PARALOID B-72 AS A STRUCTURAL ADHESIVE AND AS A BARRIER WITHIN STRUCTURAL ADHESIVE BONDS: EVALUATIONS OF STRENGTH AND REVERSIBILITY
JERRY PODANY, KATHLEEN M. GARLAND, WILLIAM R. FREEMAN, & JOE ROGERS
ABSTRACT—Acrylic copolymers are now well known in conservation practice. Based upon relative stability and reversibility, Paraloid B-72 has found a particularly wide range of uses as a coating, consolidant, and adhesive. Increasingly, B-72 is being used as an adhesive for reassembly of large stone sculpture. It has also found use as an interface barrier that, in theory, lends greater reversibility to joints made with less reversible adhesives such as epoxies or polyesters and limits the penetration of these materials into the adherend substrate. The following two studies were undertaken as an initial evaluation of these systems and to make a direct comparison between B-72 and the more commonly used structural adhesives, epoxy and polyester, from the standpoint of shear and tensile strength, as well as reversibility.Modified ASTM standard tests were used to determine the strength of marble-to-marble bonds formed with epoxy, polyester, and high-concentration solutions of B-72 in two commonly used solvents. Bonds made with epoxy or polyester adhesives including additional barriers of B-72 on both substrates of the adherends were also tested. The results indicate that in some solutions B-72 alone may be sufficiently strong under tensile load for use as a structural adhesive and that B-72 used as an interface barrier is sufficiently strong for structural joints as well as able to increase reversibility of bonds where polyester or epoxy is used as the adhesive. However, solvent loss from the B-72 adhesive or B-72 barrier must be sufficient to assure full bond strength. Further study is needed to evaluate the adhesive qualities of B-72, since characteristics such as the porosity and absorbency of the substrate and the possible long-term reordering and creep of the copolymer under constant long-term stress could affect the adhesive bond.
TITRE—L'utilisation du Paraloid B-72 comme adh�sif dans les structures et comme couche isolante pour de tels joints: �valuation de sa solidit� et de sa r�versibilit�. R�SUM�—Les copolym�res � base d'acrylique sont maintenant bien connus des restaurateurs. De par son estimable stabilit� et r�versibilit�, le Paraloid B-72 est tr�s fr�quemment utilis� en tant que couche de finition, consolidant et adh�sif. De plus en plus, le B-72 est utilis� comme adh�sif dans le remontage de grandes sculptures en pierre. Il est aussi utilis� comme couche isolante afin th�oriquement de pr�ter une plus grande r�versibilit� aux joints cr��s avec des adh�sifs moins r�versibles, tels ceux � base d'�poxyde ou de polyester, et aussi pour limiter la p�n�tration de ces adh�sifs dans le mat�riau de base. Deux diff�rentes �tudes ont �t� entreprises afin d'�valuer ces syst�mes et afin d'�tablir une comparaison directe entre le B-72 et les adh�sifs � base d'�poxyde et de polyester qui sont couramment utilis�s dans les structures, du point de vue de leur r�versibilit� et de leur r�sistance � la tension et au cisaillement.Des essais modifi�s bas�s sur les tests de l'association am�ricaine des standards ASTM ont �t� employ�s pour d�terminer la force des joints form�s entre deux surfaces en marbre avec des adh�sifs � base d'�poxyde et de polyester et des solutions tr�s concentr�es de B-72 dans deux solvants couramment employ�s. On a �galement test� des joints cr��s avec des adh�sifs � base d'�poxyde ou de polyester et dont les surfaces des mat�riaux de base avaient d'abord re�u une couche isolante de B-72. Les r�sultats indiquent que dans certains cas, le B-72 peut �tre suffisamment fort � lui seul pour r�sister aux forces de tension en tant qu'adh�sif pour les structures. Lorsqu'il est utilis� comme couche isolante, le B-72 est suffisamment fort pour des joints structuraux et sert � augmenter la r�versibilit� des joints faits d'adh�sifs � base de polyester ou d'�poxyde. Cependant, l'�vaporation du solvant utilis� avec l'adh�sif ou la couche isolante en B-72 doit �tre compl�te afin d'assurer une pleine force au joint. D'autres recherches sont n�cessaires afin d'�valuer les qualit�s du B-72 en tant qu'adh�sif car la porosit� et l'absorptivit� du mat�riau de base, ainsi que le vieillissement et fluage du copolym�re sous la pression constante pourraient � long terme affecter l'adh�sion.
TITULO—El Paraloid B-72 como adhesivo estructural y como barrera en uniones adhesivas estructurales: evaluaciones de fuerza y reversibilidad. RES�MEN—Los copol�meros acr�licos son bien conocidos en la pr�ctica de la conservaci�n. Debido a su relativa estabilidad y reversibilidad, se ha encontrado un amplio rango de aplicaciones para el Paraloid B-72 como revestimiento, consolidante y adhesivo. El B-72 esta siendo usado cada vez con mayor frecuencia como adhesivo para ensamblar grandes esculturas de piedra y tambi�n en barreras interfaciales en las que, te�ricamente, otorga gran reversibilidad a uniones hechas con adhesivos menos reversibles, como epoxy o poli�ster, y limita la penetraci�n de estos materiales en el substrato. Los dos estudios que se describen a continuaci�n fueron realizados con el fin de hacer una evaluaci�n inicial de estos sistemas y de comparar el B-72 con los adhesivos estructurales m�s comunes, como epoxy y poli�ster, desde el punto de vista de los esfuerzos de corte y tracci�n y de su reversibilidad.Se realizaron ensayos de acuerdo a normas ASTM modificadas para determinar las fuerzas de cohesi�n m�rmol-m�rmol formadas con epoxy, poli�ster y soluciones altamente concentradas de B-72 en dos solventes com�nmente usados. Tambi�n se evaluaron uniones hechas con adhesivos epoxy y poli�ster que inclu�an barreras adicionales de B-72 en ambos substratos adherentes. Los resultados indican que en algunas soluciones el B-72 por s� solo puede ser lo suficientemente fuerte bajo carga de tracci�n para ser usado como adhesivo estructural y que, cuando es usado como barrera interfacial, es lo suficientemente fuerte para formar uniones estructurales, pudiendo incrementar la reversibilidad en los casos en que el epoxy o el poli�ster han sido usados como adhesivos. No obstante, la p�rdida de solvente cuando el B-72 es usado como adhesivo o como cubierta de barrera debe ser suficiente como para asegurar la total fuerza de la uni�n. Ser�a necesario realizar estudios adicionales para evaluar las cualidades del B-72 como adhesivo ya que las caracter�sticas de substrato, como porosidad y absorbencia, y el posible reordenamiento y deslizamiento del copol�mero bajo efectos de tensi�n constante a largo plazo, podr�an afectar la uni�n adhesiva.
1 INTRODUCTION
Finding appropriate adhesives for the reassembly of sculpture has challenged objects conservators for many decades. The conservator seeks a combination of properties, such as long-term stability, appropriate bond strength, ease and flexibility of application, minimal shrinkage, and, most important, maximum reversibility, that together are not the same set of characteristics typically sought by the adhesive manufacturer. In most cases it is the last characteristic—reversibility—that is lacking in modern structural adhesive formulations, since the commercial emphasis is on the lasting qualities of the bond and not on its intentional undoing.
1.1 ATTRIBUTES OF ADHESIVES
The use of more traditional animal glues and plant gum or resin-based adhesives, such as hide glue and shellac, has been superseded by the availability of modern synthetic resins, such as epoxies and polyester resins, for the reassembly of large stone sculpture. While one reason for this shift was the growing concern over the long-term stability and strength of these traditional organic-based materials, of greater influence was the ease of application offered by the newer products. One can now choose from a vast array of viscosities and working times (both pot life and cure schedule) and be assured of the reliability of a room-temperature cure and a strong bond as long as relatively simple guidelines are followed.
As a result, for the last 30 to 40 years (epoxy adhesives became commercially available in the early 1950s and polyesters slightly earlier) conservators have faced trading ease of reversibility for ease of application, as well as strength and reliability. Reversibility—that is, the complete undoing of an adhesive bond with no or minimal effect on the substrates—is, however, one of the most important tenets of modern conservation. Bonds made on large-scale marble sculptures must at times be undone, either during the reassembly process or during a subsequent treatment, often many decades later. There are always risks attending any attempt to reverse a treatment, and efforts to undo bonds formed with modern structural adhesives carry a significant number of potential problems and dangers. Adhesives such as epoxies are not formulated to be taken apart once the cure is complete; indeed, their resistance to most common solvents is considered to be one of their best characteristics. As a result, such adhesives will not directly dissolve, or at least will not readily dissolve, in solvents most familiar to the conservator or under conditions that the conservator is able to provide with practicality. At best the conservator might soften and swell the epoxy or polyester, and thus weaken and break the bond, by the direct application of a solvent or by placing the bonded object in an environment that contains solvent vapor. The solvents most effective in this process, however, present significant health risks to the conservator. Dichloromethane (methylene chloride), for example, is a suspected carcinogen, the fumes of which metabolize in the human body to carbon monoxide. It is not only a highly effective degreaser of skin but also has detrimental effects on the central nervous system (Sullivan and Krieger 1992; Clydesdale 1998). Nonetheless, methylene chloride is a major component in commercial formulations that are sold as epoxy or paint “strippers” and are often used by conservators to swell and soften cured epoxy bonds. If the bond surface is large and the bond line thin (and/or the substrates have complex surface morphologies), the solvent may take a considerably longer time to penetrate and completely affect the adhesive, particularly in the central area of the joint. A large amount of solvent or prolonged exposure to solvent vapor is often required. The swelling undergone by the adhesive as it takes up solvent is also of concern. Such a volume increase can present significant problems by introducing stresses that can cause cracking or spalling of original material of the sculpture. Evaluations carried out at the J. Paul Getty Museum have shown that a wide range of both epoxy and polyester adhesives swell significantly when exposed to solvents such as methylene chloride.1
Fig. 1.
Two approaches to calculating the maximum tensile and compression loads, as well as the shear load, on a joint bonding an arm fragment to a torso of a life-size figurative marble sculpture
 |
All these drawbacks have been reluctantly accepted in order to take advantage of the strength and long-term stability of these adhesives. However, a review of the characteristics of most commonly used epoxies shows that most are stronger than necessary for the applications to which the conservator puts them. For example, a bond holding an extended arm (without an internal support pin) to the shoulder of a life-size figurative marble sculpture may experience a maximum tensile stress of only 48 kPa (7 psi) and an overall shear stress on the adhesive of 5 kPa (0.75 psi) (fig. 1).This calculation is based on an arm segment with a diameter of 15.2 cm (6 in.) at the joint; a segment length of 27.9 cm (11 in.); a distance of 17.8 cm (7 in.) from the joint to the center of gravity of the segment; and a segment mass of 9.545 kg (21 lbs. or 93.45 Newtons of force). The calculation assumes that both substrates are relatively smooth and that the joint angle is close to 90 degrees. The tension, compression, and shear forces can be calculated using figure 1, formula A, a common cantilevered beam formula (Oberg et al. 1988), or a basic stress formula (fig. 1, formula B), adapted for this particular application.2 A more complex surface such as a fractured break would increase with both the surface area of the joint and the coefficient of friction, lowering the actual shear stresses on the adhesive bond. It should be kept in mind, however, that in reality the maximum tensile stress of 48 kPa (7 psi) modeled for this example joint can, and most probably would, be exceeded by many magnitudes due to dynamic loads such as someone lifting the sculpture by the arm or conditions imposed by transport. Because of these unknown but likely additional loads, adhesives are often chosen that have greater strength than actually required in static load conditions.
Fig. 2.
A cross section of a joint showing a bond made with a structural adhesive such as epoxy or polyester and the presence of a barrier layer such as B-72 over the two substrate surfaces
 |
Nonetheless, while joint configurations that result in far greater stress in the bond line occur quite often, the previous example does serve to compare the required 48 kPa (7 psi) tensile strength to the common tensile strengths of most structural adhesives such as Araldite AY 103 cured with HY 991 hardener from Ciba Geigy (Ciba 1999), which provides an ultimate tensile strength of 42,749 kPa (6,200 psi). Adhesives based on unsaturated polyester resins such as Akemi Marmokitt 1000, another common adhesive used in sculpture conservation, offer tensile strengths of around 33,785 kPa (4,900 psi) or greater (Akemi 1999; Axson 1999). Clearly the strength of these two adhesive types is often greater than needed even when accommodating necessary safety factors. The conservator has little choice, however, when faced with the need for a structural bond, since nothing has yet appeared to take the place of this limited group of adhesives.
Some conservators have attempted to overcome the problem of reversibility by turning to the acrylic copolymer Paraloid B-72, not as an adhesive directly, but as a barrier applied to both substrates of the joint prior to introducing a structural epoxy or polyester adhesive (Garland and Rogers 1995; Bone 1999; Bruno 1999; Christman 1999). Figure 2 illustrates a bond made with this approach. At first glance the concept has merit. The B-72 layer is readily (or more readily) soluble in acetone, which lends a degree of reversibility to the bond. The barrier layer is dissolved with minimal or no swelling of either the B-72 or the structural adhesive. However, there is an apparent flaw in this logic when one considers that the structural adhesive is simply bonding the two coatings of B-72 together with no significant penetration of one into the other (assuming there is no interaction between the structural adhesive and the acrylic copolymer to create a form of fusion bond). The bond strength is directly dependent upon the adhesion of the B-72 to the marble substrate and to the structural adhesive interlayer, as well as the cohesive strength of both. Given the strength of epoxy and polyester adhesives, the critical link, therefore, is the B-72, and in large part the integrity of the bond depends upon the strength of this material as an adhesive.
Surprisingly, for a material so prevalent within the field of conservation, little information is available on the strength of B-72 as an adhesive, particularly tensile and shear evaluations. Few controlled tests following standard adhesive test procedures based specifically on the American Society of Testing and Materials' guidelines, such as ASTM D1002 for lap shear and ASTM D2095 for tensile (Pocius 1997), appear in the literature. To date the most-controlled studies have evaluated B-72 as a film (Down 1984; Down et al. 1996) rather than evaluating its “practical adhesion” characteristics as a bonding layer between two substrates. B-72 is known to soften at 30-35�C (86-95�F) and can, at that point, yield (Selwitz n.d.). Although bond failure through separation may not occur, the softened adhesive will allow movement in the bond that, for a structural adhesive used in a joint to assemble large-scale stone sculpture, must be considered failure. Additionally there has been little work done on the long-term creep and cold-flow of the copolymer at room temperature under long-term stress.
1.2 SET OF STUDIES
This account is the integration of two separate studies. Shear tests using a range of adhesive combinations were undertaken by Kathleen Garland and Joe Rogers at the Nelson-Atkins Museum of Art, Kansas City, Missouri, as part of the reassembly of a large Greek marble sculpture depicting a lion. Tensile strength evaluations were undertaken at the J. Paul Getty Museum, Los Angeles, California, by Jerry Podany as a continuation of work begun in 1994 by Martha Simpson, then interning in the Department of Antiquities Conservation at the Getty Museum. This work focused upon evaluating the ultimate strength and apparent benefits of B-72 as a barrier within a structural joint. William Freeman of Allied Signal Federal Manufacturing and Technologies in Kansas City, Missouri, undertook the design and execution of the direct testing and data coordination in both cases. While differences in approach and choice of materials exist in the two studies, such as the type of marble used and minor differences in percentages of the barrier mixtures, the conclusions, when brought together, provide useful information for the evaluation of B-72 as an adhesive.
The initial purpose of both studies presented here was to evaluate the strength of a bond made with B-72 barriers on marble substrates before the introduction of either a structural epoxy or polyester adhesive. The hypothesis made was that the bonds including a B-72 barrier would be similar, if not identical, in strength to those made with a high concentration B-72 solutions alone, such as a 1:1 w/w solution in acetone (Koob 1986) or toluene, both often used in conservation practice. It was also postulated that both these adhesive bonds (those made with a B-72 barrier and those made with B-72 alone) would be significantly weaker than bonds made with commercially available epoxy and polyester structural adhesives. This assumption suggested that there was little reason to include the structural adhesive between the joint where a B-72 barrier had been applied, since the strength of the bond would be fully dependent upon the strength of the barrier material and therefore equal to a B-72 adhesive bond. Whether the B-72 as an adhesive was sufficiently strong to meet the needs of a structural joint for marble substrates was unknown. The final results of the tests confirmed some parts of these assumptions but also presented surprising information about the nature of B-72 as an adhesive. They also reflect the complexity of choices presented to the conservator when an appropriate adhesive must be selected.
2 APPROACHES TO TESTING
Marble test coupons were prepared using a range of adhesives and the bonds evaluated by both shear and tensile tests. Shear testing (tests designed to place opposing loads along opposing faces of a bond) is the most common evaluation of adhesives, since the test coupons are more easily produced and since most demands on an adhesive bond in commercial applications are defined as shear. However, evaluations of the shear test results and the experience of preparing the test samples led the authors to also pursue tensile load evaluations and to increase the number of samples and range of adhesive mixtures tested. Tensile tests impose a force along the axial direction of a bond perpendicular to the bond plane. This kind of load is often prevalent in joints of reassembled largescale stone sculptures. An example of a common tensile load is the outstretched arm of the sculpture in figure 1. If we assume that a shear pin is not part of the joint in this particular example and that the assembly looks like figure 3a, then the forces acting upon the arm might at first appear to be only shear, that is, directly downward due to gravity and roughly parallel to the joint surface. However, should this joint derive from a fracture, the substrates will have a complex surface morphology, and the two surfaces will, to some degree, physically “lock” into place. On closer examination, it is easy to see that the direct downward movement of one surface relative to the other is blocked by the interlocking roughness of the two substrates (the coefficient of friction is high). If the arm is to move in a direction parallel to the joint surface (shear movement), one substrate must move away from the other in a perpendicular direction (fig. 3b) to overcome the “interlocking surfaces” of both substrates. The adhesive layer, as a result, experiences tensile load. Even if we were to introduce a shear pin, which is common in joints such as the one we are discussing, the forces in the adhesive remain predominantly in tensile. Approximately one-half of the joint (that part below midpoint of the joint surface) will be placed in compression, while the other half will be placed in tension as the arm attempts to drop away. With the exception of relatively flat fragments that are adhered to a vertical or nearly vertical surface and have smooth substrates, the predominant force acting upon most joints will be a combination of tension and compression. Nonetheless, shear load evaluations can be significant, particularly when the joint surfaces are smooth, and a proper evaluation of an adhesive system will include both shear and tensile data.
Fig. 3.
A. A joint of two fractured surfaces showing the complex morphology that provides physical “key locking” of the two substrates, increasing the coefficient of friction and the resistance to shear load. B. Diagram as in A, illustrating the necessity of outward movement normal to the two interfaces before downward displacement (shear slip) can occur. This type of movement places the bond in tensile load and is typical of joints made in the reassembly of sculpture.
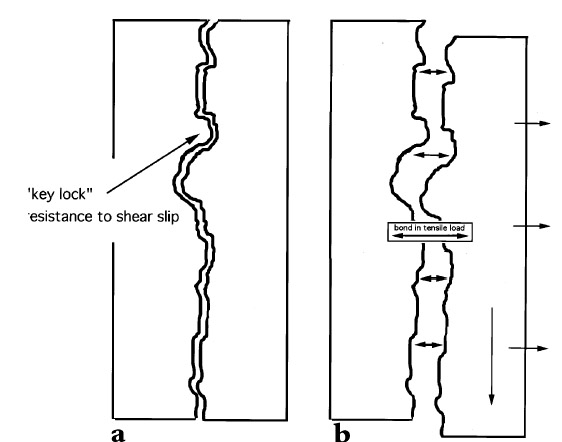 |
3 SHEAR TESTS
Lap shear tests were undertaken in October 1997 in conjunction with the design of a treatment for a fourth-century B.C. Pentelic marble lion in the collection of the Nelson-Atkins Museum. Several large sections of the sculpture, weighing approximately 4,000-5,300 Newtons (900-1,200 lbs.) and a mass of 400-540 kg each, were to be adhered to others as part of the reassembly process. Pursuing the desire that these joints be more easily reversible, the conservation staff began to evaluate the use of B-72 barriers and B-72 as an adhesive compared to more commonly used structural adhesives such as Akemi polyester and Araldite AY 103 epoxy. To more directly mirror the bonds that would be made in the project, the test coupons were made of Pentelic marble (Marble Enterprises 1999), identical to the type used to carve the lion sculpture in ancient times.
Fig. 4.
A shear test coupon
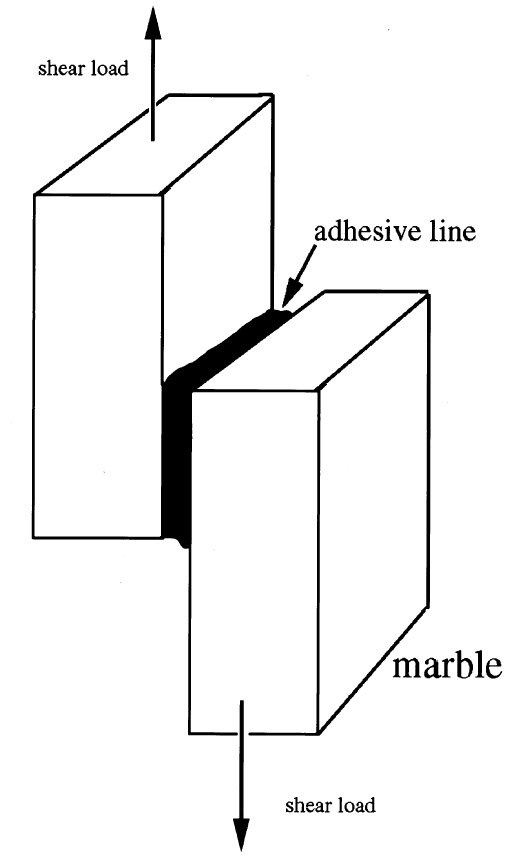 |
3.1 ADHESIVES EVALUATED
Five samples of each of the following adhesive groups were prepared:
- Araldite AY 103 with HY 991 hardener (100 resin to 40 hardener, w/w), cured for one week.
- Akemi Marmokitt 1000 transparent knife grade polyester with benzoyl peroxide–based hardener, cured for one week.
- 22% B-72 in acetone w/v applied as a double coat to both joint substrates and then bonded with epoxy. A 22% solution was chosen because of good working properties as a coating. One coat was applied by brush, left to dry for several hours, followed by an application of a second coat, which was left to dry for one month prior to the application of the Araldite epoxy (AY 103 with HY 991 hardener), which in turn was allowed to cure for one week.
- 22% B-72 in acetone w/v applied as a double coat to both joint substrates and then bonded with Akemi polyester. One coat was applied by brush, left to dry for several hours, followed by an application of a second coat, which was left to dry for one month prior to the application of the Akemi polyester, which in turn was allowed to cure for one week.
- Araldite AY 103 with HY 991 hardener with the addition of fumed silica to make a thick paste (100 parts resin to 40 parts hardener to 30 parts fumed silica by weight). These samples were applied using a shim to ensure that the five samples had a consistent adhesive line of 3 mm (0.125 in.) in thickness. In this evaluation the fumed silica was added to increase the viscosity of the resin so that it might be evaluated as a gap filler as well as an adhesive. Improvements of the cohesion and adhesion were not the intention in these specific samples, though the results do suggest that both were increased.
3.2 SAMPLE PREPARATION
Each sample was made of two small slabs of Pentelic marble (shear strength of 4,212 kPa or 611 psi) nominally 19 mm (0.75 in.) wide x 31.75 mm (1.25 in.) long x 9.52 mm (0.375 in.) thick, adhered with an overlap of 12.7 mm (0.5 in.) as described in ASTM D1002 (Skeist 1977; Pocius 1997) and illustrated in figure 4. Since there was a small variation from the nominal dimensions in each of the samples, the actual measured width and overlap length to the nearest 0.025 mm (0.001 in.) were used to calculate the shear area for each specimen. Even small variations in the width and lap length cause substantial changes in the calculated shear area and stress, so the accurate dimensions of each sample were used in the individual calculations. The samples were washed in a dilute Orvus (sodium alkyl sulfate) anionic detergent solution using stencil brushes, rinsed in distilled water, and then air-dried for three weeks. The samples were degreased with acetone, allowed to dry, then adhered with “finger” pressure. Excess adhesive was wiped off with acetone on a dry swab or mechanically removed after full cure.
4 TENSILE TESTS
Tensile evaluations were based upon the standard ASTM D2095 (Skeist 1977; Pocius 1997) but modified sufficiently to accommodate a marble substrate and assure strict alignment of the samples for accurate results and minimal deviations. It was decided that a common epoxy and polyester adhesive would be compared to both a commercially available B-72 adhesive (HMG brand) and B-72 in a high solids solution, often used as an adhesive for ceramics (Koob 1986). Joints prepared with a B-72 barrier and a structural adhesive were also tested. Acetone and toluene were used for the B-72 high solids solutions (used as an adhesive) and for the lower percentage barrier coatings. While other solvents are used in the application of B-72, such as methyl ethyl ketone, it was felt that acetone and toluene represented the most common and appropriate ranges of volatility and solvent action. Toluene provided a reasonable comparison to acetone, clearly reflecting the different effects the two solvents have on the polymer chain (Hansen 1994), the absorption of the polymer solution into the substrate, and the differing rates of solvent loss after application.
4.1 ADHESIVES TESTED
Seven samples of each of the following adhesive groups were prepared at the J. Paul Getty Museum:
- Epoxy: Araldite AY 103 epoxy resin with HY 991 hardener
- Polyester: Akemi Marmokitt 1000 knife grade polyester with benzoyl peroxide–based catalyst
- B-72 HMG adhesive
- 1:1 w/w B-72 in acetone
- 1:1 w/w B-72 in toluene
- 17% B-72 in acetone3 w/v applied in a single coat to both joint substrates and then bonded with epoxy
- 17% B-72 in acetone w/v applied in a single coat to both joint substrates and then bonded with polyester
- 17% B-72 in toluene w/v applied in a single coat to both joint substrates and then bonded with epoxy
- 17% B-72 in toluene w/v applied in single coat to both joint substrates and then bonded with polyester
Fig. 5.
A tensile test coupon. All dimensions are given in metric (mm) with inches in parentheses
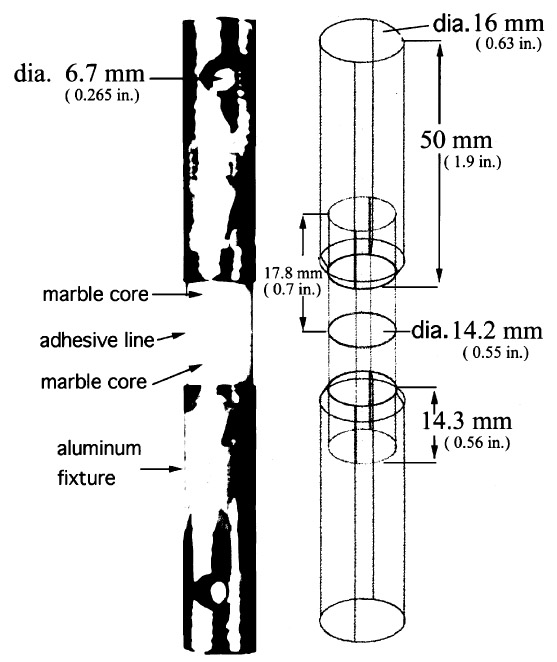 |
4.2 SAMPLE PREPARATION
The test specimens were prepared as follows: one end of each marble core was adhered into one end of an aluminum holding fixture. The exposed ends of the marble cores were joined together with a range of adhesive types to produce sets of test coupons shown in figure 5. One hundred and twenty-six aluminum holders were prepared by drilling a single hole into one end of an aluminum rod (see fig. 5 for dimensions). At the opposite end of the aluminum holders, a small-diameter hole was drilled at right angles to the central axis. These were used for securing the coupon in the testing apparatus.
Marble cores, 14.2 mm (0.55 in.) in diameter and 17.8 mm (0.7 in.) long, were drilled from a single slab of Sivic marble, which was selected because it is a medium-grain white marble from Yugoslavia that is highly consistent and free of inclusion planes, vugs, and voids (Walker Zanger 1999). An average tensile strength for Sivic marble of 4,520 kPa (655 psi) can be inferred by the results reported in table 3. Both ends of each marble core were ground flat and 90� to the central axis and finished with a 220 grit wetsanding. The cores were placed in an ultrasonic water bath and rinsed with deionized water followed by an acetone rinse to ensure the removal of any surface contaminant.
The first step in preparation of the actual test coupons was to adhere a marble core into one of the two aluminum holding fixtures using Araldite AY 103 with HY 991 hardener. Approximately 50-60 mg of adhesive was applied to the bottom of each fixture end hole to assure a thin and consistent bond line with minimum squeeze-out onto the edges of the core. The core was fully seated in the flatbottomed hole, leaving approximately one-half of the core length exposed. Alignment of the core with the central axis of the aluminum holder was critical and was assured before the bond was allowed to cure at room temperature. Once the first half of the test coupon was prepared, a matching core was bonded to the already seated core using one of the nine different adhesive types to be tested. The application of the adhesive on all the core-to-core bonds utilized a relatively consistent 20 mg of adhesive. The adhesive was dropped in the center area of one side of the joint. The other core was then brought into contact with the first and gently moved in a circular, as well as back-and-forth, motion to assure an even distribution of the adhesive. Firm downward hand-pressure was then applied to assure the squeeze-out of any excess adhesive and the creation of a thin, consistent bond line. Excess adhesive was gently removed with a dry swab, and the sample was aligned and allowed to set in an ambient environment of 21�C (70�F) (+/− 5�) and an RH of 55% (+/− 5%). The sample was supported in the vertical position for 24 hours to assure a reasonable set of the adhesive bond.
Following that 24-hour period, the second aluminum holding fixture was adhered to the assembly in the manner described above. True axial alignment of the four parts was assured by rolling the assembled test coupon on a horizontal steel bed that had been milled flat. If the coupon was in full contact with the bed surface and rolled smoothly over it, axial alignment was proper. After any needed realignments the coupons were laid in a metal v channel to cure for two weeks; then the axial alignment was again verified.
Table .
Bond Line Dimensions for Tensile Samples (μm)
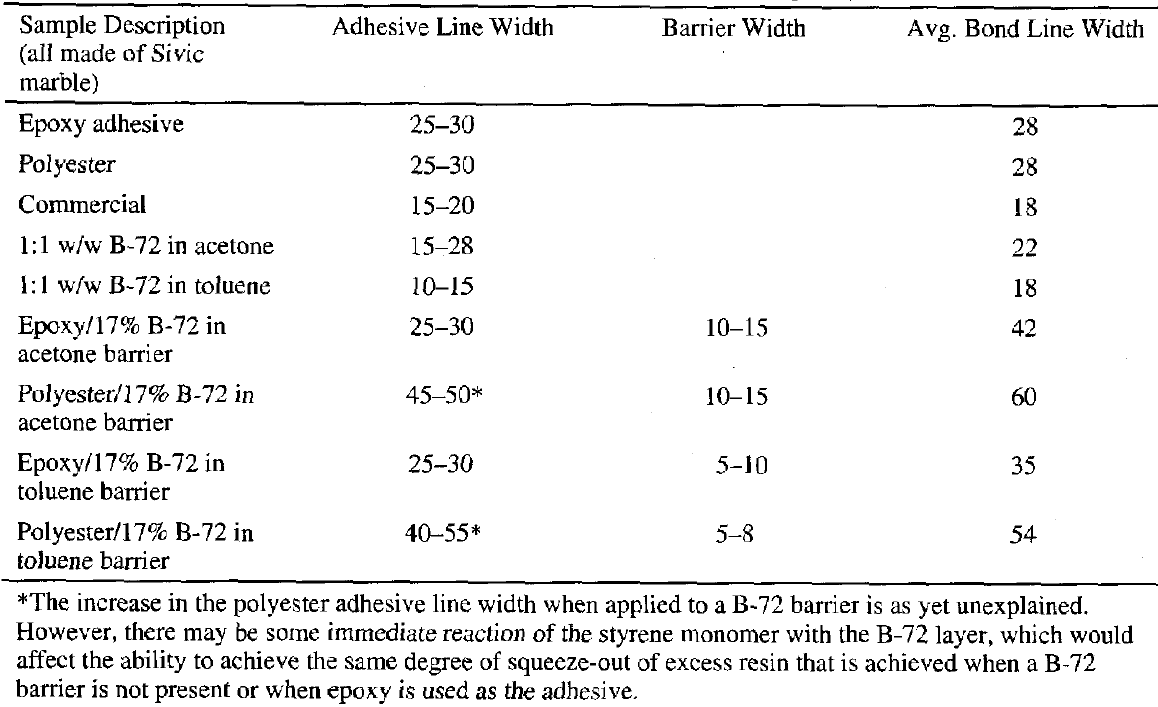 |
When the B-72 was to serve as a barrier layer between the substrates and the structural adhesive, a single coat of 17% w/v B-72 in acetone or toluene was applied to the substrate by four brush strokes using a soft 6 mm (0.25 in.) natural bristle brush, each stroke originating from the central point of the core surface and one final stroke to even any edge buildup. The barriers were allowed to dry for two weeks prior to bonding of the cores. All adhesive bonds were allowed to cure or air-dry for three weeks prior to testing.
4.3 BOND LINE EVALUATIONS FOR TENSILE TESTING
Two assemblies of each of the adhesive groups, consisting only of bonded marble cores, were prepared at the same time in an identical manner. After curing, these core sets were cut in half along the axial direction and the cut surface was polished. Each set was examined to determine bond characteristics, specifically the dimension of the adhesive line. Generally an average bond thickness of 0.1-0.3 mm (0.004-0.01 in.) is preferred in joints, since below 0.1 mm (0.004 in.) the stress applied to the bond may point-load asperities that have less adhesive between them (Bradley 1984). In addition, thinner bond lines may present a greater chance for voids, leading to weaknesses in the bond line. Bond lines greater than 0.4 mm (0.016 in.) may also lead to weaknesses due to voids in the bond line or an increase in microfissures. Moreover, thicker bond lines increase the dependency upon the cohesive strength of the adhesive, which is often weaker than its adhesive strength and may be insufficient for the stresses placed on the bond by shear loads.
Fig. 6.
Ultraviolet fluorescence microscopy images of three bond cross sections: A. epoxy; B. epoxy with 17% w/v B-72–toluene barrier layers; and C. epoxy with 17% w/v B-72–acetone barrier layers. The scale divisions are 10 �m each.
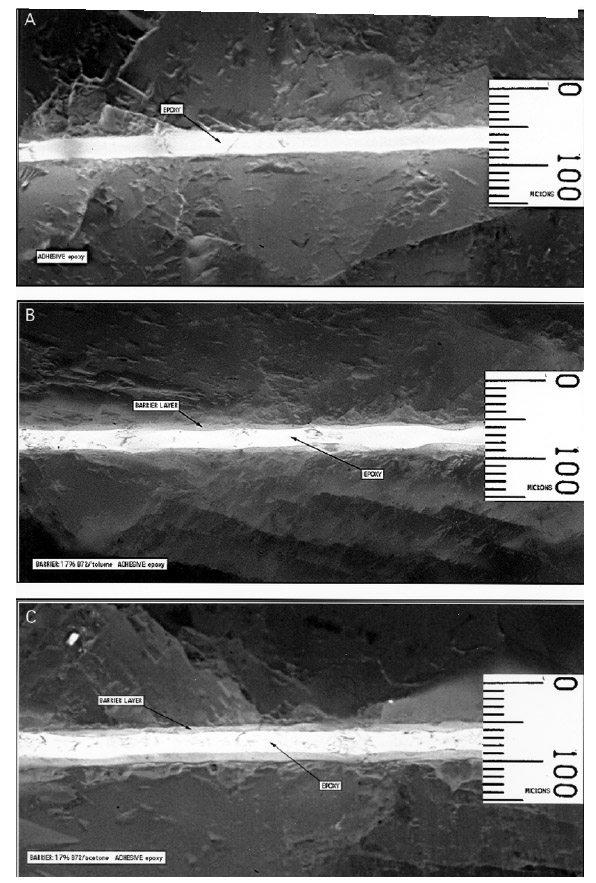 |
Table .
Pentilic Marble* Shear Test Results
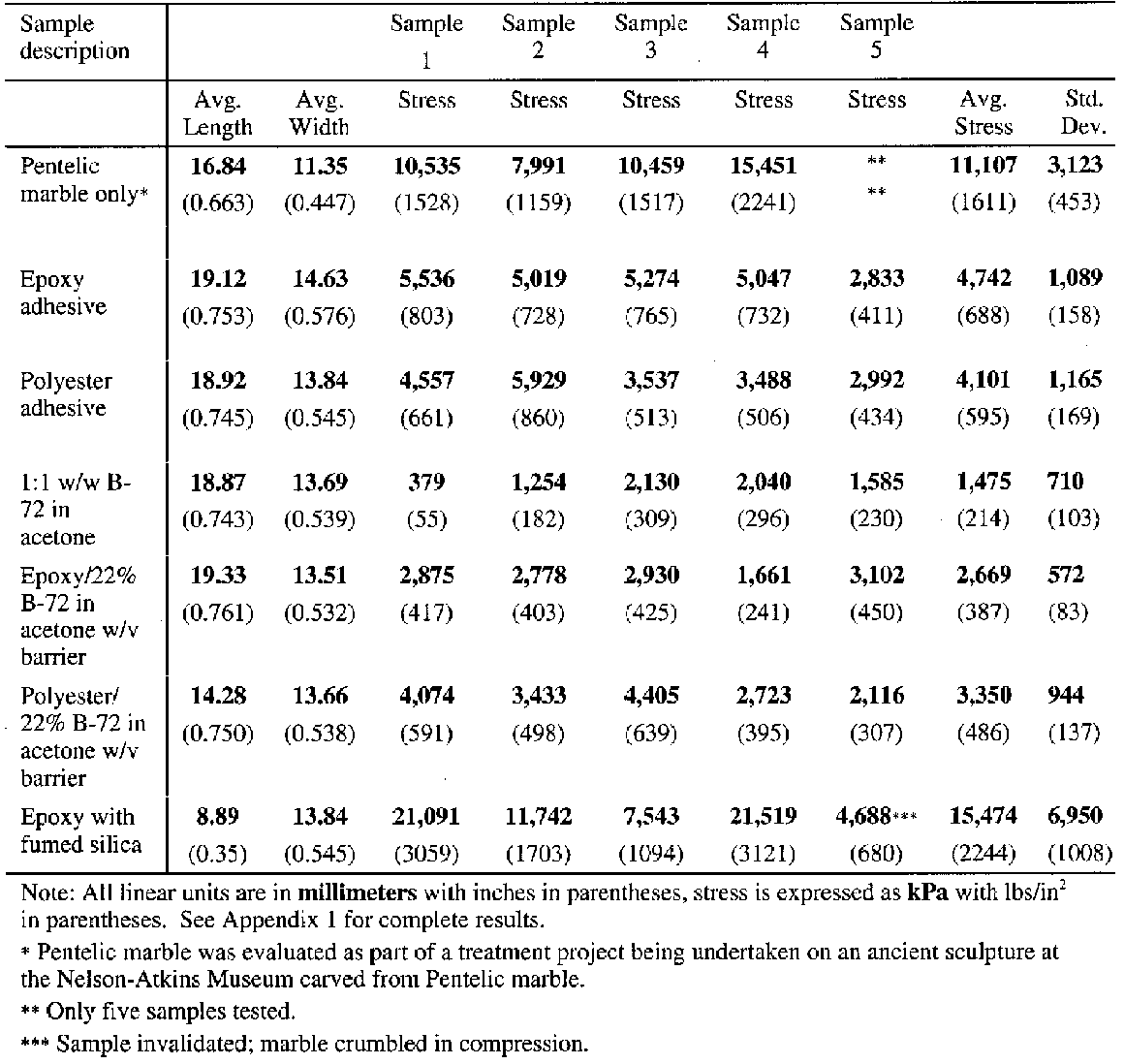 |
Examination of the sectioned bonds using UV microscopy revealed a relatively consistent bond line across the length of the joint, consistency between pairs of sectioned samples, and consistency between similar groups. For example, the epoxy bond line thickness was approximately the same whether the epoxy was used alone or with a barrier film, 25-30 �m. The average bond lines for the groups are listed in table 1.Figure 6 clearly shows that the difference in the barrier thickness achieved was somewhat dependent upon the solvent used to prepare the 17% w/v solution. The barrier thickness of the B-72–toluene mixture is less than the B-72–acetone mixture, most probably because the toluene mixture has greater “flow” under pressure and before drying. Because of this the squeeze-out from the joint is greater than the squeeze-out from the acetone mix for the same amount of applied pressure. Other factors, such as a slight absorption into the marble, may also come into play; however, there was no clear indication that the B-72 had penetrated to any significant degree into any of the marble samples.
Table .
Sivic Marble* Tensile Test Results
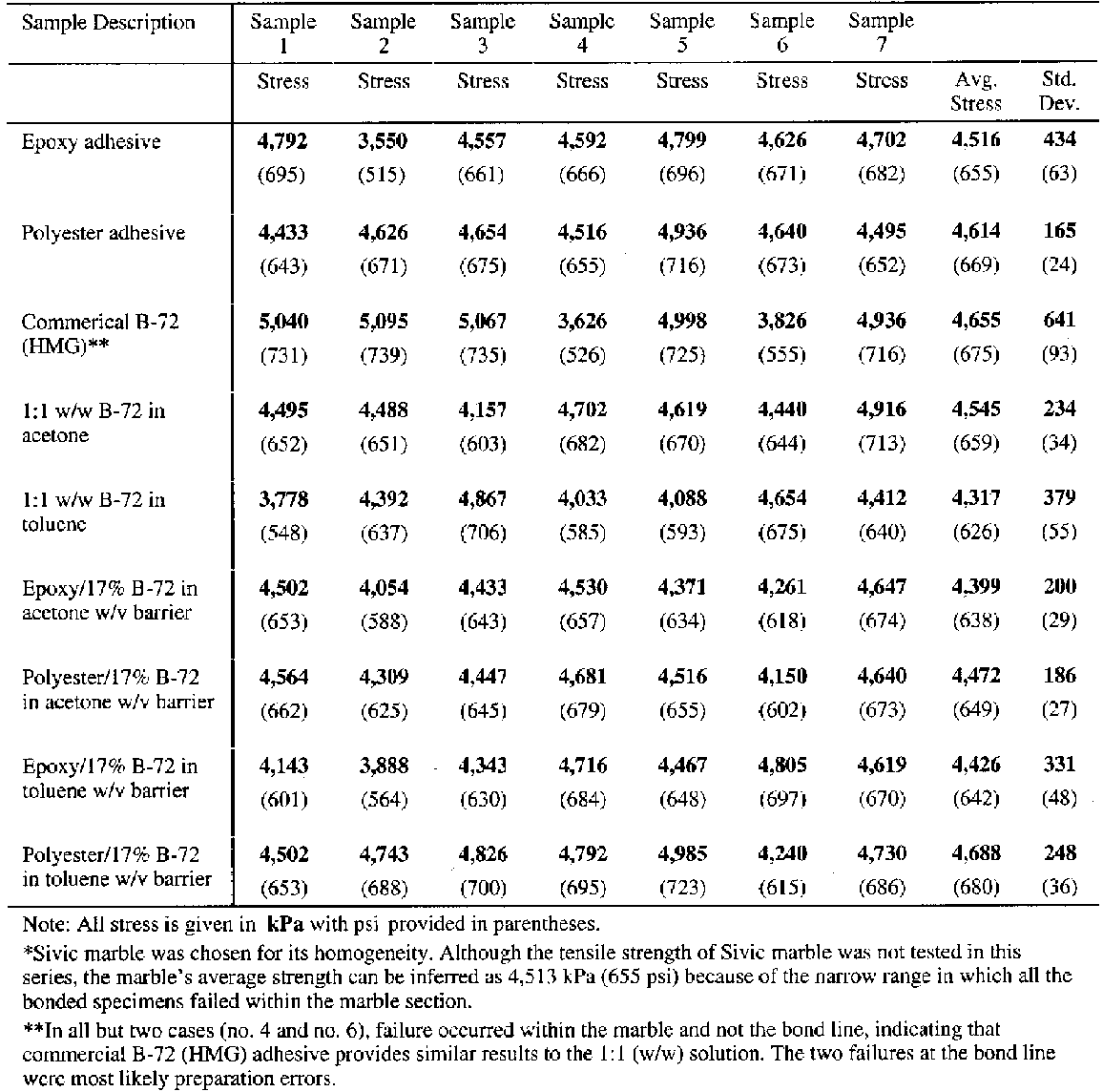 |
5 REVERSIBILITY EVALUATIONS
Since an assumed major benefit of the B-72 barrier coating as part of the structural joint was that it would lend greater reversibility to the joint, it was essential to evaluate this assumption. Nine test coupons consisting of two cores each adhered with the same adhesive and prepared in the same manner as described for the tensile test (not including the aluminum carrier) were prepared and dried or cured for the same period of time under the same conditions. These samples were then suspended in a closed vessel containing a saturated acetone vapor environment. The joint was considered “reversed” or failed when the bottom core of the bonded pair dropped away.
6 TEST RESULTS
The results of the shear and tensile tests are reported in tables 2 and 3 respectively. The shear and tensile tests were carried out at Allied Signal FM&T using applied tensile load at a rate of 0.1 in./min. (crosshead speed) at 20.5�C (69�F), +/− 2�, and an RH range of 50-55%. In both shear and tension loads, the epoxy and polyester adhesive bonds were stronger than the marble. Under shear stresses the polyester assemblies failed within the marble at an average stress of 4,101 kPa (595 psi), while the epoxy-bonded assemblies failed within the marble at an average stress of 4,742 kPa (688 psi). In the tensile evaluations the epoxy bonded assemblies failed within the marble at an average stress of 4,519 kPa (655 psi), and the polyester-bonded assemblies failed, at an average stress of 4,616 kPa (669 psi). The results are not unexpected, since the strength of both adhesive types is quite high and was assumed to be higher than that of the two marble types making up the coupons.
It is interesting that the shear coupon bonded with epoxy with added fumed silica showed a significantly higher fracture strength, 15,474 kPa (2,244 psi), than did the solid marble coupon or the coupons bonded with either unbulked epoxy or polyester tested under shear load. Although this coupon was initially prepared to evaluate the bulked epoxy as an adhesive or gap filler, the results suggest that fumed silica may have significant effects on the physical characteristics of an adhesive. While some investigations have been reported on this topic in the conservation literature (Byrne 1984), more work clearly needs to be pursued.
The explanation for the higher bond strength of this bulked sample as compared to the marble control sample is unclear; however, improved stress distribution may be part of the answer. Brittle materials like marble fail catastrophically rather than progressively like malleable materials such as metals. Failure in such brittle materials occurs when the stress anywhere exceeds the maximum that the material can withstand. If the stress distribution is nonuniform, failure still occurs when the highest stress anywhere in the joint reaches the failure stress limit. For a nonuniform stress distribution, the average stress computed will be lower than the actual maximum stress that precipitated the failure since some of the joint was below the failure stress. This relationship implies that in most cases the reported failure or average stress will be lower than the actual failure or local stress, due to nonuniform distribution of the stress. It also implies that any change in bonding methods that improves the uniformity of stress over the joint will enable the joint to carry a higher load, and the average stress at failure will be higher, even though the actual, local, failure stress in the marble is unchanged. Thus it can be postulated that the bulked epoxy distributed the load more evenly over the surface of the bond and hence increased the overall effective strength. It may appear that the marble broke at considerably higher stress levels, higher than the value for whole marble, but what actually occurred is that the bulked epoxy bond is so strong that it distributes the stress more evenly to the marble. The marble then seems to break at much higher stress levels.4
The tests using 1:1 w/w solutions of B-72 used as an adhesive provided interesting data as well. In shear the B-72–acetone mixture failed within the bond line at an average stress of 1,475 kPa (214 psi). However, in tension the B-72–acetone mixture failed at an average stress of 4,545 kPa (659 psi) and the B-72–toluene mixture at 4,316 kPa (626 psi); both failed in the marble, not in or at the bond line.
When B-72 was used as a barrier coat, there was again surprising information from the test results. In shear the B-72–acetone barrier combined with the epoxy bond failed at an average stress of 2,669 kPa (387 psi). All five samples in this case failed in the marble parallel to the bond line in shear failure. In tension, the B-72–acetone barrier combined with an epoxy bond failed at an average stress of 4,399 kPa (638 psi) and the B-72–toluene barrier combined with an epoxy bond failed at an average stress of 4,426 kPa (642 psi). Although the differences between the shear and tensile results are significant, it is worth pointing out that in tensile all the coupons, and in shear most of the coupons, bonded with a B-72 barrier failed in the marble and not within or at the bond line.
When polyester was used as the interlayer adhesive between the B-72 barrier layers, shear failure occurred within the marble parallel to the adhesive line at an average stress load of 3,350 kPa (486 psi). In tension the failure again occurred within the marble, often far from the bond line, at an average stress of 4,472 kPa (649 psi) for the B-72–acetone barrier layers and 4,688 kPa (680 psi) for the B-72–toluene barrier layers, both with polyester bond lines.
The results of the reversibility tests indicated that the bonds made with B-72 as an adhesive and those made with B-72 as a barrier coat were considerably more reversible than those made with only Araldite AY 103 epoxy or Akemi polyester. All the bonds made with B-72 as an adhesive or as a barrier reversed within the first 8 hours of solvent fuming. The polyester bond reversed within 16 hours. After two months in the fume chamber (at which time the test was concluded), the epoxy bond remained intact.
7 DISCUSSION
It is important to note that all of the samples tested for tensile strength, with the exception of two samples prepared with HMG B-72 adhesive, failed within the marble structure and not within or at the adhesive bond line. The two anomalies can be assumed to represent errors in the coupon preparation, though other factors yet unidentified may be at work including the lower viscosity of the commercial B-72 mixture or possible inconsistency of the solvent/copolymer ratio within the tube. All eight adhesive categories failed within the stress range of 4,317-4,688 kPa (626-80 psi) in tension, with the exception of the B-72 HMG adhesive group, which failed at higher stress (an average stress of 5,027 kPa (729 psi) if the two anomalies, which failed at the adhesive line, are dropped from the calculation). This latter set of results warrants further investigation, since the largest variation in results was within the two groups using commercially prepared B-72.
7.1 SHEAR AND TENSILE STRENGTH OF B-72
From the shear data, the B-72–acetone adhesive mixture appears unsuitable for marble-to-marble bonds where large shear forces might be present. On the other hand, the B-72–acetone barriers in shear and the B-72–acetone and B-72–toluene in tensile appear to function quite well. Clearly the B-72 marble-to-marble bonds, by themselves, are stronger under tensile loading conditions than the marble. The strength difference between the coated barrier bonds compared to those bonds formed by traditional structural adhesives is insignificant. This finding suggests that for tensile bond strengths there is little or no difference between the use of a structural adhesive (with or without a barrier coat) and B-72 used as an adhesive.
It is likely that the discrepancy between the shear strength of the B-72 as an adhesive and the shear strength of the bonds where B-72 was used as a barrier is not due to any interaction between either the epoxy or the polyester and the B-72 but rather results from the degree to which the B-72 layer had dried prior to the test. Solvent loss from the B-72 will be significantly slower within an adhesive joint compared to the same mixture when applied as a thinner coating and dried with the entire substrate surfaces exposed before bonding. The more solvent that remains in the B-72, the softer the material will be and the more “elastic in nature” it will appear since the solvent acts as a “plasticizer” and adds to the free volume of the polymer, resulting in a weaker bond line. We know, for example, that the choice of solvent, and certainly the retention of that solvent (based on factors such as volatility and rate of diffusion through the free volume of the polymer), can significantly lower the glass transition temperature (Tg) of a polymer compared with the bulk polymer (Horie 1987; Schilling 1989; Hansen 1994). For example, Selwitz (n.d.) reports that films cast from acetone have a higher Tg than those cast from a number of other solvents. Above some critical solvent content, the B-72 layer will cause the barrier or the bond line to act as a weak elastomer, stretching and eventually losing cohesive strength (Down 1984; Down et al. 1996).
An important point for the conservator to remember is that numerous variables come into play when preparing a real bond on a real sculpture. The tensile test coupons represented a 12.7 mm (0.5 in.) diameter bond surface, approximately 1.38 sq. cm (0.23 sq. in.), on low-porosity, relatively consistent marble types. Different substrates can have dramatic effects upon the bond strength as well as the reversibility. Higher open porosity of the bonded surfaces will lead to more absorption of either the solvent or the solvent-adhesive-barrier combination, which may change the strength of the bond. A larger bond area and a bond area with a more complex surface (such as a fractured marble surface) will impede the loss of solvent and dramatically increase the time needed to form a good, strong bond. In any case, it would be difficult and impractical, given the variety of sizes, complexities, and configurations of bonds, to determine with accuracy the point at which sufficient solvent loss has occurred and the bond has reached full strength. Although the authors intend to further test these variables, one suggestion does come forward. Given the evident tensile bond strength of B-72 with this particular type of marble, it may make good sense to coat both sides of the joint with B-72 and then, after sufficient drying time, to adhere the joint with an optional, non-solvent-based adhesive such as epoxy or polyester. The advantage of this method is that it ensures a full or sufficient loss of solvent from the B-72 barrier coatings while the joint substrates are fully exposed before the introduction of the second structural adhesive and before the mating or closing of the joint. Additionally, the 100% solid adhesive (such as epoxy) would assure the filling of any voids that might result from a less-than-intimate match of the two substrates to be bonded. A higher degree of reversibility is, in any case, maintained because of the barrier layer. Conservators should bear in mind that B-72 is a thermoplastic resin with the relatively low glass transition temperature range of 37-41�C (98.6-105.8�F) (Schilling 1989), and it may creep or cold-flow over time when the adhesive bond is under continual stress since creep is both temperature and stress dependent.5 This characteristic may be a concern even at midrange temperatures (18-24�C [65-75�F]) when the stress is maintained over decades.
7.2 CREEP AND COLD FLOW
All solids are elastic to some degree under stress. When that stress is high and/or when it is applied for extended periods of time, in the range of decades, some materials will break while others will become plastic. Materials presenting such plasticity may creep—that is, deform slowly under constant stress. When creep occurs at room temperature, it is normally called “cold-flow” (Skeist 1977, 101). Equally, all thermoplastic amorphous polymers or the amorphous parts of semicrystalline thermoplastic polymers (which can range from 40% to 70% of the polymer structure) are susceptible to creep under the influence of temperature (particularly rising above the polymer's Tg range) or stress (particularly over long time frames). Although it is commonly assumed that B-72 will not creep or cold-flow, very little verifiable testing of the copolymer has been done in this area, particularly looking at the copolymer as an adhesive and under continual stress levels expected of an adhesive. Most polymers are viscoelastic to some degree, however, and most viscoelastic solids show time-dependent deformation even at low stresses and temperatures below their Tg. Recent research has shown that some polymers, such as polymethyl-methacrylate, undergo strain hardening, which increases the polymer's resistance to plastic deformation (creep) after the initial yield (Crist 1993). However, no direct measurements of B-72 have been done to evaluate the contribution of these phenomena to its resistance or susceptibility to creep. Applications of Plexiglas in commercial glazing recognize design limits to avoid permanent deformation (creep) over a long time due to the weight of the Plexiglas sheet (Luskin 1983-84).
Susceptibility to creep is determined by a number of factors including the polymer's Tg. The lower the Tg, the more likely the polymer is to deform under normal conditions (serviceable loads at room temperature over a time frame of years or decades). The closer a polymer comes to its Tg, the more elastic it becomes. The softening of a polymer at its Tg is due to long-range segmental motions of the polymer chain, twisting and repositioning to accommodate stress, which in turn is dependent upon the stiffness of the polymer backbone as well as the presence and nature of pendant groups. In this second-order transition there is an increase in the polymer free volume, the ratio of which is normally around 0.025 at the Tg, but this ratio can also be greatly influenced by plasticizers or the presence of solvents. Over time, polymer chains can also reorder, leading to a change in the polymer's Tg as well as its strength and susceptibility to creep. Helical jumps in the polymer chain have been reported over time that can lead to mechanical relaxation which, in turn, can lead to chain diffusion between crystalline and amorphous regions, ultimately resulting in creep (Schmidt-Rohr and Richert 1999). To avoid these various movements and increases in elasticity, adhesives used for structural joints are often chosen for their high Tgs, which are considerably higher than room temperature. This characteristic also avoids differences in the linear coefficient of thermal expansion between the polymer (as it reaches its Tg) and the substrate. Araldite AY 103 with 956 hardener has a Tg of 83�C (181�F), and Akemi polyester has a Tg of 68-76�C (156-70�F). By comparison, B-72 has a Tg of 37-41�C (98.6-105.8�F). Although some epoxies such as Hxtal NYL, Araldite 2020, and Fynebond have Tgs closer to B-72 (40-45�C, 40-46�C, and 44-48�C, respectively), these are not commonly used in the conservation of large-scale sculpture, and some, such as Araldite 2020, are not commercially available in the United States.
8 CONCLUSIONS
These studies were prompted by reported uses of B-72 solutions in either acetone or toluene as barriers applied to substrates of a joint prior to the introduction of Araldite AY 103 epoxy or Akemi polyester structural adhesives. The evident purpose of this combination was to lend reversibility to the joint. As noted previously there is little information in the literature regarding the strength of B-72 as an adhesive. Since the strength of a bond in which B-72 might be used as a barrier directly depends upon the physical adhesive characteristics of the copolymer, it was decided to evaluate both B-72 and the commonly used structural adhesives, Araldite AY 103 epoxy and Akemi polyester, using standard tensile and shear tests.
The test results suggest that the application of a barrier, if relatively thin, conformal, fully continuous, and fully dried (solvent free) before the introduction of a secondary adhesive, provides a considerable degree of reversibility to a joint without decreasing the needed strength required to sustain either shear or tensile loads imposed on most structural bonds made for the reassembly of large stone sculpture. It appears that the B-72 is sufficiently strong to safely be part of a structural joint and to provide a reversible barrier between the substrates and the less reversible structural adhesive. However, the B-72 barrier must be fully dry. This state can be achieved by applying a thin barrier on both faces of the joint when both substrates are fully exposed and can easily lose solvent prior to the application of the structural adhesive. Because of the critical function of the barrier coating, the conservator must be diligent in assuring a consistent and thorough coating of the substrate. Any gaps or voids in the coating might provide an opportunity for the secondary adhesive (epoxy or polyester) to bond with the substrate and reduce the reversibility of the joint. These imperfections may also weaken the adhesive and cohesive strength of the barrier layers.
It must be kept in mind that these are preliminary results and the number of variables that must be considered and tested in the future are significant. The two types of marble used in these tests have a relatively dense structure, and results may change significantly if a more porous substrate were used. Perhaps a more important issue is that to date little has been reported on the cold flow or creep of B-72 (Griffin et al. 1983; Selwitz n.d.), particularly when prepared using a variety of solvents, under either continual tensile or shear loads over a range of temperatures or over extended periods of time. Additionally, solvent type may affect the thickness and consistency of the barrier (Selwitz n.d.), which in turn may have a significant effect upon the cohesive and adhesive strength of the barrier layers and on the strength of the overall bond. Retention of solvent will also affect the overall bond strength when practical drying times are used. Some solvents in B-72 mixtures require considerably greater time to leave the polymer, as in the case of toluene, which can take up to two months to evaporate thoroughly (Horie 1987, 104). The barriers and solvent-based adhesive bonds in this study were allowed to dry for several weeks at room temperature. Additionally, these evaluations were done by considering objects that are meant to be protected within a relatively controlled environment. Environmental variables, particularly in the outdoors, might have significant effects on the characteristics of the adhesive bond and also need further study.
As in any effort within conservation, progress toward a more reversible and yet sufficiently strong adhesive bonding system must be made cautiously and slowly, as each of these variables is identified and evaluated. The authors have presented this study as an initial step to bring this effort under way.
NOTES
1. In 1995 the Department of Antiquities Conservation of the Getty Museum undertook an evaluation of the swelling characteristics of seven common epoxies and two polyester formulations. It was found that after sufficient exposure to dichloromethane (methylene chloride) fumes in order to destroy the cohesive and adhesive strengths of the material, unrestrained samples of the adhesives swelled between 8% and 14%. This increase in volume, when experienced by a restrained sample (adhesive in a closed joint or within a hole under confined conditions), may induce sufficient pressure to fracture marble and disrupt fragile surfaces.
2. For calculation A: The stress (S) is arrived at by multiplying the moment, M (which is arrived at by multiplying the distance from the joint to the center of gravity, L), by the distance to the extreme fiber (C), which is measured outward from the axial center of the cross section of the segment to the farthest edge of that cross section. This product is then divided by the section moment of inertia (I), which, for a round cross section, is p multiplied by the diameter to the fourth power. This is then divided by 64. By necessity some measurements are generalized or idealized, such as the average diameter. The shear load on the joint is calculated by dividing the weight of the arm (force) by the area of the joint surface, which for a 15.24 cm (6 in.) surface is A = pr2 or 182 cm2(28 in. 2).For calculation B: Center of gravity is that point in the mass of the segment where all forces act upon the mass equally. It is a point at which the arm could be supported or suspended in perfect balance. The diameter used in this stress calculation is the diameter of the joint, since that is where the stress we are interested in is. The diameter used to calculate the shear load discussed above is the diameter of the arm segment at the joint interface. Fy is equal to the weight of the arm or force. In this calculation a/(maximum tension) and b/ (maximum compression) are equal.
3. A 17% solution was chosen for samples 6-9, rather than the 22% solution used for the shear test specimens, since this has been commonly used in the Getty laboratory as a barrier coating. The authors are confident that this 5% concentration difference had no substantial effect upon the results reported.
4. Stress is computed by dividing the load at failure by the area that is resisting the load. This is the average stress. If the stress distribution is perfectly uniform, the average stress is the same as the stress at all locations in the joint. Failure occurs when the stress exceeds the maximum that the material can withstand. If the stress distribution is nonuniform, failure still occurs when the highest stress anywhere in the joint reaches the failure stress limit. But in the case of nonuniform stress distribution, the average stress that is computed will be lower than the actual maximum stress that precipitated the failure. Therefore, in most cases, the reported failure stress (average stress) will be lower than the actual failure stress (local stress), due to nonuniform distribution of the stress.
5. Horie (1987) points out that the value of the measured glass transition temperature can be lowered considerably by increasing the time during which the force is applied. He gives an example of a polymer with a 30�C (86�F) Tg, but when measured over a period of 1.2 years with continual stress, the Tg lowers to 12�C (53.6�F) (Horie 1987). This finding has significant implications for structural joints that are meant to be serviceable for long terms.
ACKNOWLEDGEMENTS
The authors would like to thank the following people for their valuable assistance and involvement: Martha Simpson for her initial work on the study of B-72 barriers during her internship in the Department of Antiquities Conservation at the J. Paul Getty Museum; Will Thornton, mount maker in the Department of Antiquities Conservation, J. Paul Getty Museum, for preparing the aluminum holding fixtures used in the tensile tests; Bill Ginell and Michael Schilling of the Getty Conservation Institute, and Charles Selwitz, consultant and chemist, for their guidance, review of the manuscript and many helpful insights; and Narayan Khandekar for his invaluable advice regarding UV fluorescence microscopy while an associate scientist at the Getty Conservation Institute. We are also grateful to our respective spouses for giving up numerous weekends so that we might play with marble blocks.
REFERENCES
Akemi Plastics Inc.1999. Product information. Eaton Rapids, Mich.
Axson North America Inc.1999. Product information. Eaton Rapids, Mich.
Bone, L.1999. Personal communication. Fine Arts Museums of San Francisco, San Francisco, Calif.
Bradley, S.1984. Strength testing of adhesives and consolidants for conservation purposes. In Adhesives and consolidants, ed.N. S.Brommelle et al. London: International Institute for Conservation of Historic and Artistic Works. 22–25.
Bruno, L.1999. Personal communication. Brooklyn Museum of Art, Brooklyn, N.Y..
Byrne, G.1984. Adhesive formulations manipulated by the addition of fumed colloidal silica. In Adhesives and consolidants, ed.N. S.Brommelle et al. London: International Institute for Conservation of Historic and Artistic Works. 78–80.
Christman, B.1999. Personal communication. Cleveland Museum of Art, Cleveland, Ohio.
Ciba Specialty Chemicals Inc.1999. Araldite adhesive selector guide. East Lansing, Mich.: Ciba Specialty Chemicals Inc.
Clydesdale, A.1998. Chemicals in conservation: A guide to possible hazards and safe use. Edinburgh, Scotland: Conservation Bureau, Scottish Development Agency, Scottish Society for Conservation and Restoration.
Crist, B.1993. Plastic deformation of polymers. In Materials science and technology: A comprehensive treatment. Vol. 12,Structure and properties of polymers, ed. E. L.Thomas. New York: VCH Publishers. 427–67.
Down, J.1984. Adhesives testing at the Canadian Conservation Institute, past and future. In Adhesives and consolidants, ed.N. S.Brommelle et al. London: International Institute for Conservation of Historic and Artistic Works.18–21.
Down, J. L., J.MacDonald, J.Tetreault, and R. S.Williams. 1996. Adhesive testing at the Canadian Conservation Institute, past and future. Studies in Conservation41:19–44.
Garland, K., and J.Rogers. 1995. The disassembly and re-assembly of an Egyptian limestone sculpture. Studies in Conservation40:1–9.
Griffin, P. S., M. R.Fenn, and H. F.Beaubien. 1983. A preventive conservation case study: The stabilization of ceramic vessels at Harappa, Pakistan.. Pakistan Archaeology28:253–71.
Hansen, E.1994. The effects of solvent quality on some properties of thermoplastic amorphous polymers used in conservation. In Materials issues in art and archaeology IV, ed.P. B.Vandiver et al. Pittsburgh: Materials Research Society. 807–12.
Horie, C. V.1987. Materials for conservation. London: Butterworths.
Koob, S. P.1986. The use of Paraloid B-72 as an adhesive: Its application for archaeological ceramics and other materials. Studies in Conservation31:7–14.
Luskin, L. S.1983-84. Acrylic. In Modern plastics encyclopedia. ed.W.Kaplan. New York: McGraw-Hill. 14.
Marble Enterprises. 1999. Product information. Houston, Tex.
Oberg, E., FranklinD. Jones, and HolbrookL. Horton. 1988. Machinery's hand book.. New York: Industrial Press.
Pocius, A. V.1997. Adhesion and adhesives technology.. Munich, Germany: Hanser.
Schilling, M. R.1989. The glass transition of materials used in conservation. Studies in Conservation34:110–16.
Schmidt-Rohr, K., and R.Richert. 1999. Molecular dynamics. www.mpip_mainz.mpg.de/documents/projects/sr_ri.html.
Selwitz, C. n.d. The use of acrylic polymers for consolidation and preservation. Getty Conservation Institute, Los Angeles. (In production)
Skeist, I., ed. 1977. Handbook of adhesives. 2d ed.New York: Van Nostrand Reinhold.
Sullivan, J. B., and GaryR. Krieger. 1992. Hazardous materials toxicology. Baltimore: Williams and Wilkins.
Walker Zanger Inc. 1999. Product information. Sun Valley, Calif.
SOURCES OF MATERIALSAkemi Marmokitt 1000(an unsaturated polyester containing styrene monomer and fumed silica, catalyzed using High Speed Hardening Paste, a benzoyl peroxidebased catalyst) Akemi Plastics Inc. P.O. Box 40 Eaton Rapids, Mich. 48829 Sivic marbleWalker Zanger Inc. 8901 Bradley Ave. Sun Valley, Calif. 91352 B-72 adhesiveHMG (H. Marcel Guest Ltd.) Riverside Works Manchester M10 7RU UK Araldite AY 103(a modified epoxy used in this case with HY 991, a polyamine hardener) Ciba Polymers Duxford Cambridge UK Paraloid B-72Rohm and Haas Co. Philadelphia, Pa. 19105
AUTHOR INFORMATION
JERRY PODANY received his M.F.A. from Claremont Colleges, Claremont, California, in 1978 and a certificate in archaeological conservation from the Institute of Archaeology, University of London, in 1982. He has been employed in the Department of Antiquities Conservation at the J. Paul Getty Museum since 1978 and has served as the head of that department since 1985. He is adjunct professor in the Museum Studies Program at the University of Southern California. He has a particular interest in the reassembly methodologies for large-scale sculpture, on-site conservation, and protection of collections and monuments from seismic risks by the application of engineering principles to conservation practice. He has been an AIC Fellow since 1998. Address: J. Paul Getty Museum, Department of Antiquities Conservation, 1200 Getty Center Drive, Suite 1000, Los Angeles, Calif. 90049-1687. E-mail: JPodany@getty.edu
KATHLEEN M. GARLAND has a B.A. in art history from Brown University (1981) and an M.A. in conservation from the State University of New York, Cooperstown (1985). She had an internship in sculpture conservation at the Victoria and Albert Museum, London, and served as senior sculpture conservator for the National Trust for Great Britain, 1986-89. Currently, she is conservator of objects, Nelson-Atkins Museum of Art, Kansas City, Missouri. She is an AIC Fellow. Address: Conservation Department, Nelson-Atkins Museum of Art, 4525 Oak St., Kansas City, Mo. 64111. E-mail: Kgarland@nelson-atkins.org
WILLIAM R. FREEMAN has a B.S. in mechanical engineering, University of Florida (1973), and a master of engineering in mechanical engineering, University of Florida (1975). He was a machinery engineer at Union Carbide Technical Center, 1975-78, and since 1979, he has been staff engineer at Allied Signal Federal Manufacturing and Technologies, Kansas City, Missouri. The simulation of physical phenomena by computer has been a specialty since his graduate research. He currently utilizes a world-class dedicated SGI/Cray supercomputer to simulate a wide range of mechanical phenomena for the U.S. Department of Energy utilizing both implicit and explicit finite element methods. He is author of several papers on computer modeling, advanced simulation techniques, and bioengineering topics. He provides engineering assistance for a number of small businesses and nonprofit organizations (such as the Nelson-Atkins Museum of Art and J. Paul Getty Museum) supported under DOE's Technical Assistance Program. Address: Allied Signal FM&T, D/ME1, MC 1C41, 2000 E. 95th St., Kansas City, Mo. 64131. E-mail: bfreeman@kcp.com
JOE ROGERS has been a staff member of the Nelson-Atkins Museum of Art since 1983. He was the conservation assistant in the Paintings Conservation Department until 1988 and is currently the objects conservation associate. He is interested in the treatment of furniture and large sculpture. Address as for Garland. E-mail: conservation@nelson-atkins.org Received for review January 27, 2000. Revised manuscript received July 25, 2000. Accepted for publication September 11, 2000.
Section Index |