CELLULOLYTIC BIODEGRADATION OF COTTON FIBERS FROM A DEEP-OCEAN ENVIRONMENT
RUNYING CHEN, & KATHRYN A. JAKES
ABSTRACT—Items of clothing recovered after 133 years of submersion at a deep-ocean shipwreck site provided a unique source of marine-degraded textiles. In this research, both dyed and undyed cotton samples taken from a man's waistcoat were studied by optical and scanning electron microscopy and by energy dispersive x-ray spectroscopy. The undyed fibers showed features typical of localized biodegradation, and two different forms of cellulolytic micro-organisms were observed. Black deposits, perhaps formed by sulfate-reducing bacteria, were observed on both the dyed and undyed samples. After treatment with a mixture of sodium hydroxide plus carbon disulfide, fibrillation and horizontal fragmentation of the dyed and undyed cotton fibers, respectively, were observed. In contrast, new cotton samples treated similarly with sodium hydroxide and carbon disulfide formed the “balloons” typical of fibers with intact primary walls. Tin was present only in the dyed sample. Its source is probably a mordant used in the dyeing process. It is likely that the tin aided in protecting the fabric from attack by cellulolytic micro-organisms.
TITRE—La biod�gradation cellulolytique des fibres de coton r�cup�r�es d'une �pave marine en grande profondeur. R�SUM�—Des morceaux de v�tements r�cup�r�s apr�s 133 ans d'immersion dans une �pave en grande profondeur ont fourni une occasion unique d'�tudier les dommages caus�s sur les textiles dans un environnement marin. Durant la recherche, des �chantillons de coton �cru et d'autres qui avaient �t� teints ont �t� pr�lev�s d'un gilet d'homme et �tudi�s au microscope �lectronique optique et � balayage, ainsi que par la spectrom�trie de fluorescence des rayons X � dispersion d'�nergie. Des dommages typiques de biod�gradation localis�e et deux diff�rentes formes de micro-organismes cellulolytiques ont �t� observ�s sur les fibres �crues. Des d�p�ts noirs, probablement form�s par des bact�ries ana�robies, ont �t� observ�s sur les deux types d'�chantillons. Apr�s traitement avec un m�lange d'hydroxyde de sodium et de bisulfure de carbone, on a observ� une fibrillation sur les �chantillons de coton teint et une fragmentation horizontale des fibres de coton �cru. Comparativement, de nouveaux �chantillons de coton qui avaient �t� trait�s de la m�me fa�on avec l'hydroxyde de sodium et le bisulfure de carbone ont form� les "bulles" typiques qui indiquent des fibres avec des parois cellulaires intactes. L'�tain, qui a probablement servi de mordant pour la teinture, �tait pr�sent seulement dans les �chantillons teints. Il est probable que la pr�sence d'�tain ait jou� un r�le dans la protection du tissu contre l'attaque par les micro-organismes cellulolytiques.
TITULO—Biodegradaci�n celulol�tica de fibras de algod�n provenientes del fondo oce�nico. RESUMEN—Restos de vestimentas recuperados luego de estar sumergidos 133 a�os en el sitio de un naufragio en el fondo oce�nico proporcionaron una fuente incomparable de textiles degradados por el mar. En esta investigaci�n se estudiaron muestras de algod�n te�ido y sin te�ir de un chaleco masculino por microscop�a �ptica y de barrido electr�nico y micro sonda dispersiva de rayos X. Las fibras sin te�ir mostraron caracter�sticas t�picas debiodegradaci�n localizada y se observaron dos formas diferentes de microorganismos celulol�ticos. Tanto las muestras te�idas cuanto las sin te�ir mostraron dep�sitos negros, formados quiz�s por bacterias reductoras de sulfato. Luego del tratamiento con una mezcla de hidr�xido de sodio y disulfuro de carbono, las muestras te�idas y sin te�ir mostraron fibrilaci�n y fragmentaci�n horizontal, respectivamente. Por el contrario, muestras de algod�n nuevas tratadas en forma similar con hidr�xido de sodio y disulfuro de carbono presentaron "globos" caracter�sticos de fibras con paredes primarias intactas. Se detect� esta�o s�lo en la muestra te�ida. Su fuente probablemente sea un mordiente utilizado en el proceso de te�ido. Es probable que el esta�o haya contribu�do a proteger al tejido del ataque de microorganismos celulol�ticos.
1 INTRODUCTION
1.1 THE SS CENTRAL AMERICA SHIPWRECK
The trunk of textiles that provided materials for this research project belongs to the shipwreck of the SS Central America, a passenger steamship that sank in the Atlantic Ocean in 1857 (Herdendorf et al. 1995). For 130 years, the location of the SS Central America remained unknown, but in 1987 the Columbus-America Discovery Group, Columbus, Ohio, successfully located the ship using modern deep-ocean exploration technologies. The organization then conducted a systematic investigation of the shipwreck, assisted by members of an affiliated Adjunct Science and Education Program who aided in the study of the deep-ocean environment of the site.
The shipwreck lies at a depth of 2,200 m in a mainly foraminiferal ooze comprised of shells of numerous small sea animals. The water at this depth is influenced by the North Atlantic deepwater flow. It is cold, oxygen-rich, and less saline than surface water (Herdendorf et al. 1995). Specific conditions of seawater appropriate to this depth include: pressure, 220 atmospheres; dissolved oxygen, 5.85–6.40 ml/l; temperature, 2.81–3.77oC; salinity, 34.9–35.06%; and pH, 7.99–8.14 (Herdendorf et al. 1995).
1.2 THE RETRIEVED TRUNK AND ITS TEXTILES
Of the several pieces of luggage located on the ocean floor near the shipwreck, only two were retrieved. The first, raised in 1990, belonged to a newly married couple named Easton and was the source of the fabrics used in this study. The trunk remained under water in transport to Ohio and was refrigerated until unpacking some four weeks later.
Each item in the trunk was unpacked, examined, flash-frozen, and slowly dried prior to initiation of the work reported herein (Jakes and Mitchell 1992). Items of clothing removed from the trunk smelled sulfurous, were covered by areas of black deposits, and displayed variable states of preservation.
1.3 MARINE BACTERIA
Both aerobic and anaerobic bacteria are active in the marine environment. As dissolved oxygen decreases in deeper water or in the sediment, anaerobic bacteria dominate. Sulfate-reducing bacteria grow in the absence of oxygen by using sulfate as the terminal electron acceptor and releasing hydrogen sulfide (H2S) (Florian 1987b; Vetter et al. 1989). Hydrogen sulfide reacts with organic materials in the sediment to produce thiol compounds and with certain metal ions to form the corresponding metal sulfide. One example is the black ferrous sulfide deposited on marine artifacts formed by the reaction of H2S with iron oxide. Sulfate-reducing bacteria also produce sulfuric acid, which promotes iron corrosion, e.g., corrosion of the metal components of the wrecked ship (Herdendorf et al. 1995).
1.4 STUDIES OF MARINE TEXTILE ARTIFACTS
The textiles from the trunks recovered from the SS Central America shipwreck site have been studied since 1990 by Jakes and others of the Department of Consumer and Textile Sciences at Ohio State University (Wang 1992; Foreman and Jakes 1993; Jakes and Wang 1993; Crawford 1994; Hannel 1994; Wang and Jakes 1994; Srinivasan and Jakes 1997; Chen 1998). Since the initial assessment and drying of the materials, fibers taken from different textile items have been studied by optical microscopy, scanning electron microscopy, x-ray microanalysis, x-ray diffraction, and infrared microspectroscopy. Flax, cotton, wool, and silk were identified through their typical morphological features and also by infrared spectroscopy. Changes in the morphology are noted as indicators of degradation. For example, disruptions of cotton fiber morphology, which are evidence of degradation, include bulges (i.e., localized swollen areas whether due to expansion of the secondary wall or of the lumen); cracks (i.e., fissures horizontal to the length of the fiber); and fibrillation (i.e., longitudinal splitting along the length of the fibrils (Hearle et al. 1989; Jakes and Wang 1993; Wang and Jakes 1994; Peacock 1996b). Dark deposits, found both on the fiber surfaces and inside some fibers' lumina, contained iron and sulfur predominantly (Jakes and Wang 1993). A geochemist examined samples of this black material, recovered from within the Easton trunk, and reported finding both pyrite and geothite. The sample was a “mixture of oxidized, slightly oxidized, and reduced iron” and the material indicated that it had been “sitting close to the redox boundary” (Robbins 1996). To study the effect of the marine environment on textiles, an experiment was initiated in which standard textiles were immersed on the deep-ocean floor near the shipwreck for later retrieval (Wang 1992; Wang and Jakes 1994). These textiles were placed in the debris field of the wreck within the distance that other items of luggage were strewn out from the location of the ship itself.
Apart from those related to the SS Central America, only a limited number of marine textile studies have been reported in the literature. In general, these reports focus on a method of conservation, such as cleaning, drying, and storage;rarely is the degradation process explored. The conservation of wet organic artifacts, including textiles, was reviewed by Jenssen(1987). The fabric structure of the sails recovered from the Wasa and the treatment employed in their conservation was described (Bengtsson 1975a, 1975b). The textiles from the Mary Rose were examined by scanning electron microscopy (Ryder 1984). In the case of the Defence, linen, hemp, and silk fibers from the textiles were identified (Morris and Seifert 1978). Recognizing the need to study the degradation process of organic artifacts (other than wood) in the marine environment, Florian (1987a) outlined the chemical and physical structural changes that are possible.
1.5 CELLULOSE BIODEGRADATION
The decomposition of cellulose by bacteria and fungi occurs readily in damp, warm environments, resulting in material loss and deterioration of performance. A detailed summary of the bacteria and fungi associated with the biodegradation of cotton is reported in Siu (1951). Hearle et al. (1989) present micrographs displaying the morphological changes that result from degradation. Peacock (1996a, 1996b) describes cellulosic textiles in studies of biodegradation including burial experiments. Biodegradation of cellulose by marine fungi requires the presence of oxygen, so it is limited to oxygenated seawater and does not proceed within the anoxic sediment, while bacterial degradation of cellulose can proceed not only in seawater but also within the sediment to a depth of approximately 60 cm (Florian 1987a).
Micro-organisms are classified by the temperature range at which they reach optimum activity. Thermophilic bacteria and fungi grow fast in the temperature range 45–70�C, while mesophiles grow readily in the range of 25–45�C, and psychrophiles grow at temperatures around 10�C (Siu 1951). The bacteria described in the literature concerning cotton textile degradation nearly all fall into the mesophilic type, but bacterial cultures grown from water samples from the second trunk removed from the site of the SS Central America contained obligate psychrophiles that were short, gram negative rods (Herdendorf et al. 1995).
Most cellulose-decomposing bacteria are most active around pH 7.0–8.0, while fungi thrive in acidic conditions. Light also affects the activity of bacteria and fungi. Some grow more quickly in the dark. Nutrients required include carbon, nitrogen, phosphorus, magnesium, sulfur, and trace elements. Sulfur is often supplied in the form of sulfate; cellulolytic fungi require 0.1 g/l sulfate to attain optimum cellulose-decomposing activity (Siu 1951). Thus sulfate reduction and cellulose degradation may be accomplished by the same bacteria.
1.6 CHARACTERISTICS OF CELLULOSE FIBERS DAMAGED BY BACTERIA AND FUNGI
Microbial attack on cotton fibers begins with digestion of the outer cuticular layer (Siu 1951; Heyn 1954; Peacock 1996b). A consequence of this phenomenon can be observed microscopically by treating the damaged fibers with a strong swelling agent, such as cuprammonium hydroxide or a mixture of alkali and carbon disulfide. Swelling of the secondary wall of normal cotton, restricted by the primary wall, which does not swell, results in the formation of “balloons” (Heyn 1954; Merkel 1984). Ballooning occurs along normal cotton fibers but not on fibers damaged by bacteria and fungi. Further, the histology of bacterial and fungal attack on cellulose fibers differs. In general, bacterial attack on cotton fibers proceeds from the outer layer of the fiber without wall penetration, while fungal hyphae often penetrate into the fiber wall, resulting in the production of small fiber fragments. Crystalline areas of cellulosic fibers are less accessible to attack by cellulolytic enzymes released by bacteria and fungi (Kr�ssig 1993). A prior swelling treatment can increase the number of accessible regions within a fiber and thereby increase biodegradability.
1.7 OBJECTIVES AND RESEARCH QUESTIONS OF THIS STUDY
The items of clothing recovered from the Easton trunk were intact and contiguous garments. A man's waistcoat (inventory no. 29178), selected for this research, was constructed with three fabrics: a blend of silk and cotton for front portions, a dark brown–colored dyed cotton twill for the back, and undyed plain cotton fabric for the lining. The condition of the outer portions of the garment was generally good, although random areas were covered with black or brown stains and deposits. The lining was more obviously damaged, with torn fragmented areas covered with black deposits. The black material readily flaked from the surface of the lining onto anything it touched. When samples were taken from the waistcoat, the brown-colored waistcoat back fabric remained intact, while the undyed lining broke into fragments. The objective of this study was to investigate why and how the two cotton fabrics from the same object and same environment exhibited differences in degradation. The fabrics' degradation was evaluated in three ways: (1) the morphological differences among specimens of the dyed and undyed samples before and after swelling treatments; (2) the elemental composition differences of specimens from the two fiber samples and of the deposits on their surfaces; (3) the evidence for biological degradation of the specimens based on their reactivity. Evaluation of the degradation of the same materials through examination of the infrared spectra of single fibers is described in Chen and Jakes (2000).
2 RESEARCH METHODS
2.1 SAMPLES
The dyed cotton sample was composed of three pieces measuring approximately 15 x 5 mm each and was removed from inconspicuous seams inside the waistcoat. The sample of the undyed cotton lining was composed of three pieces measuring approximately 5 x 3 mm each. These samples are designated as historic dyed (MD) and historic undyed (MU) in the discussion and tables that follow.
For comparative purposes, two additional cotton fabrics were sampled and studied in conjunction with those from the marine environment. One new cotton sample (SD) was taken from a reference fabric (TestFabrics Company, no. 8588). The other sample was taken from the same reference fabric that had been immersed for three months at the same deep-ocean site where the trunk was retrieved. This sample was labeled immersed new sample (M3) for the purposes of the discussion.
Three sets of the fiber specimens from each of the four samples were mounted for examination with optical microscopy. One set of fiber specimens (72–85 individual fibers) obtained from each of the four fabric samples was mounted on slides in Permount (table 1). A second set of fiber specimens (69–116 individual fibers) from each of the four samples was mounted on microscope slides with 18% (w/v) sodium hydroxide and observed and measured after five minutes. A third set of specimens was prepared by treating yarns from each of the four samples with a mixture of equal volumes of aqueous 18% sodium hydroxide (NaOH) and carbon disulfide (CS2) for 30 minutes. At least 30 individual fiber specimens were removed from these treated pieces and mounted on slides for examination.
2.2 OPTICAL MICROSCOPY
A Zeiss Axioplan research microscope with differential interference contrast (DIC), phase contrast, and polarized illumination capabilities was used at nominal magnifications of 200x, 400x, and 1000x. Pictures of the fibers were taken with a MC 100 camera. Fiber width was measured at 100x using a Zeiss Axioskop coupled with an Optimas 5.2 image analysis system.
Table .
Fiber Width measurements and Tukey's Pairwise Comparisons
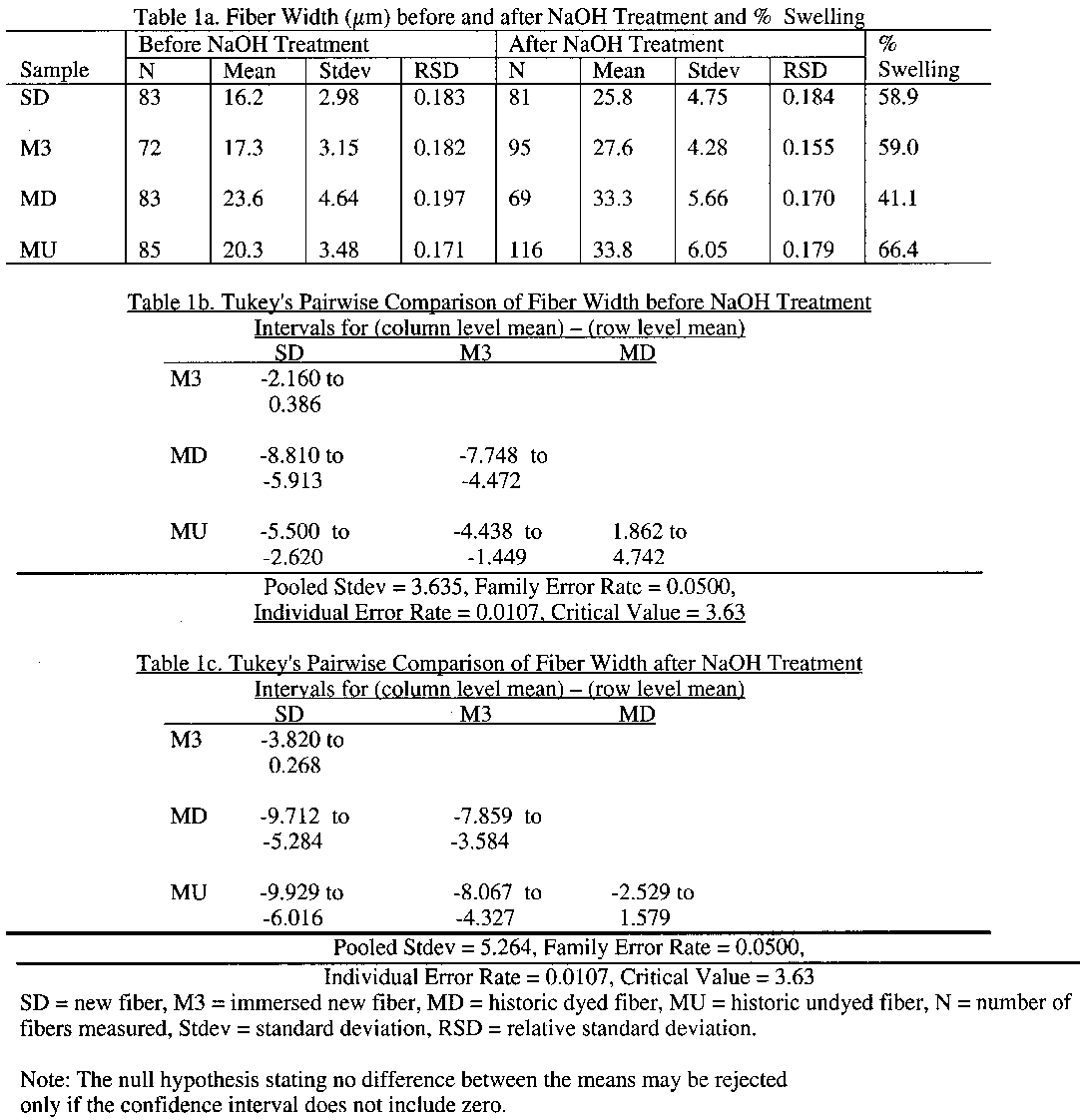 |
2.3 SCANNING ELECTRON MICROSCOPY (SEM)
A Philips XL30 equipped with a Be-window, a Tracor Northern TN-5500 Micro-Z analyzer, a VISTA SQ computer image analysis system, and a 20 keV accelerating voltage were employed in this work. In addition to micrographs taken with Polaroid type 55 film, the SEM images were digitized for analysis using Adobe Photoshop 5.0 software.
Fifteen fiber specimens from each of the four samples were mounted on carbon planchets with double-side carbon tape and coated with gold and palladium using a Denton Vacuum Desk II sputter coater.
Fig. 1.
Optical micrographs of historic fibers (DIC, 400x): a, historic dyed cotton; b, historic undyed cotton
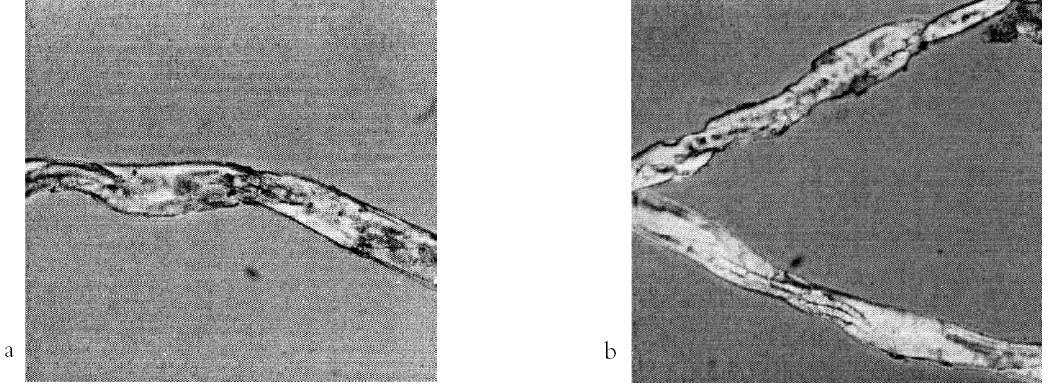 |
Fig. 2.
Optical micrographs of historic undyed fiber after 15 minutes in 18% NaOH (400x): a, intact and degraded fiber segment; b, degraded fibers
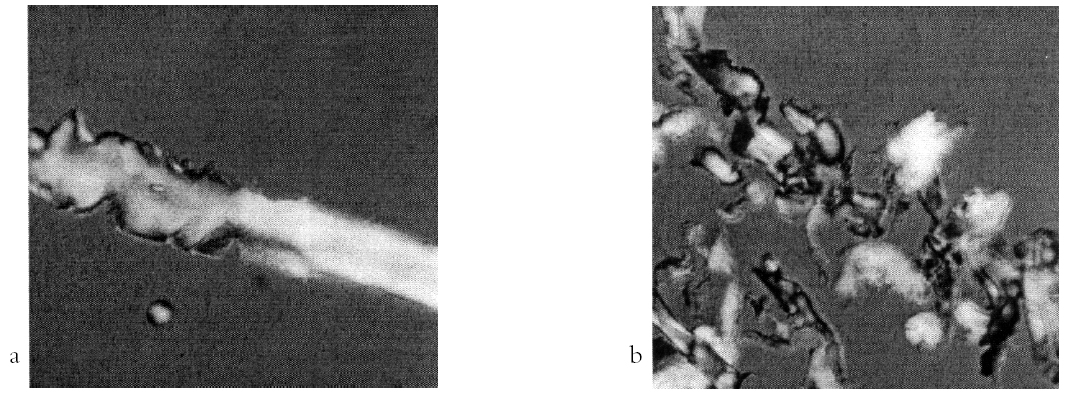 |
2.4 ENERGY DISPERSIVE X-RAY SPECTROSCOPY (EDS)
An Oxford Instruments Group Link Analytical eXL with a Pentafet detector, coupled with a JEOL JSM 820 SEM and a conventional Be window, was used for elemental analysis. Fifteen fibers from each of the four samples were mounted on carbon planchets and coated with carbon. After qualitative analysis and calibration with a copper standard, quantitative elemental analysis was performed on each of the 15 fibers. With x-ray spectra collected for 100 seconds of live-time from fiber areas under 1,000x magnification and using an acclerating voltage of 20 keV, an average elemental composition of the entire sampling area was obtained. After acquisition of the spectra, the percentage of each significant element in the spectra was normalized by dropping the elements with x-ray counts below the instrument detection limit. Because the mean percent elemental composition of each element is calculated only for the heavy elements, and the quantity of carbon, oxygen, and other light elements is unknown, the percent elemental composition that was determined was employed only to indicate relative predominance of elements present in the samples.
3 RESULTS AND DISCUSSION
3.1 OPTICAL MICROSCOPY
No morphological differences between the specimens from the new and immersed new samples were observed prior to swelling treatment, with the exception of some small particulate deposits noted on the immersed fibers. Most of the historic dyed fibers displayed distinctly large fiber widths and a flat, convoluted, ribbon like appearance, characteristic of a coarse cotton species or of immature cotton fibers. Dark deposits were observed both on the surfaces of the fibers and inside the fibers' lumina, and some encrustation of the fiber surface was also seen. Overall, the historic dyed fibers displayed structures typical of cotton, with no obvious disruptions of morphology (figs. 1a, 1b). In contrast, morphological features resulting from biodegradation were observed in a large proportion of historic undyed fiber specimens. Both intact and degraded sections typical of the localized degradation caused by micro-organisms were observed.
Fig. 3.
Optical micrographs of fibers after 30 minutes in mixed solution of sodium hydroxide and carbon disulfide (DIC, 400x): a, new fiber; b, immersed new fiber; c, historic dyed fiber; d, historic undyed fiber
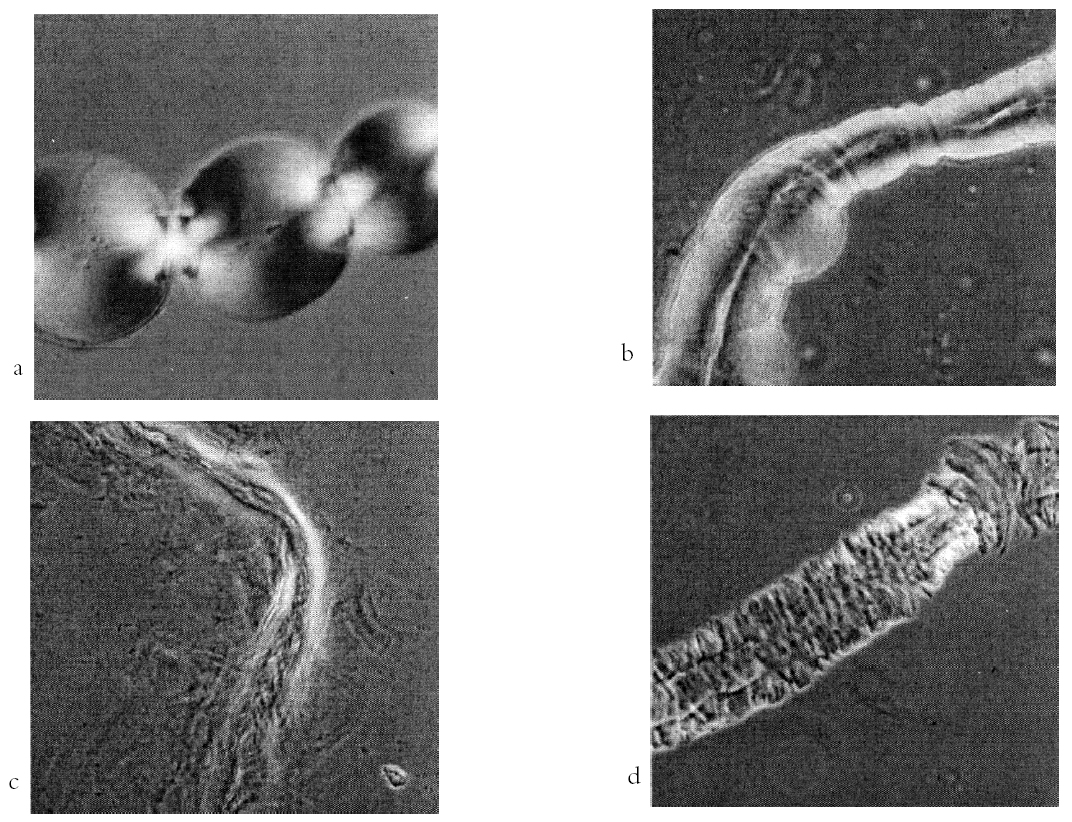 |
After treatment with 18% sodium hydroxide, fibers from the new fabric sample appeared similar to typical mercerized cotton. They were smooth and rodlike without convolutions or lumina or with very small lumina. In comparison, the immersed new fibers contained a higher proportion of fibers with medium to small lumina. Particulate deposits were observed on some of the fibers as well, both on their surfaces and in their lumina.
Treated with 18% NaOH (figs. 2a, 2b), the historic undyed fibers displayed more short fragments with more distinctive horizontal cracks in comparison to the fibers without treatment. The appearance of the historic undyed fibers suggests that they were severely biodegraded by micro-organisms. The degradation was localized, resulting in alternating fragments of intact and degraded fibers. The intact fragments are very similar in appearance to both the new and immersed new fibers after treatment with NaOH.
The mean fiber width of the historic dyed cotton (23.6 �m) and the historic undyed cotton (20.3 �m) were found to be significantly larger than those of new cotton (16.2 �m) and the immersed new cotton (17.3 �m), as supported by Tukey's pairwise comparisons (a=0.05) (Toothaker 1993). Table 1 includes the confidence intervals for differences between group means provided by the Tukey analysis. The mean fiber widths of the historic dyed and historic undyed cottons were different, but those of both the new and the immersed new fibers was not significantly different from each other.
The degree of fiber swelling, i.e., the % difference in fiber width before and after NaOH treatment, of both the new (58.9%) and the immersed new fibers (59.0%), was not significantly different (see table 1). The degree of swelling of specimens of the historic dyed sample (41.1%) was much smaller than that of the other three samples, whereas that of the historic undyed sample was highest of them all (66.4%). These results demonstrate that not only were the physical responses to NaOH treatment of the two new samples different from those of the two historic samples, but also that the two historic samples differed greatly from each other.
Fig. 4.
Scanning electron micrographs of historic dyed fiber: a, fiber with surface deposit (1,500x); b, surface deposit (15,000x)
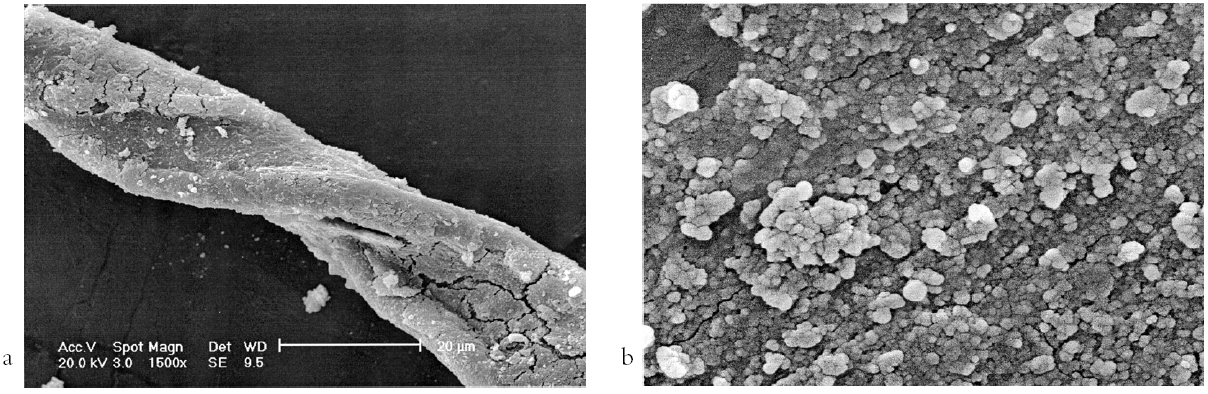 |
Treatment with a mixture of NaOH and CS2 was intended to detect the nature of degradation that had occurred in the fibers since balloons will appear along treated cotton fibers with an intact primary wall. This phenomenon was observed on most of the new fibers but was not as predominant in the immersed new fibers (figs. 3a, 3b). Ballooning was not observed on the historic dyed and undyed fibers, indicating primary wall damage (figs. 3c, 3d).
Longitudinal fibrillation was observed in fiber specimens from all four samples that had been immersed in the NaOH and CS2 mixture. Horizontal fragmentation or splitting, characterized by the development of narrow strips with tapered tips (see fig. 3d), was also observed. Only a few instances of this horizontal splitting were seen in the new and the immersed new fibers relative to the number observed in the historic dyed fibers and the historic undyed fibers. Fibers from the latter sample displayed the largest number of instances of this feature. One possible explanation for horizontal splitting is surface folding caused by a high degree of fiber swelling along the lateral direction, with shrinkage along the longitudinal direction.
3.2 SCANNING ELECTRON MICROSCOPY
The high resolution of the scanning electron microscope permitted fibers and particulate deposits on the scale of 50 nm to be examined (figs. 4a, 4b). Biodegradation, evident only in the historic undyed fibers, exposed the interior of the fiber secondary wall (figs. 5a, 5b). The size of the exposed interior area varied, and the exposed fiber interior structure indicated that the biodegradation process followed the fibril direction.
Within the exposed fiber interior, multiple grooves about 250 to 500 nm in width could be seen (see fig. 5b). Micro-organisms had digested the cellulose in the region of contact, completely removing material in this 200 nm wide area. The grooves within the exposed fiber interior were clean with no particulate deposit.
Figure 6 displays a fiber fragment on which two types of micro-organisms are observed. The rod-shaped micro-organisms are about 2 �m long and 200 nm wide, close to the dimensions of the grooves observed in exposed areas of the fiber interior. It appears that these rod-shaped micro-organisms have digested the cellulose molecules along the fibril direction, consistent with the characteristics of microbial degradation.
Oval-shaped micro-organisms also were observed on the historic undyed sample. Next to them are holes in the fiber of the same dimension, apparent in figure 6, indicating penetration of the primary wall. It is possible that the rod-shaped micro-organism was a type of deep-ocean cellulolytic bacteria, while the oval-shaped micro-organism was a deep-ocean cellulolytic fungi due to the mode of cellulolytic attack (Siu 1951); however, it is not known whether the rod-shaped organisms are the same as the ones cultured from the trunk water samples (Herdendorf et al. 1995). The fact that the organisms grew on the textiles at the site indicates that they are psychrophiles and grow well in the dark. If the oval-shaped organism is a fungus, then the conditions within the trunk must have provided some level of oxygen. Perhaps the environment changed through time, changing from an oxygenated system to an anoxic one. The presence of both iron sulfides and oxides suggests this explanation as well (Robbins 1996).
Fig. 5.
Scanning electron micrographs of historic undyed fiber: a, localized degradation (3,676x); b, the grooves formed by microbial degradation (10,000x)
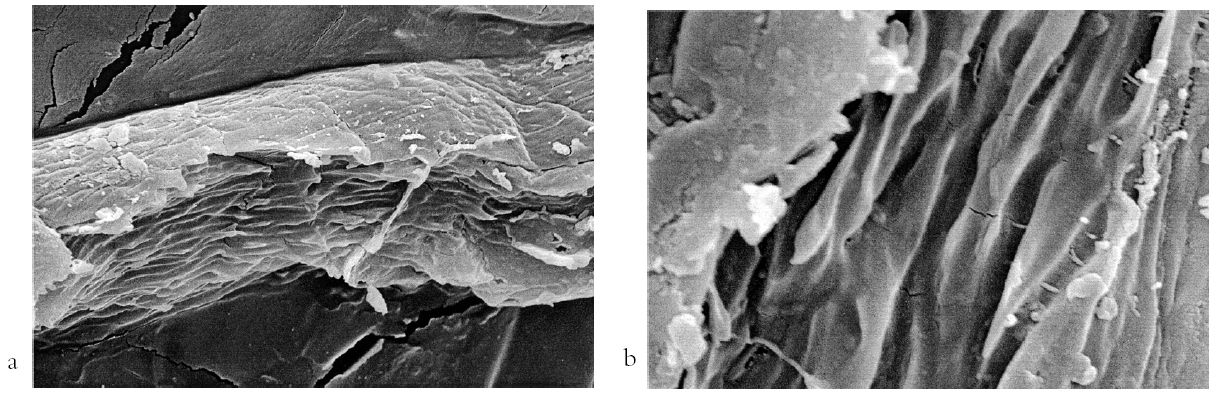 |
Fig. 6.
Scanning electron micrograph of historic undyed fiber with micro-organisms on the fiber surface (2,500x)
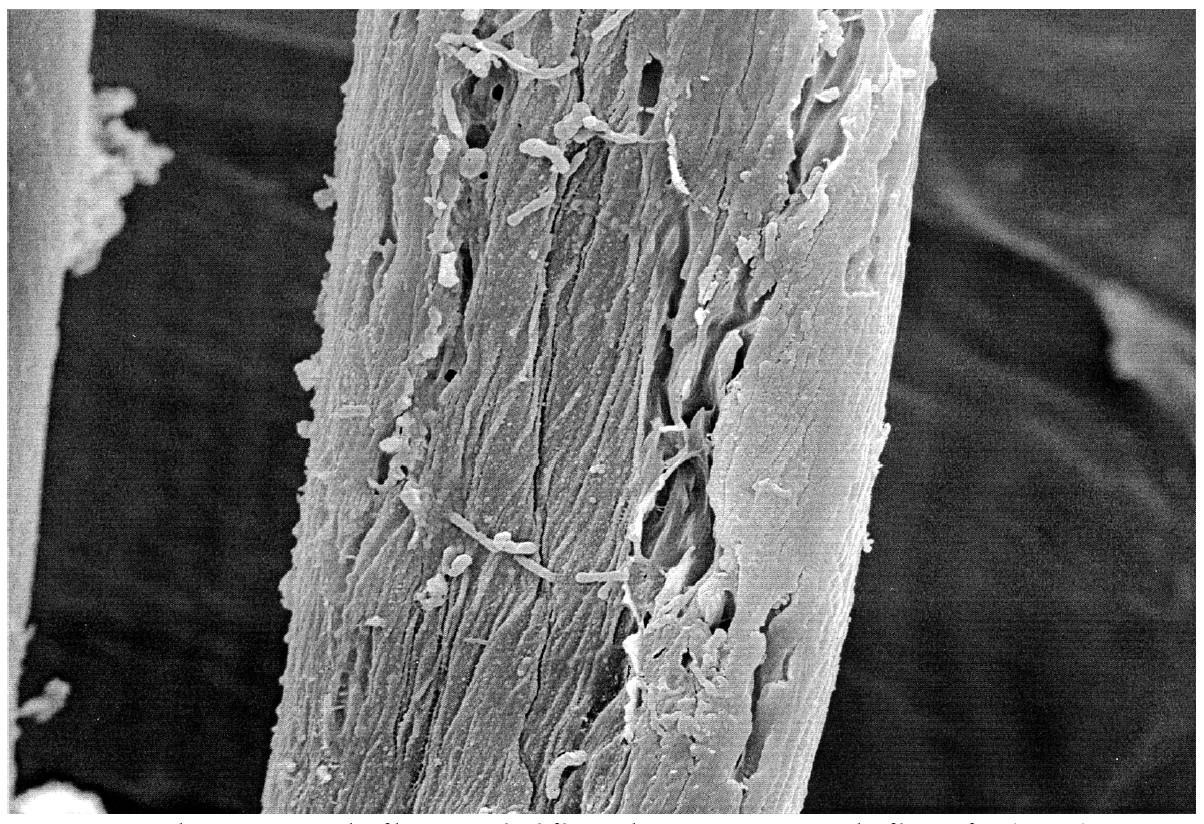 |
3.3 ENERGY DISPERSIVE SPECTROSCOPY ANALYSIS
Sodium and chlorine were the major elements present in the fibers of the three samples (table 2). The relative percentages of these, however, differ, with a combined total of about 86.3% in the immersed new sample; about 69.08% in the historic undyed sample, and 47.55% in the historic dyed sample. Because the percent composition that is calculated is a relative percent exclusive of elements lighter than sodium, the higher relative percentage of sodium and chlorine in the immersed new sample stems from the lower relative content of other heavy elements. Due to the short period of immersion, few deposits could form on the material's surfaces.
Table .
Fiber Elemental Compositions in Atom Percent(≥ Na)
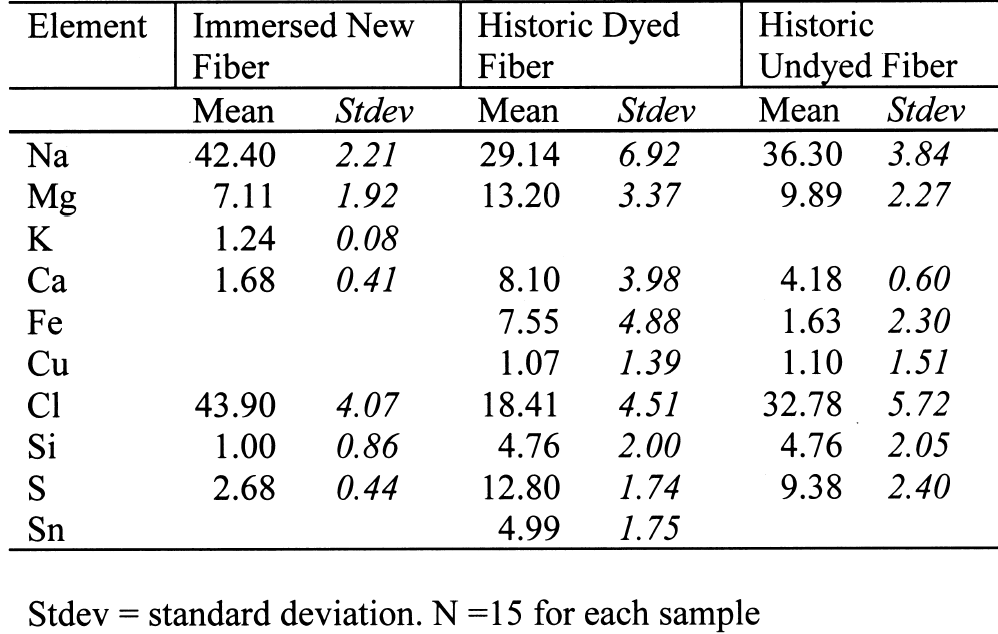 |
In both the historic dyed and undyed samples, the relative contents of sodium, magnesium, calcium, copper, chlorine, and silicon reflect the impact on the two fabrics of the accumulation of sediments and absorption of ions from the seawater. The small relative percentages of calcium and silicon in the immersed new sample compared to the two historic samples could reflect the short period of immersion and lack of accumulation of sediment. A smaller relative sulfur composition in the immersed samples may also reflect the lack of sufficient time or the appropriate conditions for sulfate reduction and sulfide deposition. It is likely that a longer period of time was required to produce the appropriate anoxic conditions for sulfate reduction.
As both the historic dyed and undyed samples were from the same environment and the same item of clothing, their elemental compositions were found to be similar to each other, with the exception of iron, sulfur, and tin. Just as the black deposits on the surfaces of the marine textiles (Jakes and Wang 1993; Wang and Jakes 1994) contained iron and sulfur, the presence of iron and sulfur within the historic fibers examined in this study is indicative of sulfate reduction occurring in the system established in the trunk. The unique presence of tin in the historic dyed sample probably indicates that some form of tin was used as a mordant in dyeing. Dyeing recipes for a dark brown color used around the 1850s included use of tree bark extracts with tin and iron mordants (Crooks 1874). Because the percentage of iron detected in the historic dyed sample (7.55%) was so much higher than that detected in the historic undyed sample (1.63%), some of the iron present may be due to a mordant treatment rather than all of it coming from a deep-ocean source.
According to Rouhi (1998), tin is toxic to bacteria and acts as a biocide agent in the marine environment. Therefore, it is highly possible that the tin contained in the dyed cotton fibers functioned as an inhibitor to the growth of marine micro-organisms and that the absence of the biocidal action of the tin in the undyed cotton fibers resulted in more severe biodegradation.
3.4 CONSERVATION IMPLICATIONS
In assessing a historic garment, the condition of the individual components must be considered. The biodegraded cotton lining in the waistcoat studied in this research looks more damaged than the dyed waistcoat back fabric, but the back fabric still shows a response to treatment different from that of a new textile. However, while the long-term storage in the deep-ocean environment affected the reactivity of the historic textiles, their prior condition and treatment influenced their reactivity as well. Thus the tin mordant limited the biodegradation of the dyed textile in comparison to the undyed textile.
Waterlogged textiles, whether from a marine site or some other wet site, will behave differently from materials that have never been stored wet. The long-term swollen state experienced by these materials might allow some molecular rearrangement even as microbial damage ensues. The textiles, returned to a terrestrial environment and dried, may still react in unexpected ways as they are treated. It is suggested that the observation of single fibers under the microscope while treating them in a planned protocol will allow the conservator to assess potential results.
4 CONCLUSIONS
The biodegradation incurred by the historic undyed sample was due to two types of cellulolytic micro-organisms in the deep-ocean environment in which the sample was submerged. One type was rod-shaped and attacked the cotton fibers layer by layer, creating an exposed fiber interior with characteristic grooves of about the same dimension as the rod-shaped micro-organisms. The other type was oval-shaped and penetrated the fiber wall, creating holes of about the same dimension as the organism. The presence of two types of microbial damage, as well as of iron sulfide deposits, reflected a combination of both anaerobic and aerobic conditions. The sequence in which these processes occurred remains to be determined.
The historic dyed sample fiber was less degraded than the undyed sample fiber, even though the two were from the same item of clothing and had experienced the same long-term storage environment. The growth of these cellulolytic micro-organisms was inhibited in the dyed cotton by the presence of a tin mordant that acted as a biocide.
Upon treatment with 18% NaOH, localized fragmentation was observed on the fibers removed in the historic undyed sample, a consequence of the localized microbial degradation. The higher degree of swelling of the historic undyed specimens demonstrated overall damage of the primary wall incurred by the undyed fiber specimens in comparison with the dyed specimens. Fibrillation was observed in both the dyed and undyed samples that were treated with a mixture of sodium hydroxide and carbon disulfide, as opposed to the balloons observed in the new samples indicating that both historic materials experienced some damage to the primary wall. One unique feature more frequently observed in the historic undyed samples was horizontal surface fragmentation or splitting. As conservators work with marine textiles, they must be aware that the environmental conditions and the types of degradation are different from terrestrial materials, and these differences may influence treatment decisions.
ACKNOWLEDGEMENTS
The authors gratefully acknowledge the helpful suggestions of the reviewers of the manuscript. The authors wish to thank Dr. John C. Mitchell at Ohio State University for his assistance in EDS data collection and analysis.
REFERENCES
Bengtsson, S.1975a. Preservation of the Wasa sails. In Conservation in archaeology and the applied arts: Preprints. Contributions to the Stockholm Congress, June 2–6. London: International Institute for the Conservation of Historic and Artistic Works. 33–35.
Bengtsson, S.1975b. The sails of the Wasa. International Journal of Nautical Archaeology and Underwater Exploration4:27–41.
Chen, R.1998. A study of cotton fibers recovered from a marine environment. Ph.D. diss., Ohio State University, Columbus.
Chen, R., and K. A.Jakes. 2000. Characterization of chemical and physical microstructure of historic fibers through microchemical reaction. In Historic textile and polymers in museums, ed. J. M.Cardamone and M. T.Baker. American Chemical Society Symposium series 779. Washington, D.C.: American Chemical Society. 55–73.
Crawford, L. C.1994. The analysis of mid-nineteenth century men's outer-garments from a deep ocean site. Ph.D. diss., Ohio State University, Columbus.
Crooks, W.1874. Dyeing and calico-printing. London: Longmans Green, and Co.
Florian, M-L. E.1987a. Deterioration of organic materials other than wood. In Conservation of marine archaeological objects, ed. D.Pearson. New York: Butter-worths. 21–54.
Florian, M-L. E.1987b. The underwater environment. In Conservation of marine archaeological objects, ed. D.Pearson. New York: Butterworths. 1–20.
Foreman, D. W., and K. A.Jakes. 1993. X-ray diffractometric measurement of microcrystallite size, unit cell dimensions, and crystallinity: Application to cellulosic marine textiles. Textile Research Journal63:455–64.
Hannel, S. L.1994. The acquisition of menswear in an American frontier town. M.A. thesis, Ohio State University, Columbus.
Hearle, J. W. S., B.Lomas, W. D.Cooke, and I. J.Duerden. 1989. Fibre failure and wear of materials. Chimchester: Ellis Horwood.
Herdendorf, C. E., T. G.Thompson, and R. D.Evans. 1995. Science on a deep-ocean shipwreck. Ohio Journal of Science95(4):4–224.
Heyn, A. N. J.1954. Fiber microscopy. New York: Inter-science Publishers.
Jakes, K. A., and J. C.Mitchell. 1992. The recovery and drying of textiles from a deep ocean historic shipwreck. Journal of American Institute for Conservation31:343–53.
Jakes, K. A., and W.Wang. 1993. The characteristics of textile fibers and fabrics recovered from a historic deep ocean site. Ars Textrina19:161–81.
Jenssen, V.1987. Conservation of the wet organic artefacts, excluding wood. In Conservation of marine archaeological objects, ed. D.Pearson. New York: Butter-worths. 122–63.
Kr�ssig, H. A.1993. Cellulose: Structure, accessibility and reactivity. Philadelphia: Gordon and Beach Science Publishers.
Merkel, R. S.1984. Methods for analyzing damage in textile materials. In Analytical methods for the textile laboratory, ed. J. W.Weaver. Research Triangle Park, N.C.: American Association for Textile Chemists and Colorists.
Morris, K., and B. L.Seifert. 1978. Conservation of leather and textiles from the Defence. Journal of American Institute for Conservation18:19–32.
Peacock, E. E.1996a. Biodegradation and characterization of water-degraded archaeological textiles created for conservation research. International Biodeterioration and Biodegradation36(1):49–59.
Peacock, E. E.1996b. Characterization and simulation of water-degraded archaeological textiles: A review. International Biodeterioration and Biodegradation36(1):35–47.
Robbins, E.1996. Personal communication. U.S. Geological Survey, Reston, Va.
Rouhi, A. M.1998. The squeeze on tributyltins. Chemical and Engineering News76(17):41–42.
Ryder, M. L.1984. Wool from textiles in the Mary Rose, a sixteenth-century English warship. Journal of Archaeological Science11:337–43.
Segal, L., and P. J.Wakelyn. 1985. Cotton fibers. In Fiber chemistry, ed. M.Lewin and E. M.Pearce. New York: Marcel Dekker. 809–908.
Siu, R. G. S.1951. Microbial decomposition of cellulose. New York: Reinhold Publishing Corporation.
Srinivasan, R., and K. A.Jakes. 1997. Examination of silk fibers from a deep-ocean site: SEM, EDS, DSC. In Materials issues in art and archaeology, vol. 5. Materials Research Society Symposium Proceedings 462, ed. P.Vandiver et al. Pittsburgh: Materials Research Society. 375–80.
Toothaker, L. E.1993. Multiple comparison procedures. Newbury Park, Calif.: Sage Publications.
Vetter, R. D., P. A.Murrai, B.Jacor, and J.O'Brien. 1989. Reduced sulfur compounds in the marine environment: Analysis by HPLC. In Biogenic sulfur in the environment, ed. E. S.Saltzman and W. J.Cooper. American Chemical Society Symposium series 393. Washington, D.C.: American Chemical Society. 243–80.
Wang, W.1992. Evaluation of the effect of the deep ocean environment on the physical and chemical characteristics of linen fabrics. M.A. thesis, Ohio State University, Columbus.
Wang, W., and K. A.Jakes. 1994. Evaluation of the degradation of cellulosic textiles in the deep ocean environment. Ars Textrina22:33–64.
SOURCES OF MATERIALSPermountFisher Scientific Co. 585 Alpha Drive Pittsburgh, Pa. 15238-2911 Cotton test fabricTestFabrics, Inc. 200 Blackford Ave. P.O. Box 420 Middlesex, N.J. 08846
AUTHOR INFORMATION
RUNYING CHEN holds a Ph.D. in textile science with a minor in analytical chemistry from Ohio State University. She is an assistant professor in apparel merchandising at East Carolina University, and she conducts research in analyzing textile materials via various analytical methods. Address: Department of Apparel, Merchandising and Interior Design, East Carolina University, 270A Rivers Building, Greenville, N.C. 27858-4353.
KATHRYN A. JAKES holds a Ph.D. in textile and polymer science from Clemson University. She is a professor in textiles at Ohio State University, and she conducts research in the identification and characterization of degraded polymeric materials. Much of her work deals with historic and archaeological textile materials. Address: Department of Consumer and Textile Sciences, Ohio State University, 245 Campbell Hall, 1787 Neil Ave., Columbus, Ohio 43210-1295.
Section Index |