (op. cit.[note 32], pp. 247–8) that the bound calcium makes the enzyme less liable to changes in its environment. An environmental change would include ionization as well as temperature.
66. Ibid.
67. A hot water bath has been recommended to denature this enzyme as well. See Segal and Cooper, art. cit.(note 18), p. 48.
68. Boyer op. cit.(note 32), v. III, pp. 744ff. Also see above, note 65.
69. To given the reader a sense of the amounts involved: α amylase has three binding sites for calcium per molecule of enzyme; for a neutral protease weighting 40,000 g-atoms, 0.0033M calcium ion was needed for stability against heat; a 0.00025M solution of EDTA removed all of the calcium from a B.subtilis∗ amylase; 15 micrograms of CaCl2 were sufficient to obtain full activity for 0.6 ml of a calcium-dependent extracellular amylase at 40�C; B.subtilis α amylase needs four or more gram-atoms of calcium/mole enzyme for complete activity. For discussions of the mechanism of these phenomena, see K. Yutani, “Role of Calcium Ion in the Thermostability of Amylase Produced from Bacillus Stearothermophilus”, pp. 91–103; W. Heinen and A. Lauwers, “Amylase Activity and Stability at High and Low Temperature Depending on Calcium and other Divalent Cations”, pp. 77–89; K. Mizusawa and F. Yoshida, “Role of a Sulfhydryl group in the Structure and Function of Alkaline Proteases from a Thermophylic Actinomycete”, pp. 61–65, all in H. Zuber, ed., Enymes and Proteins from Thermophilic Microorganisms, Birkhauser Verlag, Basel, 1976. Also see Boyer, op. cit.(note 32), v. V., pp. 247f.
70. E. Ichishima, F. Yoshida, “Conformation of Aspergillopeptidase A [A.saitoi protease] in Aqueous Solution”, Nature, v. 128 (1966) pp. 130–135; Boyer, op. cit.(note 28), v. III, pp. 722ff.
71. Boyer, op. cit.(note 32). M. A. Johnson has noted that such preparations are more resistant to inactivation than most, and believes that the conservator should thus use a minimum percentage and only in cases where the object can withstand extensive rinsing.
72. As described above, section 3, pp. 4f.
73. Dr. M. D. Appleman, personal communication, 5/4/83. Another consideration would be whether the enzyme will break down into acidic byproducts, a question which the pH testing presented here hopes to begin to investigate. Theoretically, of course, enzymes are amphoteric compounds. It is interesting to note that the protease from A. saitoi showed 5% autodigestion after 16 hours in a solution of pH 5.5 and temperature 22–25�C (Ichishma and Yoshida, art. cit.[note 70]). Consideration of this data in the light of the findings of the unsophisticated experiments discussed above, pp. 10–11, suggest the importance of moisture to enzyme breakdown, at the least, in enzyme breakdown due to autodigestion.
74. Boyer, op. cit.(note 32), v. III, pp. 723f.
75. See above, note 70.
76. The author is grateful to M. A. Johnson for these suggestions. Since the enzymes used by conservators are quite water soluble but often difficult to inactivate, he surmises that the best procedure is to use the least percentage of reagent possible and rinse well. The theories proposed for the mechanism of enzyme reactions suggest that in the case of an undenatured enzyme, the enzyme's potential bonding sites will be interacting with appropriate sites on the water molecules and the preferred substrate. In a system of proteolytic enzyme, water, glue and paper, then, paper should not compete well with glue and water for the enzyme's binding sites. (The same conditions apply in a system of starch paste and starch digesting enzyme.) It seems reasonable to assume that as long as an enzyme remains stable during treatment and rinsing and is used in small quantities, it should not tend to stay in the paper and should be easy to remove.
77. Bob Futernick has suggested the use of radioactivity tagged enzymes to study paper's retention of these reagents (personal communication, 5/1983). The author is currently pursuing examination of her samples under the Scanning Electron Microscope.
78. See above, note 70.
79. Ibid.
80. Ibid. Indeed, 10 minutes in a pH 7.8 solution of deionized water passed through a column containing calcium and magnesium inactivated this enzyme. The author lacked the reagents and equipment to determine whether the inactivation was irreversible. In at least some cases, incorrect folding of the enzyme is what causes irreversible denaturation. Boyer, op. cit.(note 32), v. V, p. 256.
81. Discovered through testing the enzyme on a Rives paper coated with gelatine that had been over aged at 100�C for three days. The pH of the enzyme bath was six. It was found that for a satisfactory reaction rate, it was necessary to maintain a temperature of 37�C. A 0.08% solution that was five weeks old (stored in the refrigerator) still attacked the aged gelatine when heated to 37�C. (Pure water heated to this temperature had no effect in the same time period.)
82. Tests were not performed on a system of paper and media, since the protease used by Burgess and Charette (art. cit.note 21) attacks a broader range of substrates and is more reactive than that derived from A.saitoi.
83. Aquabee Newsprint Rough 887-R, Bee Paper Co. Inc., Passaic, New Jersey, sold in 8″ � 11″ pads.
84. Rives “Infinity”, Lightweight, white, 115g/m2, sheets cut 19 � 26″. Unfortunately, no data as to the nature of fillers or sizing could be obtained from the United States distributors of this paper.
85. The Paper Conservation Lab of the National Gallery of Art, Washington, D.C., uses the Culligan D series deionizer column with a weak base anion resin. The water will “typically contain less than 0.5 mg/1 of dissolved minerals” (Culligan Product Literature). Before coming through the tap, the water is passed through a particulate material column. If alkalinity is desired, the water can also be diverted through a column containing marble chips and magnesium, usually in the hydroxide form. Water from the deionizer was used for all solutions in this series of experiments, and water from the alkaline column was used for all the rinse baths.
86. See note 85.
87. To safeguard the distilled water from absorbtion of carbon dioxide from the atmosphere, Barbara Miller of the Analytical Lab, National Gallery of Art, devised a method of storing the water under soda lime. A tube was equipped with open cone stoppers at either end. These stoppers were plugged with angel hair, and the tube was filled with chips of soda lime. The apparatus was fixed into the center hole of the cork used to plug the water container. It may be of interest to other researchers that a 1 gram sample of paper is not essential to meet TAPPI standard T509 os77, as long as the specified ratio of water to paper is maintained for the test. It is more important to have two readings to average than one test with the full gram of paper. I would like to thank Mr. Wilson of the National Archives and Mr. Bohanan of TAPPI for discussing this matter with me.
88. Barbara Miller, personal communication, 4/83; see also articles such as the study by Graminski, Parks and Toth in Restaurator, 2, 1978, pp. 175–178, where it is recorded that little if any degradation of their specimens occurred at 0% RH regardless of the temperature setting of the oven (p. 176). The scientists who deal with consumer questions at Customer Services of the Sigma Chemical Company, M. Mahinka of the Novo Laboratories, and Dr. M.D. Appleman, formerly of the University of Southern California, do not believe that there is any chemical reason that an enzyme should discolor or leave a colored material in paper. An article in Technology (see note 4) also speaks of enzymes as “colorless compounds”.Apparently, if an enzyme is pure enough, it should not discolor. In a personal communication with Victoria Blyth Hill of the Los Angeles County Museum of Art, the author was made aware of tests ran by Leslie Kruth while working at the museum in 1983. She found that Whatman papers yellowed with oven aging if they were dipped into solutions of trypsin and α amylase and dried without rinsing. Rinsed samples did not yellow. Trypsin is not the purest of preparations, however, and this might explain at least one of the results obtained.
89. The mean and standard deviation were calculated on Hewitt Packard HP45 calculator, which uses the formula
Fig. .
 |
for mean and
Fig. .
 |
for standard deviation. I would like to thank Barbara Miller for explaining these formulas and their practical application.
90. Dr. McComb and Dr. Tang, Preservation Office, Library of Congress, personal communication, 4/83.
91. Indeed, using the “student t” test for probability, the only comparisons which fell below eighty repeatable experiments out or 100 for preaged specimens are comparisons between specimen groups 3 and 4, and 1 and 5. For post-aged specimens, the “student t” test indicates that the results are quite reliable, the lowest repeatability being for a comparison between 4 and 5, where repeatability is 90 out of 100. Formula:
Fig. .
 |
where s = standard deviation, X� = mean, μo is fold endurance for control specimens and n is the number of measurements. I am grateful to Barbara Miller for her explanation of these formulas and their practical application.
92. See, for example, J. Nelson, A. King, N. Indictor, D. Cabelli, “Effects of Wash Water Quality on the Physical Properties of Three Papers”, Journal of the American Institute of Conservation, v. 21, no. 2, Spring 1982; Wilson, Golding, McClaren and Gear, “The Effect of Magnesium bicarbonte Solutions on Various Papers” pp. 87ff., in J. Williams, ed. Preservation of Paper and Textiles of Historic and Artistic Value II, Advances in Chemistry Series #193, American Chemical Society, Wash., D.C., 1981; L. Tang and N. Jones, “The Effects of Wash Water Quality on the Aging Characteristics of Paper”, Journal of the American Institute of Conservation, 1979, 18(2), 61–81.
93. This result could be due to the hour long soak in warm water followed by another hour long immersion, and not due to any effect of the enzyme per se.
94. This result could be due to the difference in pH: deizonized water, 5; enzyme solution, 6. It is interesting that the paper retained the pH of the enzyme solution.
95. M. A. Johnson has stated that both conditions could be moderately non-optimal and one could still achieve good results (personal communication, 10/8/83).
96. A ten minute bath in deionized water raised to pH 7.8 with calcium and magnesium inactivated the enzyme in primitive tests devised by the author (as described above). To determine whether or not the enzyme was irreversibly inactivated would require reagents and equipment not available to her at this time.
Section Index |