THE CONSERVATION OF WET MEDIEVAL WINDOW GLASS: A TEST USING AN ETHANOL AND ACETONE MIXED SOLVENT SYSTEM
D. R. GRIFFITHS, & A. M. FEUERBACH
ABSTRACT—This article describes some of the factors associated with the deterioration of ancient silicate glasses. On the basis of this model, a procedure is proposed for the conservation of an assemblage of excavated, wet, painted medieval window glass fragments. The fragments had been stored wet since excavation and needed to be consolidated for long-term storage in a way that was inexpensive, quick, and safe. The proposed treatment removes the water with ethanol and consolidates the fragments with Paraloid B-72 (an acrylic polymer) dissolved in a mixed ethanol and acetone solvent system. Examination of the stored samples five years after this treatment indicates that the method appears successful.
TITRE—La conservation du verre arch�ologique humide provenant de vitraux du Moyen ge: un test avec une solution combinant l'�thanol et l'ac�tone. R�SUM�—Cet article d�crit quelques-uns des facteurs reli�s � la d�t�rioration des verres anciens � base de silicate. Sur la base de ce mod�le, on propose une m�thode pour la conservation d'un groupe de fragments en verre peint provenant d'un vitrail moyen�geux qui ont �t� excav�s encore humides. Les fragments avaient �t� gard�s sous des condition humides depuis leur excavation et devaient �tre consolid�s de fa�on peu co�teuse, rapide et s�re avant leur mise en r�serve � long terme. Le traitement qui est propos� consiste � remplacer l'eau avec de l'�thanol et � consolider les fragments avec du Paraloid B-72 (un polym�re acrylique) dissous dans une solution � base d'�thanol et d'ac�tone. L'examen des �chantillons en r�serve depuis maintenant cinq ans apr�s un tel traitement indique que la m�thode semble bien r�usssir.
TITULO—Conservaci�n del vidrio de una ventana medieval h�meda: prueba usando un sistema de solventes mezcla de etanol y acetona. RES�MEN—El presente art�culo describe algunos de los factores asociados con el deterioro de vidrios de silicato antiguos. Con base en este modelo, se propone un procedimiento para la conservaci�n de un grupo de fragmentos de vidrio de una ventana medieval pintada que se hab�an mantenido h�medos desde su excavaci�n. Estos fragmentos necesitaban ser consolidados para su almacenamiento a largo plazo de una forma barata, r�pida y segura. El tratamiento propuesto consisti� en remover el agua con etanol y consolidar los fragmentos con Paraloid B-72 (un pol�mero acr�lico) disuelto en un sistema de solventes mezcla de etanol y acetona. La inspecci�n de las muestras cinco a�os despu�s del tratamiento indica que el m�todo fue exitoso.
1 INTRODUCTION
The conservation procedure proposed in this article was developed in response to the need to conserve an assemblage of excavated, wet, painted medieval window glass fragments. They appeared on art-historical grounds to date from a variety of periods. The treatment had to remove the water in which the fragments had been stored since excavation, consolidate the deteriorated pieces, and leave them in a fit state for long-term storage. While achieving these aims, the treatment also had to be inexpensive, require no specialized equipment, be relatively quick to perform, and involve a minimum of health and safety risks. The proposed treatment removes the water by replacing it with ethanol and consolidates the fragments with Paraloid B-72 (an acrylic polymer) dissolved in a mixed ethanol and acetone solvent system. Examination of the stored samples five years after this treatment indicates that the method appears successful. It may thus be worthy of further consideration and testing by others who need to conserve glass in a similar condition.
To explain some of the rationale behind the treatment proposed, the article includes a brief description of the mechanisms by which archaeological glass is attacked, the compositional and environmental factors influencing the rate of deterioration, and some of the features that these deterioration processes create. Although some of this information may be found elsewhere (for example, in Weyl and Marboe 1962–67; Newton and Davison 1989; Pollard and Heron 1996), other aspects of the model are more speculative and are the subject of current experimental work.
Fig. 1.
This reaction depicts the formation of glass from silica and an alkali metal oxide
 |
Fig. 2.
Alkali metal ions are lost from the surface layer of the glass and replaced by hydrogen ions accompanied by water. Sodium ion migrates into the solution, which becomes progressively more alkaline, in effect becoming a solution of sodium hydroxide.
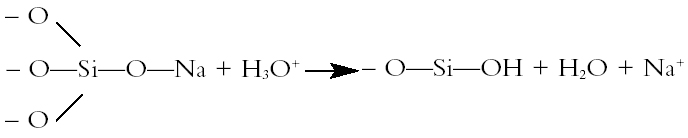 |
2 ARCHAEOLOGICAL GLASS AND ITS DECAY
2.1 STRUCTURE AND MECHANISMS OF ATTACK
Archaeological glasses are all silicate glasses. The melting temperature of pure silica (1710�C) is too high to be reached using traditional technology, so fluxes such as alkali metal oxides had to be used to produce a glass of lower melting point. Figure 1 depicts the formation of glass from silica and an alkali metal oxide (M is an alkali metal such as lithium, sodium, or potassium). The alkali metal compounds act as fluxes that break up the silica network and so lower the viscosity and glass transition temperature of the resulting melt.
Aqueous attack on alkali metal silicate glasses has two stages. The first stage is ion exchange. Alkali metal ions are lost from the surface layer of the glass and replaced by hydrogen ions accompanied by water. The reaction in figure 2 shows the sodium ion migrating into the solution, which becomes progressively more alkaline. In effect, it becomes a solution of sodium hydroxide (NaOH). The rate of leaching of alkali metal out of the glass is governed by rates of diffusion. The nonbridging oxygens (i.e., those that do not form a link or bridge between two SiO4 tetrahedra in the glass but instead are bonded on one side to silicon and on the other to an alkali metal such as sodium or potassium) may be pictured as being more polarizable than the bridging oxygens and thus more susceptible to attack by a hydrogen ion. This depiction is in line with the observation that potassium silicate (potash) glasses are more susceptible to aqueous attack than sodium silicate glasses of the same molar composition, the potassium ions being larger, the center of positive charge being further away, and the corresponding nonbridging oxygens thus being more polarizable than their sodium silicate counterparts (Weyl and Marboe 1962–67).
The second stage in aqueous attack on silicate glasses involves hydrolysis and breakdown of the silica network. When the alkalinity of the attacking solution rises above a pH of 9, hydrolysis of the hydrated silicate network occurs, with hydroxyl ions attacking the silica network itself eventually forming Si(OH)62- ions, which dissolve in the alkaline solution. As the silica network breaks down, it becomes less of a barrier to diffusion, and the rate of leaching of alkali metal into the solution becomes limited by factors other than diffusion.
Both ion exchange and network dissolution occur to some extent at all pH levels, but ion exchange is the dominant mechanism of attack on glass below a pH of 9 and network dissolution predominates above a pH of 9.
The leached surface layer of a silicate glass being attacked by water is under tension because the H+ ions are far smaller than the alkali metal ions they replace (Metcalfe et al. 1971). The tension in the leached layer may cause it to crack or break away from the underlying unaltered glass, thus exposing a fresh glass surface for the cycle of leaching to start again. The surface ion–exchanged layer may also be to some extent hydrated and bulked out by water: such layers may lose water and crack on drying.
2.2 COMPOSITIONAL FACTORS INFLUENCING RATE OF DETERIORATION
The factors that influence rates of deterioration of glass may conveniently be considered in terms of the composition of the glass, the state of its surface, and the nature of the environment to which it is exposed. The fact noted above that potash glass is less durable than the corresponding sodium glass is of particular import in the conservation of medieval window glass, much of which has a high potash content owing to the use of wood ash as the source of alkali to flux the silica. The presence of moderate quantities of alkaline earths, aluminium, or phosphorus improves the durability of the glass.
Glass durability is not solely a function of bulk composition. Microheterogeneities in the glass structure, such as those arising from phase separation during cooling of the glass melt or poor mixing of the components of a melt, are likely to influence the durability of glass considerably. In the case of phase separation during cooling, one phase may be less durable than the corresponding homogeneous glass. Furthermore, the weathering of a less durable phase may produce a local environment that is detrimental to the stability of the other phase, even though the more durable phase might be quite stable with regard to the overall ambient environment. The tendency of a glass melt to undergo phase separation during cooling is a function of composition and also depends on whether the rate of cooling is slow enough to allow time for phase separation to occur. If phase separation produces droplets much greater than 180 nm in diameter and the refractive indices of the two phases are significantly different, the glass will be cloudy or white like milk, due to scattering of light by the droplets of one phase suspended in another.
2.3 SURFACE STATE FACTORS INFLUENCING RATE OF DETERIORATION
Apart from composition, a second significant factor controlling the deterioration of silicate glass is the state of its surface, since that is where the deterioration begins. One of the most important parameters governing the rate of glass deterioration in a specific instance is the ratio of the glass surface area (SA) to the effective volume (V) of the attacking solution (SA/V ratio). This parameter controls the time that must pass before, through the mechanism of ion exchange, the attacking solution reaches a pH of 9 and network breakdown starts. pH is a measure of the concentration of hydrogen ions in aqueous solution, so the change in pH brought about by a given number of alkali metal ions replacing hydrogen ions in solution is greater for small solution volumes. Surface roughness increases the surface area of the glass available to undergo ion exchange with the aqueous medium. Cracks, scratches, pores, and indentations contain very small volumes of solution surrounded by glass surface. Very little alkali has to diffuse into the solutions held within them to raise the pH above 9.
Surface defects promote rapid attack, so conservation procedures should, as far as possible, avoid roughening or scratching the glass surface. High SA/V ratios should be avoided when packing samples. Flat pieces of wet glass should not be left lying against each other for long periods with a thin film of water between them. If glass needs to be packed wet, there should be lots of water rather than a little, and alkali-resistant materials should be used. The use of flat or flexible sheet materials in a way that keeps a small volume of water next to the glass should also be avoided. Changing the holding solution periodically helps to avoid the development of elevated pH and may also limit the development of organic growths.
Stress in the surface of the glass is another factor that is detrimental to its stability. Stress causes disproportionation of bonding forces and regions of greater polarizability and greater chemical vulnerability than are present in the absence of stress (Weyl and Marboe 1962–67). Stresses arise during cooling of glass after manufacture and are due to ion exchange. Grinding causes plastic deformation and a region of residual stress below the tracks of the abrasive particles, in addition to the detrimental effects of scratches, surface roughness, and cracks noted above. Inhomogeneities such as regions of phase separation, bubbles, and crystals cause stress because of their different mechanical and thermal properties.
2.4 ENVIRONMENTAL FACTORS INFLUENCING RATE OF DETERIORATION
Apart from composition and surface state, the third class of factor affecting the durability of glass is the environment with which the glass is in contact. The most damaging aspect of the ambient environment is water vapor. A thin layer of water condenses on glass at all relative humidities above zero. Leaching of alkali occurs in the ordinary air, and not only when the surface of the glass is palpably wet. For example, sodium ions are leached out of soda-silica glass in air and react with water and carbon dioxide to form crystals on the surface of the glass. Potassium salts are particularly deliquescent, so crystals of potassium salts forming on the surface of a potassiumsilica glass might result in the repeated re-creation of aggressive droplets of solution on the glass surface during commonplace cycles of relative humidity.
A number of tests on the effects of humidity have been published, and the results are not wholly clear-cut in their implications. It may safely be said, however, that the worst attack occurs at above 50% RH. It is not entirely clear whether condensation is more or less deleterious than high humidity, but it is clear that both are very deleterious to the condition of susceptible glass. Heavy condensation may be less harmful than slight condensation because droplets running off the surface may wash away the ions leached out of the glass and so prevent the buildup of high pH in the droplets. Cycles of condensation and drying would cause the droplets to have an increasingly high pH as they dried out and would leave a precipitate of deliquescent salts. Upon a subsequent increase in relative humidity, these might again be the points at which condensation would first occur. This idea is speculative at present, but experiments are being conducted to test the model. Such a mechanism might explain some of the deterioration phenomena observed on ancient glass, such as the development of pits.
The importance of pH as an environmental factor influencing glass deterioration has already been noted. Frequent replacement of the attacking solution (in the case of glass in wet storage) or washing of glass in windows or on display might lower the mean pH of the attacking solution. Buffers control pH in solution but they may also have other effects on the leached surface layers of the glass surface, either promoting or impeding the passage of particular constituents. Similarly most ions in solution have some effect on the transport properties of the leached layers of the glass, but understanding of these processes is not yet at a level where solutions of a particular composition can be recommended for uses as holding solutions or to impede or prevent deterioration. Although it has been attractive to implicate gaseous atmospheric pollution as contributing to the deterioration of glass exposed to urban environments, there seems to be little evidence to support such a case.
2.5 TYPES OF DETERIORATION
The interaction of glass with the environment gives rise to a number of fairly common visible signs of deterioration. The leached layer is a hydrated layer of alkali-depleted hydrogen glass. It is not usually visible to the naked eye, although the change in refractive index between the leached and the bulk glass can be seen in section under the microscope. The behavior of the leached layer is crucial in governing glass deterioration (Hench and Clark 1978).
Fracture is rendered more likely by internal stress (from cooling, inhomogeneities, ion exchange, etc.), since less additional stress has to be added to the preexisting internal stress to exceed the strength of the material and result in fracture (Metcalfe et al. 1971). If thin layers crack off the surface of the glass but remain in place, iridescence may be observed due to constructive interference of light of certain wavelengths reflected from successive glass-air interfaces. Drying corroded glass reveals existing cracks because light is reflected from the air-glass interface to a far greater extent than from the previous water-glass interface (due to greater differences in refractive indices). In addition to revealing existing cracks, drying probably creates more cracks from shrinkage associated with dehydration of a hydrated glass layer. Cracks and stresses are not purely mechanical phenomena; they are accompanied by chemical changes associated with changes in the polarizability of oxygen ions (Weyl and Marboe 1962–67).
Crizzling and weeping are generally observed in glass with less than 5 mol% alkaline earth metal oxide, this being too low to stabilize the amount of alkali present (Brill 1975). In such glasses a very thick hydrated layer forms, which may crack into a crazy paving pattern (crizzle) in any case and will crack if stored at low ambient relative humidity. Where low alkaline earth glasses have a high potash content, potassium salts deposited on the glass surface as a result of ion exchange with water adsorbed on the surface may deliquesce if the ambient relative humidity becomes too high (weeping).
Blackening is quite commonly found on the surface of glass, particularly excavated glass, and it may exhibit a dendritic invasion into the interior, perhaps along microcracks. The staining might be due to lead sulphide, ferric ions, or manganese dioxide. Our preliminary electron beam analyses on excavated glass indicated that at least in the few samples studied so far, there was a markedly raised manganese concentration in the blackened areas. A similar phenomenon was noted by Alten (1988). The blackening may be the result of bacteria that use manganese in their metabolic processes and thereby concentrate it on and in the glass. Further work will be undertaken on the cause and possible remedy of this blackening.
A feature found particularly on medieval window glass is pitting. The pits may contain crystalline deposits or be empty. They may occur in clusters or lines but are not well understood. Pit distribution may be correlated negatively or positively with devitrification, organic growths, leading, painting, staining, surface deposits, or scratching, possibly by the effect of these factors on condensation patterns and the repeated occurrence of alkaline solutions at particular points on the surface. Under the microscope, pits can often be seen to be comprised of concentric hemispheres of glass originating from a point on the surface, sometimes with those hemispheres nearest the surface having disintegrated into a crystalline mass. The hemispheres are under tension, and if pressed with the point of a pin will sometimes detach themselves from the underlying glass and leap into the air. Further research is being undertaken to understand this phenomenon more fully.
Crusts on the surface of poorly durable window glass may range from off-white to black, depending perhaps on pollution and organic growths. The crusts are primarily composed of gypsum (CaSO4.2H2O) or syngenite (CaSO4.KSO4.H2O). They occur on glass that has too much lime and too little silica: the calcium leaches out and forms salts. There are sharp compositional boundaries between crusting and noncrusting glasses (Newton 1969).
Weathering layers are lamellar structures within the glass structure. They have high crystallinity, and elemental concentrations rise and fall in phase with the visible layers. There is often staining around fissures and milkiness. Weathering layers may be formed by leaching followed by the leached layer breaking away from the substrate and restarting the cycle at the new surface. With continued deterioration these layers may be transformed into a crystalline end product. It had been suggested that these layers might reflect annual rhythms and so provide a dating system akin to tree-ring or varve dating. The reliability of this method has been clearly refuted by Raw (1955) and others, but the processes of formation still retain their fascination.
3 THE BEDFORD GLASS ASSEMBLAGE
The window glass for which the following treatment was first conceived was excavated in Bedford, England, in the vicinity of a medieval ecclesiastical building. The glass appeared on art-historical grounds to date from a variety of periods. The glass fragments had been excavated from damp soil under what had become a sand and salt dump for the Bedfordshire Council road maintenance section.
Many of the features of glass deterioration described above were seen in the Bedford glass assemblage. Knowledge of some of the causes and effects of the deterioration process has influenced the choice of conservation treatment. The condition of the glass varied from well preserved to highly deteriorated. These two states are well described by Alten (1988) in her experiments on waterlogged medieval window glass from York, although the milky areas she describes were not noted in the Bedford glass. The highly deteriorated glass was black and opaque. Some pieces had surface pits (presumed to originate from the time the glass spent in a window prior to burial), which often contained white powdery deposits. A couple of pieces of the black Bedford glass were allowed to dry in air to determine the result of this approach. After air-drying, the black deteriorated glass was very fragile. On handling, the black corroded glass tended to crumble into sugary fragments, exposing a thin central core of translucent glass with hemispherical depressions on its surface. Some of these hemispherical depressions and most of the corresponding parts of the black corroded glass that encased it exhibited pronounced iridescence. The better-preserved glass retained a good proportion of its transparency, and this was only slightly reduced by a superficial cloudiness on some pieces after air-drying.
3.1 DECORATION
Many of the pieces had retained their paint, while others had only indirect evidence of painting in the form of differently deteriorated areas resembling painted designs in their distribution. These patterns most probably reveal the decoration that was once painted onto the surface. It may be envisaged that under different circumstances the painted area may be more or less resistant to decay than the surrounding unpainted glass. Factors influencing the effect of painted decoration on deterioration of the glass include the composition of the glass, the composition of the glass paint, the degree of vitrification of the paint, the degree of fusion of the paint to the substrate, and the environmental history of the sample. For example, a paint that is poorly fused to the surface of the glass may trap a small volume of water in contact with the glass, which may quickly become alkaline by ion exchange and then attack the glass network.
It may be of interest to note that where paint has vanished and no ordinarily visible trace of its position remains, it may still be possible to discover the position it formerly occupied by a number of other approaches, none of which were employed with the Bedford glass assemblage but some of which are currently being investigated. For example, areas that were formerly painted would often display subtle differences in surface chemistry compared to surrounding glass that had never been painted. These differences might be revealed by causing a liquid to condense from the vapor state onto the surface of the glass, the tendency to condensation being influenced by surface state and the painted design being transitorily revealed during the condensation process.
3.2 TREATMENT IMMEDIATELY AFTER EXCAVATION
The glass had been excavated a few years before the involvement (ca. 1994) of the present authors. According to a report, the pieces had been repeatedly immersed in baths of tap water after being excavated to remove soluble salts. The fragments had then been placed between wet foam and plastic sheets in resealable plastic boxes. By the time long term conservation was undertaken about two years later by one of the present authors (Feuerbach), two of the boxes contained visible greenish organic growths. More than 300 fragments of painted medieval window glass needed to be removed from their short-term storage containers, documented, and conserved for longterm storage. The work had to be completed within a six-week period. This task was easily achieved, and the conservation process required only occasional attention.
An intermediate stage of temporary wet storage between excavation and conservation obviously carries the risk of mold and algal growth as well as the risk that, unless conscious action is taken to avoid it, damagingly alkaline solutions may form in cracks within the glass structure or in small uncirculating volumes of solution held in scratches or in thin films between the glass and some other surface. Antifungal and antialgal agents might help the situation but may have unknown effects on the glass and its deterioration. The use of pH buffering substances in the holding solution might mitigate the damage due to alkaline pH but may have unknown complexing effects that might detrimentally influence the state of the bulk-leached glass interface, the leached glass layer, or the glass-water interface.
In many cases it may be possible to apply long-term conservation procedures directly from the time of excavation and so avoid the complications of how exactly to arrange adequate interim wet storage. It would of course be necessary to ensure that any long-term conservation on-site was undertaken in an informed manner and with proper care.
When long-term conservation is to be postponed, excavators and site conservators may wish to consider interim storage in ethanol rather than in water. Ethanol would avoid the risks of algal and microbial growth, would arrest aqueous attack, and would probably be a reversible process. The possible fire hazard associated with the transport and storage of baths of ethanol would, of course, have to be considered.
4 THE CONSERVATION OF THE BEDFORD GLASS ASSEMBLAGE
4.1 CONSERVATION BACK-GROUND
At the time of conserving the Bedford glass, the approach of dewatering with a hydrophylic solvent followed by the application of a consolidant system was already established (Newton and Davison 1989). The use of Paraloid B-72 dissolved in toluene as a consolidant system for excavated glass had been suggested by Newton and Davison (1989), and this method is still in use today (Johnson 2001). The novel aspect of the method reported here was the use of the mixed ethanol-acetone solvent system for the Paraloid B-72 (Davison 1994).
4.2 INITIAL WASHING
As the first stage of the long-term conservation procedure reported in this article, the glass fragments were removed from between the foam and plastic of the temporary storage. Although the glass was all damp, it was not all wet in the sense of being surrounded by excess water. The fragments were therefore immersed in tap water to soften any attached soil deposits and facilitate removal of the visible areas of greenish, slimy organic growth. Washing prior to long-term storage was also important to dissolve any soluble salts that may have remained on the surface of the glass or in cracks or that may have accumulated due to ion exchange following the original desalination. (In general terms it may be noted that some form of equilibrium state may have been approached over the long term during burial but that the drastic change in immediate environment occasioned by excavation may result in rapid reactions under the new environment.) The preconservation washing was also employed to dilute and remove any localized volumes of highly alkaline solution that may have formed in the small volumes of water surrounding most of the individual pieces during temporary storage.
The fragments were weighed, drawn, measured for thickness, and examined under a binocular microscope for condition and traces of paint or staining. The pieces were kept wet during the recording process by repeated immersion in the tap water. The fragments were then placed individually on a piece of finely woven nylon netting. The netting allowed the pieces to be suspended in the solution so that all surfaces were in contact with the liquid. The netting also supported the fragments and facilitated moving them from one solution to another.
4.3 CHOICE OF MATERIALS
The overall rationale behind the treatment proposed in this article was to wash the glass in water to make it clean and then: (1) to replace the water in the friable parts of the glass samples with another liquid (one that would be miscible with a solution of a desirable consolidating polymer) without allowing the glass to become dry; (2) to introduce the consolidant in solution; and (3) to cause the consolidant to be precipitated within the friable glass structure as its solvent evaporated.
The consolidant chosen for this application was Paraloid B-72, reportedly a 70:30 molar poly(ethyl methacrylate/methyl acrylate) copolymer (Horie 1987, 106). It was chosen for its good yellowing resistance and particularly for the fact that it has a sufficiently high glass transition temperature (roughly speaking, a high enough softening temperature) to avoid the surface's being in any way sticky or likely to entrap extraneous particulate matter (dirt and dust) by the particles sinking into the surface. Many alternative consolidants, including some that can be prepared as water-based emulsions, have low glass transition temperatures that would lead to undesirable accretion of dirt and dust over the course of time.
As the chosen consolidant is not water soluble, it was necessary to select a dewatering liquid that would on the one hand be miscible with the water in the wet glass and on the other hand be miscible with a solvent suitable for dissolving and introducing the consolidant into the glass. The liquid chosen for the dewatering was ethanol.1
It is important for the water to be removed from the glass without allowing the pieces to become dry. The extent to which the ethanol will replace the water in the hydrated layer and so postpone shrinkage and new cracking is uncertain. Observations by Alten (1988) on highly deteriorated glass, which from her description was probably very similar to the highly deteriorated Bedford glass, suggest that no damage is done by immersing the glass in ethanol and that new cracking occurs only on air-drying, be that from a water bath or from an ethanol bath. A most important aspect in preserving the appearance of the wet glass is to prevent any access of air to any cracks, whether ancient or modern. In the approach proposed in this article, the water needs to be replaced with another liquid that will allow a dissolved polymer to be brought into the cracks and be precipitated there to maintain structural integrity and visual appearance. Simply drying the glass in air would cause air to enter cracks and voids in the glass, and this air would impede subsequent introduction of the consolidant. Air in the cracks would also make the cracks far more visible and might give the glass a frosted, cloudy, or iridescent appearance dissimilar to the original appearance of the glass. (The refractive index difference across a glass-air interface is far greater than that across a glass-liquid or glass-consolidant interface and is thus far more likely to result in reflections that make the cracks visible.) Acetone would have been an alternative to ethanol as a dewatering agent but might have evaporated too quickly in moving between solutions and so allowed air into cracks. Most workers would consider it less pleasant to work with acetone than with ethanol. (Acetone has a degreasing effect on the human skin and can cause headaches.)
The choice of solvent for the consolidant was a third consideration. Ethanol does dissolve Paraloid B-72 slightly but not sufficiently for it to be effective in a single introduction of consolidant. Acetone will dissolve far more of the chosen consolidant (well over 4 wt%) but tends to cause the consolidant to migrate to the surface of the piece being consolidated as the acetone evaporates. It can also sometimes leave a bloom on the surface. A mixture of these two solvents was tested on a small sample and then, after apparently satisfactory results, on all the glass.
The consolidant solution containing approximately 2 wt% of Paraloid B-72 in 50% acetone and 50% ethanol was produced. A 4 wt% solution of B-72 in acetone was first produced, and this mixture was diluted with an equal volume of ethanol to give the (approximately) 2 wt% solution. Paraloid B-72 will dissolve in ethanol to some extent but not at anywhere near the 2 wt% level. It can readily be observed in a beaker that any concentration of ethanol significantly higher than 50 vol% causes the polymer to start to precipitate out of solution. The 2 wt% solution was thus seen to be very close to saturation, a feature particularly desired for the reasons given below. It was this near saturation loading of consolidant that determined the particular consolidant concentration and the solvent ratio, but other ratios could be tested in future work.
Acetone was deliberately chosen as the primary solvent in the mixture because it would evaporate faster than the ethanol (see discussion in section 6 below). The ethanol will slow the evaporation of the acetone, inhibiting the tendency to form a surface bloom. The ethanol should also reduce the tendency for the consolidant to be carried to the surface during drying.
4.4 DEWATERING AND CONSOLIDATION
To effect the dewatering after the initial washing, examination, and recording of the glass, the fragments and netting were in the first instance immersed in a mixture of 50 vol% water and 50 vol% ethanol held in a glass container. This stage was designed to begin the removal of the water by displacing it with ethanol. A glass cover was placed over the container to retard evaporation. As a fume cupboard happened to be available, the container was kept in it in order to remove any traces of ethanol vapor from the workplace more effectively. The use of a fume cupboard is not necessary if the lid fits well or the container is kept in a well-ventilated place. After three days the pieces of glass and their supporting nylon netting were removed and placed into 100% ethanol, where they were left for a further three days. Occasional gentle agitation would minimize the possible development of concentration gradients. In addition to displacing the water, the ethanol would also kill any organic growth that may have remained on or within the fragments.
After three days in the 100% ethanol, the fragments were removed and placed into the consolidant solution described above. After three days in the consolidant solution, the fragments were removed, one by one, with a pair of plastic tongs. The fragments were immersed in a bath of 100% acetone for a few seconds to remove any excess Paraloid B-72 solution from the surface. The pieces were then allowed to dry in the fume cupboard, but a well-ventilated, relatively dust-free area would probably suffice. (The use of a solvent bag in order to slow evaporation did not appear necessary, further contributing to the ease of application of this conservation procedure.) After drying for a few days, the fragments were packed in individual resealable polyethylene bags, which were placed in storage boxes.
The fragments were kept in the dewatering and consolidant solutions for three days to ensure the penetration into cracks deep within the very deteriorated glass fragments, but this time could be shorter for less-deteriorated pieces. In practice, the dwell times of batches in the solutions allowed plenty of time for the next group to be documented.
5 RESULTS
Definitive evaluation of the success of any treatment is difficult when the treatment has not been subjected to detailed scientific investigation. On the basis of present evidence, this method is considered successful for a number of reasons. The pieces that were transparent before treatment remained so after treatment, although it should be noted that they were not highly deteriorated. The pieces that were highly degraded and prone to crumbling before treatment were strong and solid after treatment. No painted decoration was obscured, and in some cases, where the glass was very deteriorated, the darkness of the glass lightened, perhaps due to filling of microscopic cracks in the glass matrix, and the decoration previously unseen became visible as darker areas on the surface. These improvements were observed immediately after treatment and still applied when the stored fragments were checked five years after their treatment. Badly deteriorated pieces that were washed and air-dried but not consolidated (as a control and as material for potential future analysis) crumbled even as they were lifted into storage bags.
6 DISCUSSION
The use of the mixed solvent for consolidating the dewatered glass is a novel (Davison 1994) and apparently successful approach to conserving waterlogged medieval window glass, but the exact mechanism of its action has yet to be determined. Several ideas as to why the mixed solvent produced good results may be suggested, although they are still a matter of speculation until more research is undertaken.
One speculation is this: As the consolidant solution starts to diffuse into the ethanol-saturated glass cracks, it will soon reach an ethanol concentration at which the consolidant starts to precipitate. As more consolidant solution diffuses into the cracks, the consolidant may be partially or wholly redissolved with the “precipitation front” moving deeper into the glass pore structure. Depending on time of immersion in the consolidant solution, some precipitated consolidant may already be present within the glass fragments when they are removed from the consolidant solution and the excess consolidant solution is rinsed off the surface.
Solvent evaporation may be expected to begin with the acetone component starting to evaporate preferentially at a solution front just beneath the surface. As soon as the acetone concentration in the solution drops, the consolidant will start to be precipitated. Although evaporation of the acetone will occur from the surface of the solution, diffusion of the acetone from the consolidant solution within the glass cracks and pores should cause the consolidant to be precipitated wherever the solution has penetrated. Acetone will diffuse from within the glass through the solution and the precipitated polymer, but its concentration may not be high enough to carry the consolidant with it to the surface. The decreasing consolidant solubility in the increasingly ethanolrich solvent that will be created during drying should reduce the tendency for the consolidant to be carried to the surface of the object, as might occur if a single solvent such as acetone or toluene had been used. At present this mechanism and its desired effect are unverified hypotheses, but further research in this area will be undertaken in the near future.
The end result of consolidation with the mixed solvent system may thus be that both impregnation and evaporation stages favor the deposition of consolidant at some depth within the crack and pore structure of the glass. The end results of the procedure seem sufficiently promising to be worth presenting for others to consider and investigate further.
6.1 CAUTIONARY NOTE
Despite the good showing in this initial trial, it is clearly necessary to exercise caution before applying a procedure that has been tested on only one occasion on one assemblage of glass. Particular caution might be necessary in the case of crizzling glasses that have thick hydrated layers on the surface. These layers lose water, shrink, and crack when exposed to low relative humidity. Simply observing that cracks appear on the surface of a glass as it dries does not of itself indicate whether these are pre-existing cracks revealed by the loss of the liquid that previously filled them, cracks created by shrinkage of a hydrated layer or a combination of both. It is not clear to what extent water in a fairly thick initially hydrated layer might be replaced by ethanol or acetone, but such a replacement is at least plausible. It is, however, less likely that a hydrated layer could be penetrated by a large polymer in solution. It might therefore be expected that a hydrated layer would shrink or crack as the water, alcohol, and/or acetone evaporated from it. Nevertheless, the presence of a polymer precipitating around the hydrated layer as it shrank might inhibit the appearance and growth of cracks by filling them as they develop. For the present, this sequence of events is pure speculation, and this method should be carefully assessed before risking any application to crizzling glasses. The possibility of crizzling appearing slowly over the course of time from stresses in the formerly hydrated layer should also be considered.
7 CONCLUSIONS
This method was relatively inexpensive; it had minimum health and safety risks (ethanol and acetone are less toxic than toluene); it could be performed quickly; and it appears to have produced a very satisfactory result on the assemblage of glass to which it was applied, some of which was badly deteriorated. The method can be used where only basic chemical supplies and simple equipment are available. Under some circumstances, this method could be applied on-site: doing so would overcome the problems of interim wet storage and obviate the need for postexcavation conservation prior to study, recording, and storage. It is of course necessary to ensure that long-term conservation on-site is undertaken with proper care rather than in haste as though it were an interim measure. The need to conduct preliminary tests and to exercise caution before applying a novel procedure is emphasized.
NOTES
1. As the work was done in Britain and funds were limited, industrial methylated spirits (IMS) were in fact used instead of ethanol. IMS is composed primarily of ethanol, and it is not thought that its use made any significant difference to the process. The word “ethanol” has been used in the text in the interests of general comprehension. Pure ethanol can be difficult to obtain or prohibitively expensive because of taxation by national governments.
REFERENCES
Alten, H. I.1988. Changes in waterlogged medieval window glass. Materials Research Society Symposium Proceedings123:279–84.
Brill, R. H.1975. Crizzling: A problem in glass conservation. In Conservation in archaeology and the applied arts: Preprints. Contributions to the Stockhom Congress, June 2–6. London: International Institute for the Conservation of Historic and Artistic Works. 121–31.
Davison, S.1994. Personal communication. Formerly at Institute of Archaeology, University College London, U.K.
Hench, L. L., and D. E.Clark. 1978. Physical chemistry of glass surfaces. Journal Non-Crystalline Solids28:83–105.
Horie, C. V.1987. Materials for conservation. London: Butterworths.
Johnson, R.2001. Personal communication. Museum of London, London, U.K.
Metcalfe, A. G., M. E.Gulden, and G. K.Schmidt. 1971. Spontaneous cracking of glass filaments. Glass Technology12:15–23.
Newton, R. G.1969. Some further observations on the weathering crusts on ancient glasses. Glass Technology10:40–42.
Newton, R. G., and S.Davison. 1989. Conservation of glass.London: Butterworths.
Pollard, A. M., and C.Heron. 1996. Archaeological chemistry. Cambridge: Royal Society of Chemistry.
Raw, F.1955. The long-continued action of water on window-glass: Weathering of the medieval glass of Weoley Castle, Birmingham. Journal of the Society of Glass Technology39:128T–33T.
Weyl, W. A., and E. C.Marboe. 1962–67. The constitution of glasses: A dynamic interpretation, Vols. 1, 2 pt. I, and 2 pt. II. New York: Interscience.
AUTHOR INFORMATION
DAFYDD GRIFFITHS, B.Sc., Ph.D., has been a lecturer at the Institute of Archaeology, University College London (UCL), since 1985. He works on the physical and chemical analysis of inorganic materials (particularly ceramics and glass) with a view to understanding the processes of their decay, the technology of their manufacture, and their provenance. He is interested in developing improved methods for conserving the information content of artifacts. Address: Institute of Archaeology, University College London, 31-34 Gordon Square, London WC1H 0PY, U.K.
ANN FEUERBACH took an M.A. in fine arts before reading for her B.Sc. in archaeological conservation and M.Sc. in archaeometallurgy at the Institute of Archaeology, University College London. She is currently reading for a doctorate at UCL on the manufacture and use of crucible steel in Central Asia during the early Islamic period. Address as for Griffiths.
Section Index |