PERMANENCY OF REPROGRAPHIC IMAGES ON POLYESTER FILM
HANNA SZCZEPANOWSKA, & WAYNE WILSON
6 6. LABORATORY TESTING AND EXAMINATION METHODOLOGY
The examination of samples of the records was conducted in three stages.
- Stage I
A visual assessment of the records to determine their condition and choose representative samples.Microscopic examination of coatings and imaging techniques.
- Stage IIExposure of samples to various environmental conditions, including:
98% relative humidity in a humidity chamber.UV light emitted by a Blacklite Blue bulb (F15T8-BLB, Sylvania, 356nm).Exposure to ambient daylight through external window.
- Stage III
Microscopic examination and analysis of exposed samples.
In one set of tests, the methodology was designed to replicate a realistic situation in which the records might be housed. In another set of tests, the methodology replicated exposure to the high humidity level characteristic of extreme environmental conditions. High humidity occurs during natural disasters, such as flooding or fire recovery. The methodology was designed to reveal what alterations occur to records during their exposure to high humidity and how much time passes before the alterations are noticeable. Other methods were based on traditional film-testing standards, such as ANSI standards (ANSI 1989; 1992), and adapted from the film-manufacturing industry.
Microscopic analysis of the support, polyester film, coatings, and images and their structure was carried out at 400x. To confirm that the damage was associated with the coating layers rather than with the film itself, the layers were sequentially removed from both sides of the film. The purpose of the testing was threefold: (1) to determine if the film base was indeed polyester; (2) to establish a relationship between the imaging technology and the type of damage; and (3) to determine the effect of extreme environmental conditions on coatings and film.
Test samples approximately 0.5 cm wide and 12 cm long were removed from the margins and central portions of the records designated for disposal. The samples were selected in such a way as to represent various types of damage and diversity within each type. For example, the discoloration on the margins and in the centers varied in color and intensity.
6.1 6.1 FILM BASE ANALYSIS
To determine if all the tested samples were indeed produced on polyester-base film, several tests were performed. The film samples were prepared for examination by gradual removal of emulsions and backing layers, mechanically and with solvents, to reveal the film base. The analysis was carried out at DuPont Testing Laboratory, Florence, South Carolina, and the Johns Hopkins University Instrumentation Laboratory, Baltimore, Maryland. The base film was analyzed using a Nanometrics NanoSpec 20IR microspectrophotometer (DuPont) and a Fourier transform infrared spectrometer (FTIR), Perkin-Elmer 1600 (JHU), to identify the polymer type. As illustrated in figure 11, the resulting spectra for the cleaned (without coatings) base film were compared with a reference infrared spectrum for a known sample of polyester film. Four strong absorption peaks marked on the spectrum (see fig. 12) are the characteristic peaks for the PET (polyester film) sample. The same characteristics were found in all samples examined, confirming that all tested records were created on polyester film.
Fig. 11.
FTIR spectra of Mylar film as the coating is gradually removed
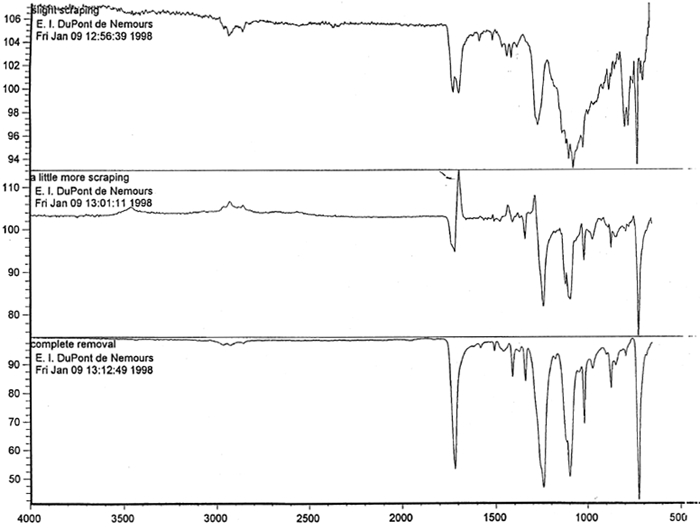 |
Fig. 12.
FTIR spectrum of sample from which both coatings were removed compared with reference spectrum of Mylar polyester film type 92A from the archives of the DuPont Films Enterprise
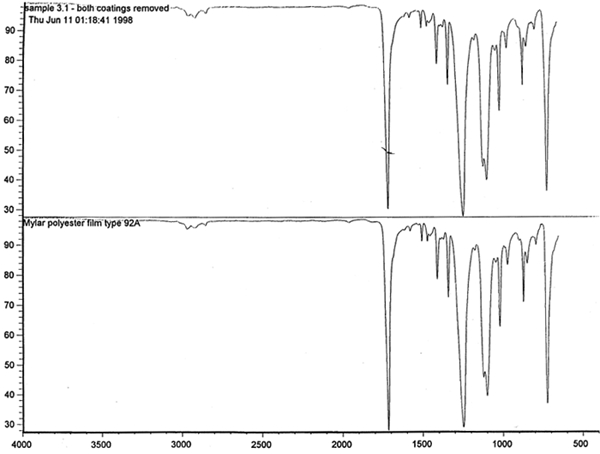 |
Spectral analysis of film samples collected with FTIR illustrate the reading of the Mylar spectrum while the coating is being gradually removed (see fig. 11). Specific details are obscured when the intact film is examined; a gradual removal of the coating from one surface allows the nature of the base film to emerge. With the complete removal of the coating, the characteristics of the infrared spectrum for polyethylene terephthalate may be seen. The characteristic peaks for polyethylene terephthalate are a single strong absorption peak at 1,715 cm−1 and 1,247 cm−1 and double strong absorption peaks at 1,122 cm−1 and 1,099 cm−1.
Figure 12 is a spectrum of a known film sample (a common grade of Mylar polyester film) provided for comparison with the sample film where both coatings were removed. Clearly, both films are made of the same material.
6.2 6.2 COATING ANALYSIS
Two samples of the records that varied most dramatically in discoloration (brown and purple) were examined to determine which part of the structure was responsible for discoloration: film or coatings. The coatings were removed one at a time from each side and examined at 10x. Once all coatings were removed, the base film had no discernible color, indicating that the discoloration originated in the coatings.
To establish which of the two coatings, imaged or reverse, was discolored, additional samples of 15 records were tested. Each side was examined separately after the coating was removed. In all cases, the discoloration was restricted to the image side. To determine which component of the imaged film was responsible for the discoloration, coatings were removed from each side separately. Coatings that were affected by water typically were gelatins. Coatings that dissolved in other mild solvents usually indicated that other organic binders (such as acrylics, polyurethanes, polyesters, and others) were used in the process of film preparation.
The portions of the sample not subjected to solvents for the removal of the coatings were analyzed instrumentally, both with FTIR (to identify organic compounds) and with scanning electron microscopy (SEM)/x-ray multichannel scaling (for elemental analysis).
The imaging layer of representative samples was examined with SEM, using JOEL model JSM-T300. The spectral analysis was carried out with a Nicolet Nic-Plan infrared microscope, attached to a Magna-IR 560 spectrophotometer, at the DuPont Laboratory.
The elements found in tested samples were (1) silver and chlorine, which might indicate silver-base chemistry but does not preclude diazo technology; (2) titanium, which most likely comes from frosting buildup; and (3) silicon, most likely a component of a slip additive and/or frosting.
Figure 13 illustrates an FTIR reflectance spectrum for a typical binder system found in the image-sided coating on an archival record that had discolored. While it is not possible to identify the exact resin used, it is very similar to several cellulosic resins found in the OMNIC reference library. Cellulosic resins are commonly used in imaging applications. (The OMNIC reference library is a collection of optional software including Aldrich Solvents Spectral Library, Polymer Additives and Plasticizer Spectral Library, and Coatings Technology Library. All are used as a resource in comparative studies of spectra of various chemicals.)
Fig. 13.
FTIR reflectance spectra of a discolored coating of a cellulose acetate polymer and various cellulosic resins for comparison. Cellulosic resins are frequently found in imaging applications.
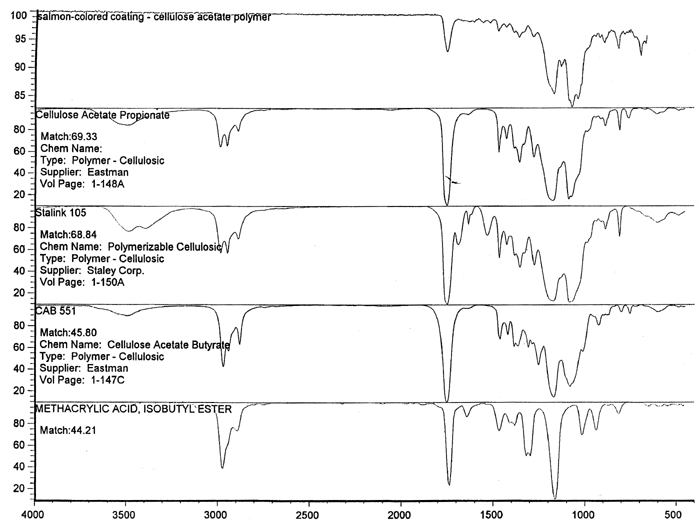 |
Figure 14 shows three FTIR spectra for the three components found in the salmon-colored sample. These components are the frost coating, the underlying film, and the salmon-colored imaged coating. Comparison of the frosted coating to reference spectra permits the identification of the filler as some form of silica, such as silicon dioxide, a major component of sand (fig. 15). The binder used for the imaged coating can be tentatively identified as a cellulosic resin or perhaps an acrylic.
Fig. 14.
FTIR spectra of the three components found in the salmon-colored sample
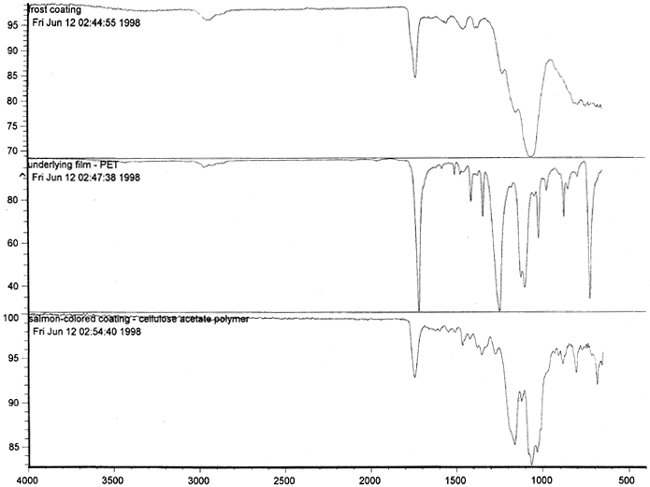 |
Fig. 15.
FTIR spectrum of the frost coating compared with several reference spectra
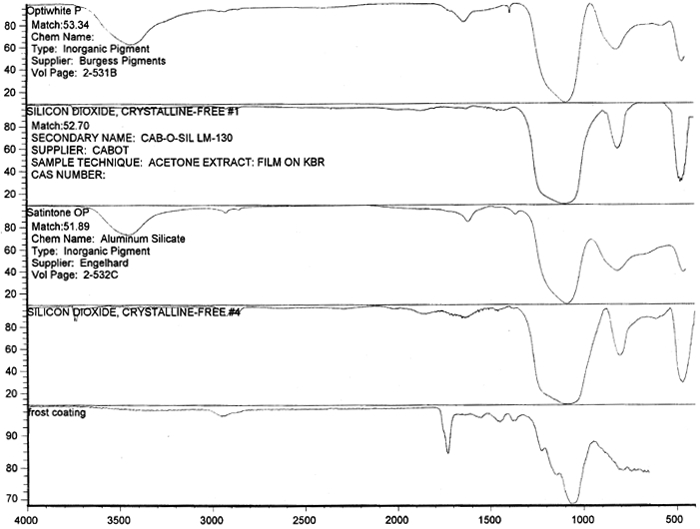 |
6.3 6.3 EFFECT OF EXPOSURE TO HIGH HUMIDITY (98% RH)
Under normal circumstances in a controlled storage environment, the records should never be exposed to severe environmental conditions, such as 98% humidity. Yet, such conditions may occur during shipment or as a result of a fire rescue operation.
The samples of 15 records on polyester film representing various types of damage were placed in a glass laboratory jar so that each sample was freely exposed to the surrounding air and separated from contact with other samples. The samples were exposed for 7, 14, and 21 days to 98% relative humidity. The glass container was placed in a dark room at an average ambient room temperature of 24�C.
The surface topography of the samples before and after exposure to high RH was examined with a Nikon Optiphot microscope equipped with differential interference optics (DIC) to highlight the surface characteristics.
6.4 6.4 SUMMARY OF RESULTS
At 7 days' exposure, cracking began to show in the coating of discolored samples. After 14 days' exposure, there was severe cracking of discolored coatings. At 21 days' exposure, the severity of cracks did not increase, but the image was affected. It showed characteristics of dissolved ink and smudged when touched. Some of the samples curled and adhered to each other as a result of the antiblocking agent's losing its stability. Attempts to separate the adhered samples resulted in emulsion splitting (fig. 16). The diazo layers cracked in a hairlike (fig. 17) or parallel pattern (fig. 18). The cracking of the gelatin-coated portion radiated from the photosensitive particle centers.
Fig. 16.
Discolored record showing emulsion splitting and image smudging
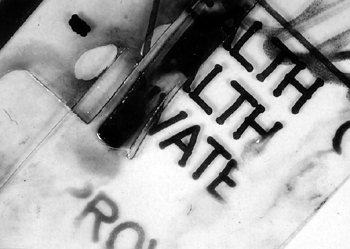 |
Fig. 17.
Hairlike cracking of imaged emulsion emanates from swelling of emulsion grains
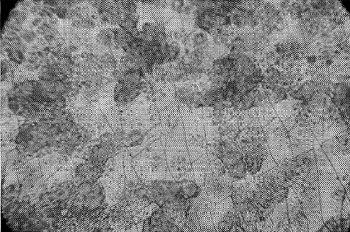 |
Fig. 18.
Parallel cracking of imaged emulsion. Record date: 1992
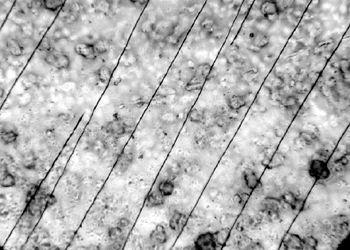 |
Based on the microscopic examination of the crack pattern, it appeared that in one case the particles that were photosensitive expanded faster than the emulsion, becoming centers of each crack. In another example, the particles changed color and gathered in clusters between parallel hairlike cracks.
6.5 6.5 EXPOSURE TO VISIBLE AMBIENT LIGHT AND UV LIGHT
The samples were exposed to visible ambient light, with moderate exposure to sunlight. The samples also were exposed for two and three weeks continuously to UV light emitted from a Blacklite Blue bulb (F15T8-BLB, Sylvania, 356nm). Microscopic examination of the coatings on 15 samples after each period of exposure did not reveal any appreciable changes. Similarly, a spectral FTIR analysis of the exposed samples did not indicate any changes.
Product information on the polyester film indicated that exposure for 500 hours (approximately three weeks) to a UV light renders the product brittle. The exposure level of UV light was compared to the light levels of a bright sun in Arizona. Degradation of the film starts at the surface, and the depth of degradation increases with exposure time. For this reason, heavier gauges of film last longer.
|