TECHNICAL EXAMINATION OF THE CLASSICAL BRONZE HORSE FROM THE METROPOLITAN MUSEUM OF ART
Kate C. Lefferts, Lawrence J. Majewski, Edward V. Sayre, Pieter Meyers, R.M. Organ , C.S. Smith , Edward V. Sayre , Robert H. Brill , I. Lynus Barnes , Thomas J. Murphy , & Frederick R. Matson
REFERENCES
Noble, J.V. ‘The Forgery of Our Greek Bronze Horse,’ The Metropolitan Museum of Art Bulletin, February 1968, 253–356.
Bl�mel, C., ‘Das Bronzepferd in New York ist keine F�lschung,’ Die Weltkunst, Heft12 (1968), 568.
Bearzi, B., Private communication during examination of horse and ‘II Bronzo Nella Antichita,’ La fonderia italiana, XV, 2 (1966), 1.
Rolley, C., ‘Fouilles de Delphes V : Monuments Figures, Les Statuettes de Bronze,’ Editions de Boccard, Paris (1969), 169-ff, pls. LI & LII.
Noble, op. cit., p. 256.
Ibid., p. 256.
Ibid., p. 255.
Lewin, S.Z., ‘A New Approach to Establishing the Authenticity of Patinas on Copper-base Artifacts,’ Application of Science in Examination of Works of Art [Ed.W.J.Young), Museum of Fine Arts, Boston (1970), 62–66.
Werner, A.E.,Letter to K.C. Lefferts 2/2/68.
Meyers, P., and VanZelst, L., verbal report.
Caley, E.R., Analysis of Ancient Metals, MacMillan Co.New York (1964), 116–117.
‘Chemical Composition of Greek and Roman Statuary Bronzes,’ Art and Technology (Ed.A.Steinberg), M.I.T., Cambridge1970, 37–49.
Fields, P.R.et al., ‘Copper Artifacts: Correlation with Source Types of Copper Ores,’ Science1152 No. 3728 (1966), 1504–1506.
Cesareo, R.et al., Non-Destructive Analysis of Ancient Bronzes,’ Studies in Conservation, 18 (1973), 64–80.
Craddock, P.T., ‘The Composition of Copper Alloys Used by the Greek, Etruscan and Roman Civilizations:’
‘1. The Greeks before the archaic period,’ Journal of Archaeological Science, 3 (1976), 93–113; 2. The Archaic, Classical and Hellenistic Greeks, Journal of Archaeological Science, 4 (1977), 103–123; 3.Origins and Early Use of Brass, Journal of Archaeological Science, 5 (1978), 1–16.
Zimmerman, D.W., Yuhas, M.P., Meyers, P., ‘Thermoluminescence Authenticity Measurements on Core Material from the Bronze Horse of the New York Metropolitan Museum of Art,’ Archaeometry, 16 (1974), 19–30.
Zimmerman, D.W., unpublished communication to P. Meyers.
APPENDIX
1 APPENDIX I
1.1 Examination of Mineral Incrustations and Two Metal Sections of the Metropolitan Museum of Art Bronze HorseR.M. Organ Conservation Analytical Laboratory, Smithsonian Institution, Analysis of Metal and Corrosion (CAL 0579)The various samples have now been examined in detail. They comprise:
- fragments of mineral incrustation received in January 1968;
- a core from the right flank drilled out of proper right hind leg. Most of the mineral had cracked off during the process of separation;
- a second core drilled at a later date completely through the wall of the belly.
Results:
- Sample No. 1, examined under a stereo-microscope (30X), was observed to contain deep-purple material surrounded by clear green material. At a higher magnification (100X) this same material appeared to be a black or deep-green in color and surrounded by colorless material. X-ray diffraction analysis (XD 8–17) revealed the presence of bornite (Cu5FeS4)∗, which is described as having in the massive form a purple-blue tarnish, and calcite, (CaCO3), which is usually white. These several observations appear to support each other.
- Sample No. 2 was mounted in a polyester mount. At (30X) it reveals, unetched, a dendritic structure containing interdendritic cavities and globules of lead. None of the lost surface mineral could be observed, but the presence of pits indicates that it actually penetrated into the metal structure to a depth of perhaps 0.3 mm.Examination at a higher magnification (146X) clearly reveals penetration by mineralization and actual replacement of one metallic phase by mineral. There can be no doubt therefore that corrosion processes have taken place on the metal itself and mineral has not merely become attached to the surface.Finer polishing of this same surface, using a mechanical method followed by ultrasonic removal of detritus in distilled water, revealed that the inter-dendritic cavities were free from corrosion products. They presumably resulted from shrinkage of the metal during rapid cooling.The scale of the dendritic structure (arms of dendrites separated by 0.04 to 0.08 mm) is not very much coarser than that of a modern 10% tin-bronze that has been chill-cast (0.02 to 0.03 mm). In view of the known presence of a clay core in the casting and the probability that the outer mould was also made of clay, this suggests that the walls of the outer mould were sufficiently thin to permit rapid loss of heat. Alternatively, use of a metal mould could have produced a similar result.The same cross-section under crossed polars (110X) confirms the presence of minute globules of lead (about 0.5% was reported in the analysis). Their small size is further confirmation that the metal chilled quickly.
- Sample No. 3 is about 10 mm long. Its two ends carry the mineralization present on outer and inner surfaces of the casting. It cracked into two pieces during extraction and was reassembled in methacrylate mount in order to make the cross-section along the axis of the core.
∗Bornite is a mineral found widely distributed in ore deposits. Daubr�e reported its occurrence on Roman coins and medals found in French mineral springs (R.J. Gettens, Corrosion Products of Metal Antiquities, Smithsonian Institution, 1964, p. 555). In this instance the iron present in the mineral was presumably acquired from the mineral water that surrounded the copper coins. In the object under present consideration the metal is reported to contain only 0.06% iron, scarcely adequate to supply the 11% of iron present in Cu5FeS4 if this mineral did develop in situ as a result of corrosion processes. It may be concluded that either the object corroded in the presence of ground-waters contained iron—and the presence of calcite supports this belief—or it was corroded deliberately in water enriched in iron and calcium carbonate, or the mineral has been applied externally. Examination of other samples may throw light on these various possibilities.
This section reveals a negligibly thin corrosion crust on the end representing the outer surface. 325X reveals that the entire crust has a thickness of only 0.05 mm in the area examined. Here the crust contains isolated thin patches of grey mineral in contact with the metal. This mineral was at first believed to consist of cuprous chloride, and it contains two rounded particles of what appears to be pure copper. Beyond this are patches of cuprite, orange when viewed between crossed polars and no more than 0.02 mm thick. Beyond this again lies a rather particulate mixture of what could be azurite and tin oxide. The surface of this layer is bounded by a line of isolated unidentified small black crystals.
This outer mineral crust has few of the characteristics of a protracted natural development. A layer of cuprous chloride can indeed be expected in the position found and pure copper can appear within it as a result of a corrosion process, but in the size observed it could also be generated on modern material within a period of only a few months. Actual experiment, directed to confirming the presence of cuprous chloride by exposure of the cross-section to 100% relative humidity for five days, failed either to confirm it or to initiate any observable corrosive activity whatever. Cuprite also should be present in the observed location but in much greater thickness. Furthermore, orange cuprite like the present example can be formed from cuprous chloride during only a few hours of exposure to an alkaline solution. The particulate outer layer is also unnatural, and its surface has clearly been brushed over with a dressing of black particles.
The end representing the inner surfaces is little better mineralized, although it does possess a number of pits that should contain mineral if corrosion processes had taken place.
The filling in these pits is actually a visually black material which appears grey when examined at high magnification. Its nature has not been determined but it does contain isolated siliceous particles.
Over the length of the edge of the section examined (about 5 mm) only about 0.25 mm retains residues of identifiable mineral (325X). A layer of cuprite closely adjacent to the metal is only about .006 mm thick. It is rather particulate and associated with black material that could be cupric oxide. Both of these oxides form on cooling metal in the presence of oxygen. Sufficient oxygen could be present in a porous core to yield this result. Some of the black particles are displaced into an adjacent mass of green-colored material which might conceivably result from a corrosion process. Its maximum depth is about 0.1 mm.
The pits present on this surface probably do not result from corrosion processes since they do not contain the normal minerals and cannot be activated by high relative humidity. They cannot be used as evidence of degree of corrosion.
2 CONCLUSIONS
- The object has a thin patina containing bornite in at least one location. This mineral could develop on a bronze during prolonged immersion in ferruginous waters but might have been applied as a cosmetic treatment. In favor of natural growth is the presence of calcite and the fact that the sample as taken (XD 8–1) contained larger crystals than the same fragment after gentle grinding between glass plates (XD 8–17). This was deduced from comparison of the two X-ray powder patterns. It would be anticipated that pigment prepared for application would have been better ground than this.
- On what appeared to be a well-mineralized area of the bronze, little penetration by mineralization has actually occurred and the hairline cracks that commonly accompany mineralization are absent from both samples examined. No corrosive inclusions could be found in the two mineral crusts on one of the samples.
- The cast metal chilled unexpectedly rapidly.
- There has been some deliberate cosmetic treatment of the surface. This is not uncommon among “collection bronzes”, but in this case little underlying pitting is present. Had pitting resulted from natural corrosion processes it should have remained untouched during cosmetic treatment unless the surface had been drastically smoothed first.
- Prolonged immersion in ferruginous sulphur-bearing waters should have stimulated extensive development of sulphide minerals, but none were found in these examinations.
It may be concluded that the results of this particular examination do little to favor a belief in the antiquity of the samples. Nevertheless, on occasion, objects that carry very little mineralization have been regarded by archaeologists as genuine and many thousands of years old.
APPENDIX
1 APPENDIX II
1.1 Metallographic examination of two samples from the Metropolitan Museum of ArtC.S. Smith Massachusetts Institute of Technology, Dept. of Humanities.Results of samples submitted on 13 December 1968.
- A small magnetic lump near the center of the core of the horse.
- A square piece about 6 � 7 � 2 mm which had been inserted as a plug in a squarish cavity chiseled into the surface of the rear left leg, evidently to repair a casting defect.
2 THE ARMATURE
Sample No. 1 is magnetic and had a rather well defined shape suggesting that it might be metallic, but when fractured it was seen to consist of a compact conglomerate of sand particles and a dark mineral. The present surfaces are, probably, mainly a result of the mechancial operations involved in extracting the sample and do not represent its form in situ. Figure 1 shows the microstructure of the sample after sectioning, grinding, and polishing. It consists of quartz particles (sand) with iron corrosion products completely filling the interstices and cementing the whole into a hard lump. The formation of an inner layer of magnetic corrosion product on ancient iron is quite common, though the identification of it as true magnetite Fe3O4 or as the magnetic form of hematite has not been made. It would eventually turn to ordinary rust on further exposure to a moist atmospere. The sample is not a piece of metal but adjacent sandy core material into which the expanding corrosion products of iron have grown, which tells nothing about the shape or composition of the armature itself beyond the fact that it is mostly iron.
Fig. 1.
Polished section through magnetic core material. Reflected light. � 10. (The porous structure surrounding the sample is that of the bakelite mounting material.)
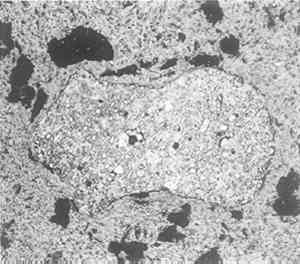 |
Early radiographs seemed to indicate that the armature was not a rod but rather a strip bent into a circular or ‘Z’ section. The uncertainty in its profile is now explained by the extensive corrosion. Nothing in the radiographs suggests any technique beyond the capacities of a Greek founder. It would be necessary to compare the radiographs with those of other bronzes of similar shape and style before any conclusions could be drawn regarding authenticity.
3 THE BRONZE PLUG
Sample No. 2 varied in thickness from 1.8 to 2.4 mm, in width from 6 to 6.2 mm, and in length from 6.7 to 7.5 mm. Two of the edges had been damaged by the drill used to free the plug from the body; the other edges had a rough array of irregular deep scratches of the kind usually associated with ancient filing. (See, for example, the filed bronze wire in H. Hoffmann, Early Cretan Armorers [Cambridge, Mass., 1972, plate 55, Fig. 2]). The flat surface is rough and oxidized, and is probably the original surface of a thin plate cast in a mold without any further mechanical treatment. The other side (the outer surface when in place) was presumably finished in the same way as the bronze surface of the rest of the horse.
Fig. 2.
Cross section of bronze plug cut parallel to the upper drilled edge. Etched. Magnification � 10.
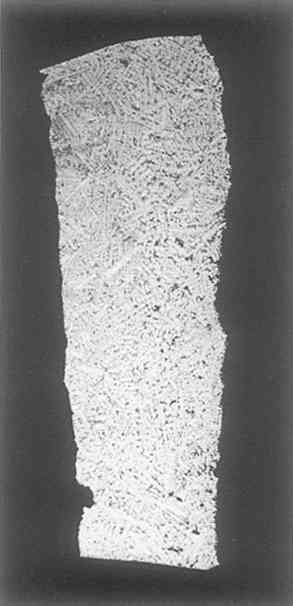 |
The longer edge that had been damaged by drill holes was ground and polished for microstructural examination. Figure 2 shows the microstructure at a magnification of 10 after polishing and etching with potassium dichromate reagent followed by 1% ferric chloride. The dendritic structure is unmistakably that of bronze in the cast condition, though the grains are fairly small. Slight distortion is visible at the left end.
There is a perceptible difference in grain size between the upper and lower parts. The top corresponds to the rough surface; the bottom represents metal solidified more quickly because it was near a cool mold surface. Both top and bottom of the piece show evidence of slight local cold work such as a chiseling operation, and the fitted edges are more heavily deformed (especially the one to the left of Fig. 2); but there is no evidence of intentional gross deformation of the piece, nor any that it had been given heat treatment after casting.
The microstructure at higher magnification is shown in Figs. 3, 4. It has a perfectly normal structure for a lead-free cast bronze with occasional shrinkage cavities and with the usual inter-dendritic areas, which at high magnification (Fig. 4) are seen to be the typical duplex structure of the alpha/delta eutectoid with some slate grey inclusions, either oxide or, more probably, sulphide.
Fig. 3.
Same as Fig. 2 at higher magnification. � 200.
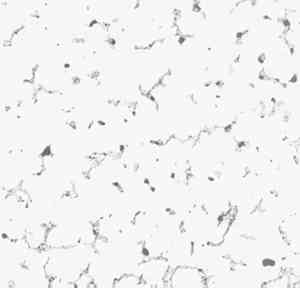 |
Fig. 4.
The eutectoid at higher magnification. Etched with potassium dichromate. � 1000.
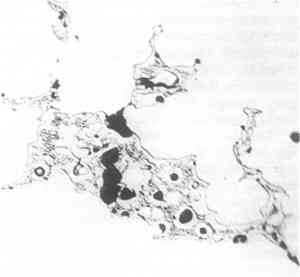 |
There are some special features to be noted, however. First, the left side of the sample shows unmistakable evidence of slight deformation, visible at low magnifications as curvature in the dendrites and at high magnification as slip lines in certain crystals (see Fig. 3). The deformation appears mainly at the edges, and probably arose during the fitting of the plug in place. Some may have occurred during removal for examination, but certainly not all, for there are places near the surface where corrosion has followed the slip lines (see Fig. 5, a cross section including at the top what had been the original outer surface of the horse).
Fig. 5.
Microstructure of unetched polished section showing internal corrosion near outer surface of plug. � 500.
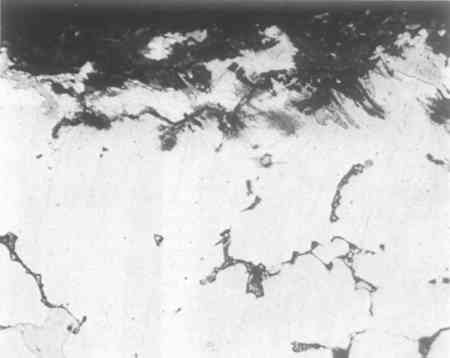 |
Figure 5 was photographed unetched in order to show the corrosion products better. It should be compared with Figure 6, a photograph of the other side of the section, including the original inner surface of the plug. The surface is of smaller grain size, and is considerably smoother than the other, and, more important, is less corroded. The metal near the original outer surface (see Fig. 5) shows corrosion along the grain boundaries to a depth of at most about 0.25 mm but usually not exceeding 0.08 mm. The irregular slip-band corrosion noted above was invariably less than this.
Fig. 6.
Microstructure of polished section showing metal near inner surface of plug. Unetched. � 500.
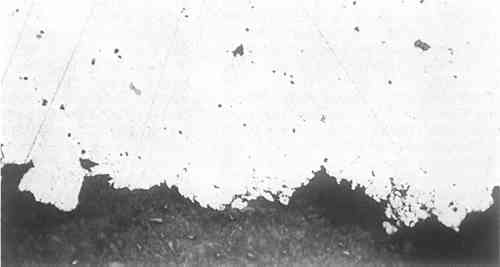 |
Most ancient bronzes that I have examined have been from the Near East and show intergranular corrosion to a greater extent than this. I would have expected the corrosion to penetrate around the side of the piece to the back side. However, the corrosion that is observed would have certainly taken a very long time to occur, and if the iron armature had been in contact with the bronze and anywhere near the locality of the sample, it would have provided good electrolytic protection for as long as metallic continuity was maintained.
It seems very unlikely that a modern repairer would chisel out a rectangular hole halfway through the piece and insert a filed cast piece of the kind studied above.
In conclusion, there is nothing in any of this that is inconsistent with ancient origin, and the general character of the patches rather strongly suggests ancient repair.
APPENDIX
1 APPENDIX III
1.1 Determinations of the Composition and Nature of the Metal and the Core Material of the Bronze HorseEdward V. Sayre Senior Chemist, Brookhaven National Laboratory
1.1.1 Emission Spectographic Analysis of Metal Specimens from the Horse.
Small specimens were taken by drilling from four separate locations. Three samples were considered to be specimens of the original metal out of which the horse had been cast, as they were taken from sites that appear to be continuous with the body of the horse (1, the rear underbelly; 2, the lower right foreleg, drilled at the bottom of the broken end; and 3, the upper part of the left rear leg, drilled into the metal exposed upon removal of a plug from the region of a cast repair). A specimen of the metal of the repair cast on to the lower portion of the left rear leg, which was obtained by drilling up into the repair from its bottom edge, was also analyzed.
Five-milligram specimens of these turnings were placed into graphite cup electrodes which were arced to complete burning in the stand of a three-meter grating spectrograph. Similar specimens of seven separate standard bronze and brass alloys were similarly arced for calibration. The optical densities of selected lines of the elements determined (two for each element) were measured by means of a photoelectric densitometer. From the measured line optical densities of the standard specimens, concentration calibration curves were plotted from which the concentrations in the object specimens were determined. The error of determination of an individual specimen was estimated to be about 20% of the reported value.
It was recognized that the three small five-milligram specimens taken from the original metal of the horse would not individually be fully representative of the composition of the metal. Collectively, however, they should provide a reasonable estimate of the overall metal composition. The results of the analysis of these specimens are given in Table 1 in the general text.
A comparison between the average basic composition of the bronze of the horse, the average of a number of published analyses of Greek bronzes of the Classical period and the analysis of the Manship bronze are given in Table 3 in the general text. The degree of agreement between alloy compositions would allow one to conclude that the bronze of the Metropolitan horse is consistent in composition with that used in pre-Hellenistic Greece.
Sixty-one analyses of early bronze objects are summarized in Table 3 in the general text. Since this summary was made, an additional 700–800 analyses of such bronzes have been reported; however, Table 3 may still be considered to be reasonably representative of such analyses.
The repair cast on to the lower end of the left rear leg has a significantly different composition, one with nearly 19% lead. The analysis of it is given in Table 2 of the general text. In a Western context, bronze with this high a lead content was not prevalently produced until the Hellenistic period. From that time on, however, high concentrations of lead have frequently characterized bronzes as they often do today. To the extent that one might accept the original atribution of the horse to the Classical Greek period one could argue that the repair was added at least a century or so after its original casting. However, the possibility of the repair being even a recent addition cannot be ruled out on the basis of composition.
1.1.2 Structural Studies of Material from the Core
Some small lumps, a few cubic millimeters in volume, of a nearly black composite of particles well cemented together had been removed by probing from the core of the bronze horse in a region that gamma-ray radiographs of the horse show to have contained a supporting metal structure. X-ray diffraction patterns of this black material have shown it to be rich in the magnetic oxide of iron, Fe3O4. It is presumed that the iron oxide is a corrosion product of a wrought iron band, but it was initially questioned whether the iron-oxide-rich composite represented the immediate residue of the oxidized iron band or was ceramic core materical near the band into which iron had migrated during the corrosion process. If the material represented an immediate residue of the supporting iron and if this had been a crude wrought iron, as would have been characteristic of fifth century B.C. iron, then one might have expected to find slag streamer residues within it. Such streamers would most likely appear as irregularly shaped filaments of a complex alumino-silicate glass containing a variety of metallic elements as impurities. On the other hand, if the specimens were of the ceramic core material into which the iron had migrated, they would likely contain a variety of particles similar to those observed in tempered pottery.
Two specimens of the sample were mounted in an epoxy resin, ground and polished for microscopic examination at Brookhaven National Laboratory. Photomicrographs, an electron scattering micrograph and a series of electron beam microprobe x-ray micrographs were taken of one of these specimens at the United Kingdom Atomic Energy Research Establishment at Harwell, England. Both specimens received careful microscopic examination by experienced metallurgists at Brookhaven and Harwell. In general, none of the metallurgists observed structures in the mounted specimen thought to be truly characteristic of slag streamers. Some filamentary-like structures did appear, however, and a region including one such structure was chosen for the electron beam microprobe study. The results of this study are shown in Figure 1.
Fig. 1.
Electron beam microprobe study of the ironrich support region within the core of the Bronze Horse (M.M.A. 23.69)
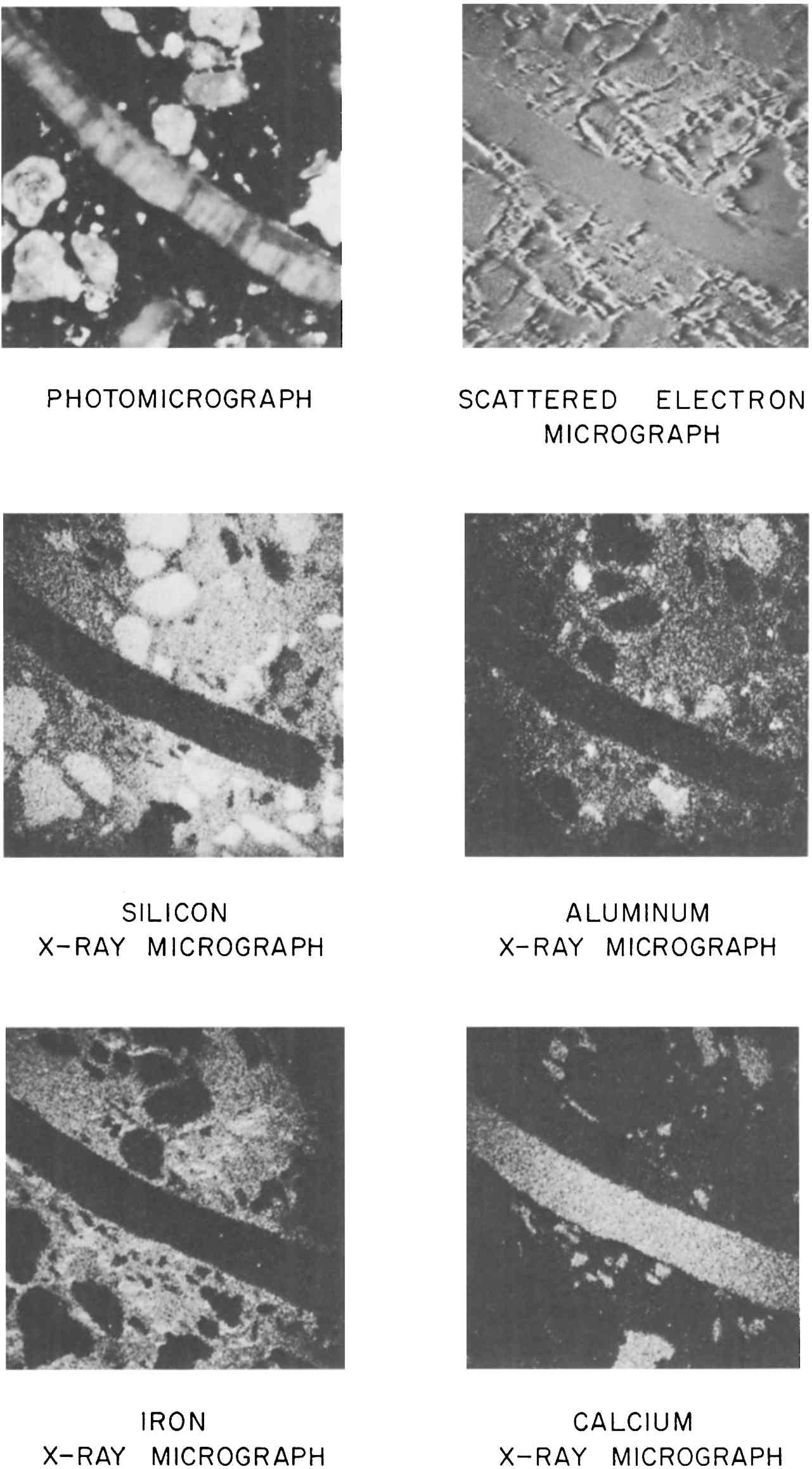 |
The photomicrograph shown in this figure was taken with polarized illumination and a polarizing analyzer in the microscope oriented at an extinction position relative to the incident light. Under these conditions one tends to see below the surface of a particle into its inner stucture. It is apparent that the long filamentary inclusion which nearly crosses the micrograph has quite a complex striated structure. The electron scattering micrograph, which was taken by the electron microprobe, shows only the surface structure of the region investigated. It tends to resemble a photomicrograph taken with bright field illumination without polarization.
The elemental x-ray micrographs are taken by the microprobe by scanning the electron beam in a raster over the area being studied. X-rays generated as the electron beam strikes the specimen surface are analyzed by the instrument, which is capable of limiting its response to individual x-rays arising from individual chemical elements. The intensities of these individually characteristic x-rays are correlated with the position of the electron beam as they are generated during the scan and are registered in a coordinated scan upon an oscilloscope screen, rather like a television picture. The resultant pictures show the distribution of individual chemical elements throughout the surface of the region scanned. The occasional fading of general intensity at the edges of some of the x-ray micrographs is an artifact of the instrument response and should not be considered as an actual reduction of concentrations at the sides of the fields.
The silicon x-ray micrograph shows several of the embedded particles to have a high silicon content. Also the fine-grained material in which the particles are embedded is rich in silicon. Notably, however, the large filamentary inclusion contains little or no silicon. The aluminum x-ray micrograph shows that some of the silicon containing inclusions also contain significant concentrations of aluminum while others do not. The fine-grained embedding matrix contains a significant concentration of aluminum but the rod-like inclusion is relatively free of aluminum. Iron shows up strongly throughout the fine embedding material, but with an uneven distribution. None of the inclusions are particularly rich in iron. The calcium x-ray micrograph reveals that the large rod-like inclusion and a few other particles contain considerable calcium. All of these calcareous particles are relatively free of the other elements measured. Additional x-ray micrograph scans for manganese and phosphorous, not shown in the figure, indicated that only small amounts of these elements were present with no pronounced regions of increased concentration.
The evidence of these micrographs is consistent with the conclusion that these specimens are of the iron-impregnated ceramic core and that this core is similar in composition to sandy earth which included some calcium carbonate shell and possibly marble fragments. The core might have been made of a clay to which a sea sand containing shell fragments had been added.
The fine-grained embedding material contained sizeable concentrations of both silicon and aluminum with only a small amount of calcium. Such relative proportion of components would be typical of the composition of a clay. The particles in which only silicon was observed are in all probability silica grains. Those containing both silica and alumina would likely be alumino-silicate rock minerals such as feldspars. One would normally expect both such minerals to be present in a sand. The particles which show only calcium would very likely be calcium carbonate, which is also likely to be in a sand, particularly a sea sand, in the form of bits of marble or shell. X-ray diffraction has confirmed the presence of quartz and calcite in the core material. The fact that the iron is concentrated predominantly in the fine matrix material only would be consistent with it having entered the structure in solution. We have found that there is a considerable amount of chloride present in this core. An initial corrosion product of the iron would likely have been water soluble iron chloride which could easily have migrated into the surrounding core.
1.1.3 Chemical Analysis of the Core Material
Samples of the general core material were analyzed for their elemental contents by the methods of neutron activation analysis and emission spectroscopy. Additional special determinations of the sodium and chlorine contents were made by atomic absorption and x-ray fluorescence. Chlorine was found to be present to the amount of 1.37 percent, a concentration commensurate with the small concentrations of sodium, potassium and magnesium found. This significant chlorine content could indicate the inclusion of clay or sand of a marine origin or the penetration of sea water into the core.
The compositional analyses are presented in the accompanying table. Most of the trace impurities occur at concentration levels of about one-third of the values normally encountered in Eastern Mediterranean clays and pottery. However, the relative pattern of these trace impurity concentrations roughly conforms to that of the clays and pottery. The calcium oxide concentration and the estimated (by difference) silica concentration are much higher than we normally encounter in pottery. It would seem most likely, therefore, that the core material was composed of a clay or soil to which a relatively high concentration of a calcareous sand had been added.
ANALYSIS OF CERAMIC CORE MATERIAL FROM WITHIN BRONZE HORSE (M.M.A. 23.69)
APPENDIX
1 APPENDIX IV
1.1 Lead-Isotope Studies of the Bronze Horse from the Metropolitan Museum of ArtRobert H. Brill∗∗Research Scientist, The Corning Museum of Glass, Corning, NY 14830 I. Lynus Barnes††Dr. Lynus Barnes, Research Chemist, Mr. Thomas J. Murphy, Research Chemist, National Measurement Laboratory Center for Analytical Chemistry National Bureau of Standards, Washington, D. C. 20234 Thomas J. Murphy†
2 INTRODUCTION AND BACKGROUND
Of four metallic samples of the bronze horse submitted to the authors for lead isotopic analysis, two (nos. 664, 1201) came from the original casting. Previous spectrographic analyses indicated that these samples contained about 0.5% lead. The other two samples (nos. 673, 1202) came from the cast-on repair of the lower section of the left hind leg and contained about 20% lead. The objective of isotopic analyses was to determine from what mining regions the lead in these two alloys might have come. The hope was that such information would be of value in deciding upon the provenance of the object.
The determination of lead-isotope ratios has become a routine method for studying the lead in early artifacts with a view towards establishing what ancient mining regions could have provided the ore from which the leads were smelted (Brill & Wampler, 1962; Brill 1970). In general this consists of matching isotope ratios from objects with ratios from galena ores from different mining regions. The method is applicable not only to metallic leads, but also to lead extracted from a wide variety of ancient leadcontaining materials, including Greek and Roman coins and other bronzes.
The advantages and limitations of lead isotope studies have been discussed in detail elsewhere but two limitiations must be mentioned here. While individual ore deposits are characterized by their isotope ratios, these ratios are not necessarily unique to individual mines. Galena ores occurring in widely-separated areas may sometimes be nearly identical. Consequently, one can usually state without ambiguity that a given specimen could not have come from some particular deposits, but in making an attribution, one can go only so far as to state that a lead came either from some particular deposits or from some other deposit (known or unknown) which has similar ratios. This is termed “overlapping.” In addition, if leads from different sources are melted down together and reused, the resulting isotope ratios lie somewhere between the original leads. This is termed “mixing.” Also, the method does not distinguish between ancient metallic objects and modern objects made from melted-down ancient metals.
Figure 1 summarizes data for some 800 specimens of ancient leads and ores analyzed previously. Group L consists of a suite of ores from the Laurion region in Greece, and of objects which are believed with good reason to have been made of Laurion lead. Group E contains many leads from England, some from European mines, and, towards the upper left part of the loop, several from objects associated with Constantinople and its environs. Group S contains relatively few samples, mostly from Spain, Wales, and Sardinia. Early Egyptian leads fall off to the left.
Fig. 1.
Isotope ratios for lead extracted from various ancient materials. The groups were developed from data from some 800 specimens. (Most of the East Asiatic specimens analyzed fall off the graph to the right.) The diagonal is a reference line showing the general trend of the data.
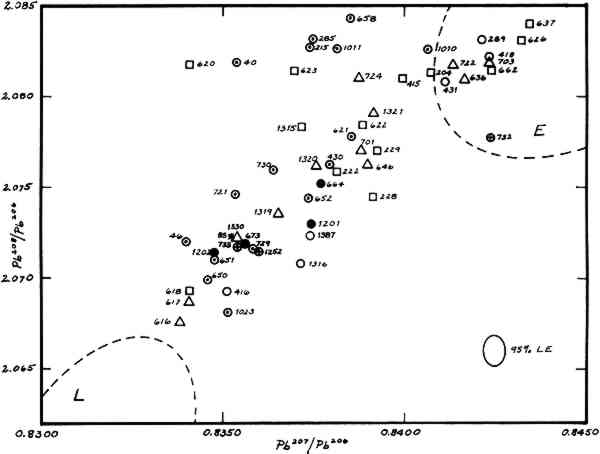 |
The area of the graph between the Groups L and E (partially overlapping Group E) has been called Group X. It contains leads associated—in an archaeological sense—with two regions, and is an exampe of overlapping. Within this range are many specimens from Italy and from around the shores of the Eastern Mediterranean, referred to here loosely as The Levant. It also contains a few objects found in Asia Minor. We are presently of the opinion that the leads in objects found in these two regions, and falling on this part of the graph, came for the most part from geologically similar mines in Italy and in Turkey, Syria, or Iran. It is worth noting that we have found no examples of leads from other regions of the ancient world which fall in Group X, with the exception of leads extracted from a few Parthian and Sasanian silver coins.
3 RESULTS
The isotopic analyses were carried out at the National Bureau of Standards employing high-accuracy methods of mass spectrometry (Barnes et al. 1974). Results are compiled in Table 1 and plotted in Figure 2, along with a selection of other ancient leads. Duplicate determinations were run on samples 664, 1201, and 1202. The results agree closely with one another, so only the mean values for each sample are plotted. Because sample no. 673 was so small only a single determination could be run. The caption gives a key whereby samples with regional associations can be identified. The regions involved are Laurion, Italy (really Etruria and southward), Greece, The Levant, Constantinople, and Asia Minor. As can be seen in the graph, the alloys of the horse are clearly within Group X.
TABLE I
Fig. 2.
Isotope ratios for lead extracted from four samples of the bronze horse, shown with comparative leads. Solid circle = MMA horse; circle with dot = Italy; circle with cross = Greece; square = “Levant”; triangle = Constantinople or Asia Minor; open circle = uncertain, but from one of the above sources; asterisk = galena ore. Isotope range shown includes Group X, with Group L (Laurion) to the left and Group E to the right.
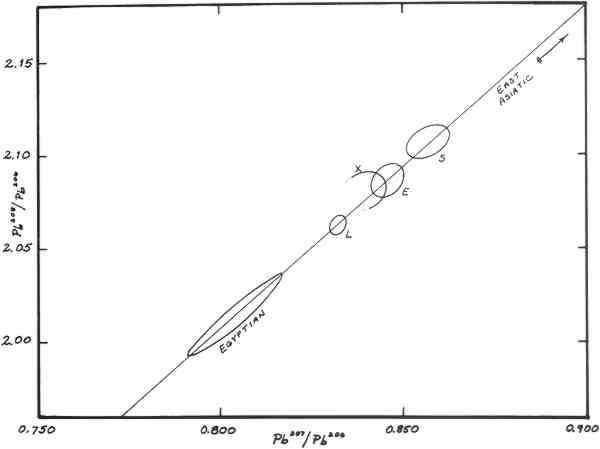 |
4 INTERPRETATION OF RESULTS
In one sense the experimental results are quite conclusive. However, the interpretation of these results, that is, the translation of comparisons made on a graph into statements regarding the proper attribution of the horse, is complicated by three factors. Two of these are the marked difference in the lead levels in the two alloys, and the question of their contemporaneity. The third complication, the “awkward” level of lead in the body alloy, is alluded to below.
It is apparent from Figure 2 that the samples from the body and the cast-on leg differ somewhat from one another. The difference is greater than could have been introduced by experimental error, but nevertheless, there is a very good chance that these two leads come from the same mining region. Viewed against the whole background of leads we have studied, they are really “more alike than unlike.”
It is easier to consider the cast-on leg first. Because the lead in the leg is not of the Laurion type, it is quite unlikely that this alloy, containing such a large proportion of intentional lead, could have been prepared in Greece during the Classical Period—or, for that matter, during any other ancient period. Laurion lead was so abundant throughout Greece in ancient times that we believe virtually no other types of lead found their way into alloys prepared there (except perhaps in the north.) Thus, if the leg is an ancient repair our data strongly suggest that regardless of where the horse might have been made, it was repaired in a location where Group � lead was abundant; i.e., in Italy or the Eastern Mediterranean or Asia Minor, but not in Greece.
If the leads in the samples of the horse are not from the Laurion region, where then, could they have come from? It is likely that the lead in the cast-on leg came from Italy, since samples 673 and 1202 closely resemble several leads known to have come from there. Because of overlapping, however, an alternative is that the leads could have come from the same mines which supplied those found in other Group-X objects from the Levant or Asia Minor. The ore sample 85, a galena from Akdag Maden in Eastern Anatolia, is a very close match for the lead in the cast-on leg. Also, Chamberlain and Gale (1976) and Gentner et al. (1978) have reported results for ores from Western Turkey, Thasos, and Filippoi which are similar to these leads. As far as we know, none of the other mining regions of the ancient world (Greece, Britain, Wales, Spain, France, Sardinia, Egypt, or North Africa) provide this kind of lead.
The comparisons from which these inferences were made can be seen from the arrangement of the data in the table, where specimens are listed according to similarity of ratios. Proximity in the table reflects similarity of isotopic ratios.
Samples 664 and 1201 are from parts of the original casting. Therefore, unlike the samples of the leg, their lead is inextricably connected with the manufacture of the horse. One might be inclined to apply again the same argument used above, saying that because the lead in the low-lead alloy is not of the Laurion type, the horse itself could not have been made in Greece in the Classical or Hellenistic periods. But this would be true only if it were known for sure that the lead was an intentional additive, or that it was an impurity introduced at the founding site. Unfortunately, neither of these conditions is known to hold, so the interpretation is not straightforward.
Spectographic analyses have shown that this alloy contains only about 0.45% lead. If a lead concentration is more than about 2–3% it can reasonably be considered an in tentional addition, and if it is down at about the 0.1% level it can reasonably be considered an impurity in an ore or just stray contamination. But the intermediate range, where the horse lies, is more awkward to explain. Some of the ways this level of lead could have found its way into the bronze intentionally are as an impurity in the copper, as an impurity in the tin, as an adulterant of the tin, or as a residue of some metallurgical process involving the copper or tin. Consequently, the isotope ratios may be telling us more about the sources of copper or tin than about where the horse was made. Actually, alloys containing this level of lead are frequently encountered among Classical and Hellenistic bronzes, so frequently, in fact, that there must be some systematic reason for it. (For example, there are Roman tin: lead alloys with ratios of about 20:1 of tin over lead. These, if incorporated into bronzes, would yield lead contents of about 0.5%.)
In any event, we have adopted a more-orless empirical approach towards this problem, and have undertaken determinations on a large suite of early Greek and Italian bronzes to see what kinds of lead they contain. Our hope is that regardless of what is ultimately found to be the explanation of this intermediate concentration of lead, the results will nonetheless show how the lead in the body of the horse compares to those in other lightly-leaded bronzes of known date and provenance.
5 COMPARISONS WITH OTHER OBJECTS
The following objects contain leads closely resembling those found in the samples of the horse.
Numbers 650 and 651 are from a large bronze statue found at Casanuovo, near Reggio (Mitten and Doeringer, 1967). It dates from the 2nd cent. A. D. and is in the Museum of Fine Arts, Boston (no. 01.7524). Both samples are of the ancient welding metal used to join cast sections of the statue in its original assembly. Like the pieces they join, they are heavily leaded, containing, respectively, 19.9% and 23.8% lead (Steinberg, 1973; Brill, et al. 1973).
Sample no. 46 is from a small piece of metallic lead found in an Archaic tomb in Etruria, La Montangnola, dating from the 7th cent. B. C. This lead is almost certainly of local origin (Brill and Wangsler, 1962).
Sample no. 1330 illustrates the overlapping effect. It is from a lead clamp (thought to be ancient) used to mend a Phrygian pottery vessel (Simpson, 1973). The vessel, dating from ca. 625 B.C., is in the collection of the Museum of Fine Arts, Boston (no. 1971.297). Presumably the repair was made somewhere in Anatolia, and would be of a local type of lead. It is a near perfect match for the galena ore from Turkey mentioned above (no. 85), which came from Akdag Maden in Yozgat Province (Brill & Wampler, 1962).
Samples nos. 721 and 729 are both from Etruscan bronzes (Mitten & Doeringer, 1967), the first from the handle of a bronze vase of the 5th cent. B.C. and the second from a bronze Lasa figure of the late 4th cent. B.C. (Fogg Museum nos. 1974.75, 1966.109).
Sample no. 733 is from a piece of bronze armor found at Dendra in Greece. It is of Mycenaean date. Sample 1252 is a Greek bronze of the 4th century B.C. These two lightly-leaded samples of Greek pedigree suggest that some of the copper used for making Greek bronzes might have come from Italy (because of the similarity to heavily-leaded Etruscan bronzes) or from those regions of Turkey which produce an isotopically-similar galena ore.
Sample no. 652, which falls between the points for the two body-alloy samples of the horse, is from a bronze thymiaterion in the R. H. Lowie Museum (no. 8-3406) (Mitten & Doeringer, 1967; Brill et al. 1973). Sample no. 730 is a bit problematical. It is from a figure of “Alexander with Lance” (Fogg Museum no. 1956.20), which is thought to be Italian and of Roman, or possibly, of Renaissance date. Sample 1387 is from the lead anchor stock of the 5th cent. B.C. Porticello wreck which was carrying, among other things, ingots of Laurion-type lead (Eiseman, 1978).
6 CONCLUSIONS
The leads in the cast-on leg and in the body alloy both fall in Group X. They are similar to one another, but not identical. These results are consistent with an assumption of an ancient origin for the horse, but are not sufficient to prove it is ancient. There is nothing in our findings to suggest that the horse is modern.
Regardless of where the body of the horse was made, it is doubtful that the cast-on leg repair was carried out in Greece. Because many of the horse's nearest neighbors, isotopically, are attested Italian leads, we prefer Italy as the most reasonable attribution for the alloy in the cast-on leg. However, that alloy could have been prepared with lead from Asia Minor, or whatever other mining regions produced the lead used in the Levant.
Until we have a better understanding of why so many early bronzes, including the body of the horse, have lead concentrations of about 0.5%, it will be difficult to interpret the lead isotope data. If it is assumed that the lead was introduced into the alloy as an impurity of the copper, it appears that the copper came from either Italy or Asia Minor. The horse could then have been made in Greece. If it is assumed that this low level of lead was introduced intentionally or by accident at the location where the alloy was prepared, then it is unlikely that the horse was made in Greece. It would then be more likely to have been made in Italy, or possibly in Asia Minor. It is realistic to expect that as more data become available on lightly-leaded bronzes, much of this ambiguity will be resolved, and it should become possible to make a reliable attribution for the horse.
7 SAMPLE DESCRIPTIONS
The following descriptions were provided with the samples by Ms. Lefferts and Dr. Meyers.
- 664—“Sample A.” From bronze horse, either of Classical Greek origin or a modern forgery. MMA acc. no. 23.69. Pb content approximately 0.45%.
- 673—“Sample Y9” From bronze horse; cast-on left rear leg. Pb content approximately 18%.
- 1201—“Horse, beneath plug, � 44 mg.” Sample extracted from under a plug in the upper (sic) left hind leg of the bronze horse. This is part of the original casting. The lead content is about 0.5%.
- 1202—“Horse, left hind leg, � 15 mg.” Drilling from the repaired section of left hind leg of the bronze horse, Acc. no. 23.69. Former analyses showed it to contain about 20% lead.
- Detailed descriptions of all the other samples listed in the table are on permanent file at The Corning Museum of Glass.
ACKNOWLEDGEMENTS
The authors thank the many people at the National Bureau of Standards laboratories, both regular staff members and visiting scientists, who have assisted us over the years by preparing and running the lead samples referred to here. In particular these include L. Dunston, J. Gramlich, G. N. Hanson and J. M. Wampler. We are grateful as well to the persons and institutions who donated the comparative samples listed in the table. In order of first appearance of their samples in the table they are: Arthur Beale (Fogg Art Museum), Arthur Steinberg (Massachusetts Institute of Technology), Earle R. Caley (Corning Museum of Glass), A. Neppi Modona (Museum of Fine Arts, Boston), Astone Gasparetto, Babis Deilakis (The Nafplion Museum), Jane Scott (Sardis Excavations), Cynthia Eiseman (University of Pennsylvania), The R. H. Lowie Museum, G. Bovini, M. V. Seton-Williams, Lawrence Cope, E. Oren, Erik Sj�quist, Honor Frost, David Grose, Sidney Goldstein, and Dan Barag.
REFERENCES
Barnes, I.L., Brill, R.H., Murphy, T.J., and Shields, W.R. (1974). “Isotope Analysis of Laurion Lead Ores.” In Archaeological Chemistry. Washington: American Chemical Society, Advances in Chemistry Series, no. 138, pp. 1–10.
Brill, R.H., and Wampler, J.M. “Isotope Studies of Ancient Lead.” American Journal of Archaeology, 71, pp. 64–77.
Brill, R.H. (1970). “Lead and Oxygen Isotopes in Ancient Objects.” Philosophical Transactions of the Royal Society London A, 269, pp. 143–164. This paper also appears in (T.E.Allibone, ed.) The Impact of the Natural Sciences on Archaeology, (1971). London: Oxford University Press, pp. 143–164.
Brill, R.H. and Shields, W.R. (1972). “Lead Isotope Studies of Ancient Coins.” In (E.T.Hall and D.M.Metcalf, eds.) Methods of Chemical and Metallurgical Investigations of Ancient Coinage. London: Royal Numismatic Society, Special Publication No. 8, pp. 279–303.
Brill, R.H., Shields, W.R., and Wampler, J.M. (1973). “New Directions in Lead Isotope Research.” In (William J.Young, ed.) Application of Science in Examination of Works of Art. Boston: Museum of Fine Arts, pp. 73–83.
Chamberlain, V.E., and Gale, N.H. (1976). “Isotopic Composition of Lead in Greek Coins.” International Symposium on Archaeometry, Edinburgh.
Eiseman, C.J. (1978). “The Porticello Shipwreck: Lead Isotope Data.” MASCA Journal, 1, p. 18.
Gentner, W., Muller, O., and Wagner, G.A. (1978). “Silver Sources of Archaic Greek Coinage.” Naturwissenschaften, 65, pp. 273–284.
Mitten, D.G., and Doeringer, S.F. (1967). Master Bronzes from the Classical World. Fogg Art Museum.
Simpson, W.K. (1973). “Century Two, Collecting Egyptian and Near Eastern Art for the Boston Museum.” Apollo, 98, pp. 255–56, Plate III.
Steinberg, A. (1973). “Joining Methods on Large Bronze Statues.” In (W.J.Young, ed.) Application of Science in Examination of Works of Art, Boston: Museum of Fine Arts,
APPENDIX
1 APPENDIX V
1.1 Analyses Of The Core Sand Found In The HorseFrederick R. Matson The Pennsylvania State UniversityA sample of the dark gray core sand removed from the horse was studied. It was granular, porous and friable. A few lumps of the material retain the form of the inner surface of the casting, but these show no evidence of greater heat alteration than the rest of the core sample. Two thin sections were prepared for petrographic study. Other samples were washed with acid to remove the carbonate binder, and a magnetic separation was then made to concentrate further the minerals for study in index of refraction liquids in powder mounts. Small portions were fired at 750� and 800�C. to oxidize them for further examination. The chemical composition was determined by a microprobe analysis. From the resulting data a rational analysis was calculated to determine the approximate proportions of the components in the sand core.
2 PETROGRAPHIC EXAMINATION
A study of two thin sections of lumps of core material and the examination of acidwashed and magnetically separated grains mounted in index of refraction liquids showed that the sand consisted principally of quartz grains in a calcareous ground mass in which were some well preserved foraminifera and occasional grains of iron compounds, sandstone, feldspar and zircon. The previous texture of the thin section prepared from an uncrushed lump of the core can be seen in Figure 1.
Fig. 1.
Photomicrograph of the core sand, taken through crossed Nicol prisms. The white grains are quartz. The finetextured gray material is the marl that serves as a chemical and physical binder. The four-chambered foram near the center is 0.4 � 0.15 mm in size. Other forams, some fragmentary, can be recognized. The black holes are indicative of the porous structure of the core. The photomicrograph shows an area covering 3.15 � 2.1 mm of the petrographic thin section.
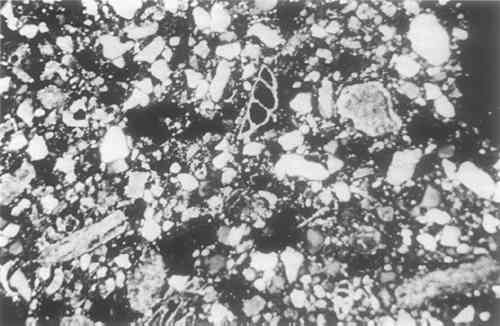 |
The quartz grains were subangular to subround in shape. The most common grain size was 0.1–0.15 mm in length, grading down to 0.02 mm. The rare larger grains were 0.25 � 0.15 mm in size. Rutile needles or lines of bubbles were seen in some pieces. There was no indication of the presence of sharp splinters of quartz such as one sees in crushed quartz bodies like those of Egyptian faience. Therefore, it is likely that the quartz used for the core was levigated either naturally, producing fine textured deposits, or was washed by man. Most of the grains would pass through a 100 mesh sieve and would be terned fine to very fine sand grains in the Wentworth scale of grain sizes. The presence of an occasional subrounded grain of very fine textured sandstone might suggest the rock origin of much of the quartz in the core. A few grains of chalcedony were found in the acid-washed non-magnetic fraction.
Calcite was the other abundant mineral present. Most of it was cryptocrystalline in texture, and of about the same range as the quartz, but an occasional piece was 0.7 mm in length. Within the larger grains one could sometimes find clear areas of crystalline calcite. This would suggest that there may have been thermal disintegration of the calcite in preparation for its use in the core or from the heat of the casting process itself. Well preserved calcareous foraminifera of several kinds are sparsely present, and are up to 0.5 mm in size, although 0.25 is a common size. Their presence would indicate that the calcareous component of the core had not been pulverized. Fine grained calcareous material coats most of the quartz grains and clearly served as a binder in the core. It is useless to speculate on the relative amounts of calcium carbonate, calcium hydroxide and related compounds that may originally have been present in the binder more than two thousand years ago, but their origin was certainly in an ancient sea bed deposit that may have become a marl that was used with quartz to form the core.
Small red and black iron oxide particles, probably goethite and magnetite, can be found in the powder separations as well as in the thin sections. An occasional grain of feldspar, perthitic in structure, occurs as do a few zircon grains which can be found in the powder separations. Extremely fine birefringent clay flakes that remained in suspension after the washing of the acid-treated samples were collected on filter paper in a microfunnel. They had an index of refraction of about 1.548, but nothing can be said as to the type of clay mineral present, for the samples were too small for X-ray diffraction studies.
3 FIRING TESTS
Samples placed in porcelain crucibles were fired to 750� and 800� C. in an electric furnace in an oxidizing atmosphere to remove any carbon that might be present from the decomposition of wax or other organic materials used in the preparation of the mold and core, and possibly to oxidize ferruginous materials to a ferric state if they had not been partially vitrified. The samples were fired from room temperature to 750� in 50 minutes. They were held at this temperature for ten minutes. The first sample was then removed and the furnace temperature increased to 800� in another ten minutes. After maintaining this temperature for ten minutes, the second sample was allowed to cool in the kiln. The resultant colors of the core sand, as matched on the Munsell Soil Color Charts, were:
After both firings some granules remained dark gray in color, those at 800� being the darkest. They might be metallic flakes from the bronze casting or the iron armature. None of the original core material that was studied under a binocular microscope had either brown or pinkish gray grains present. This was not surprising, for during the melting of the wax and the casting and cooling of the horse the core zone would have been in a strongly reducing atmosphere.
4 CHEMICAL COMPOSITION
A sample of the core material was fused with a flux into a glass bead and analyzed by a microprobe technique with an X-ray fluorescence spectrometer. The results are reported in Table I. They confirm the petrographic analysis showing that the core consists chiefly of quartz and calcareous material. A so-called rational analysis of the data was made, assuming on the basis of the mineralogical evidence that the small amount of alkali present occurred as feldspars, the alkaline earths as carbonate, the alumina other than that in the feldspar was present as clay, the metals as oxides, and the great residue of silica as quartz. The theoretical molecular compositions of the minerals were used in the calculations. Such an analysis, based on many assumptions, is only roughly quantitative, but it does offer an approximation of the proportions of the materials used to prepare the core. The results, presented in Table II, show that roughly equal amounts of quartz sand and a marl were used to prepare the core material. The assumption that all of the elements reported analytically as oxides were present as the minerals selected for the rational analysis can of couse be questioned, but the allocation of the lesser ingredients is unimportant considering the nature of the sample analyzed and the approximate proportions probably used when the core was prepared. The alkalis might well have been present chiefly in the clay or as soluble salts, for little feldspar was observed petrographically. The rutile occurs as inclusions in the quartz, although some could be present as ilmenite. The very small amount of MgO could have been in the clay or as a soluble salt instead of being associated with the calcite. The significant amount of iron, almost 5% when calculated as Fe2O3, could occur as magnetite in the quartz sand as well as in the clay, although little was observed petrographically. However, the strongly magnetic fraction of the core materials that could be isolated consisted primarily of what appeared to be dark gray ferrous clay-like material.
TABLE I Chemical Composition of the Core Sand Glass Bead/Microprobe Analysis
TABLE II Mineral Composition of the Core Sand Rational Analysis
The clay and the calcite are grouped together in the rational analysis because the evidence from the thin sections and the study of powdered samples in immersion oils showed that the calcite was chiefly present in cryptocrystalline form in a matrix of clay, with some foraminifera being present. Such an association in sea-bottom deposits would be normal.
5 CONCLUSIONS
The core sand from within the horse is composed of approximately equal parts of clear quartz sand and a calcareous paste that can be termed a marl—a sticky, naturally occurring deposit derived from an ancient sea bed deposit. These two ingredients can be used effectively to prepare a core for a casting. No evidence was found of an organic binder nor of organic inclusions such as grass or straw that are at times used in cores. The strongly reducing condition indicated by the dark gray color of the core suggests an internal atmosphere during the casting. This could readily have been produced when the wax was melted, reinforced by the limited access of oxygen during the casting process.
Nothing can at present be said on the basis of the petrographic study as to the sources of the quartz sand and the marl that might suggest the region of manufacture. However, clues do exist that may be of use as more cores are studied. Many of the quartz grains contain well-developed rutile needles. The types of forminifera present in the marl might help define its source. The absence of micas and the rarity of chalcedony, together with the presence of an occasional grain of very fine sandstone and perthic feldspar may eventually be diagnostic. The grain size distribution—0.1–0.5 mm with a second common concentration at 0.5 mm.—may be of regional significance. The quartz sand could have been washed and perhaps sieved before use, or may have come from a naturally levigated deposit which perhaps could be located.
NOTES
. All photographs are by James R. Howard of the Laboratory for the Study of Archaeological Materials, M.I.T.
|