ABILITY OF TEXTILE COVERS TO PROTECT ARTIFACTS FROM ULTRAVIOLET RADIATION
NANCY KERR, LINDA CAPJACK, & ROBERT FEDOSEJEVS
ABSTRACT—ABSTRACT—Textiles are an effective, reusable, and inexpensive means of protecting museum objects from ultraviolet radiation (UV), visible light, and dust. To determine how well fabrics screen objects from the damaging effects of ultraviolet radiation, a selection of fabrics varying in fiber content, structure, and color were characterized. The UV transmission in the 280–380 nm range was recorded for each fabric with a spectrophotometer equipped with an integrating sphere and a fluorescence filter. Results showed that the UV blockage varied from a low of 61% (Cerex spunbonded nylon) to a high of 99.9% (black nylon/spandex knit). Unbleached muslin blocked more UV than bleached muslin. The fabric characteristics most affecting UV transmission were mass, thickness, and color.
TITRE—Les housses en tissus et leur degr� de protection contre les rayons ultraviolets. R�SUM�—Les housses en tissus ont l'avantage d'�tre efficaces, r�utilisables et �conomiques pour prot�ger les objets de mus�e contre les rayons ultraviolets, la lumi�re visible et la poussi�re. Cette �tude vise � mesurer � quel degr� divers tissus r�ussissent � bloquer les rayons ultraviolets, lesquels sont nocifs pour les objets de mus�e. Divers tissus de composition, structure et couleur vari�es ont �t� �valu�s. La quantit� de rayons ultraviolets entre 280 et 380 nm que chaque tissu laisse passer a �t� mesur�e � l'aide d'un sprectrophotom�tre dot� d'une sph�re d'int�gration et d'un filtre de fluorescence. Les essais d�montrent que la quantit� de rayons ultraviolets que les tissus bloquent varie entre seulement 61% dans le cas d'un nylon non-tiss� de marque Cerex et 99,9 % dans le cas d'un jersey noir en nylon et spandex. La mousseline de couleur �crue bloque mieux les rayons ultraviolets que celle qui a �t� blanchie. La masse du tissu par unit� de volume, son �paisseur et sa couleur sont les caract�ristiques qui affectent le plus la transmission des rayons ultraviolets.
TITULO—Capacidad de coberturas textiles para proteger artefactos de la radiaci�n ultravioleta. RES�MEN—Los textiles constituyen un medio efectivo, que se puede usar muchas veces y econ�mico para proteger objetos en museos de la radiaci�n ultravioleta (UV), de la luz visible y del polvo. Para determinar cu�n efectivamente las telas protegen a los objetos de los efectos de la radiaci�n ultravioleta, se caracteriz� una selecci�n de muestras con diferentes contenidos de fibra, diferente estructura y color. Se registr� la trasmitancia UV de cada tela en el rango comprendido entre 280 y 380 nm usando un espectrofot�metro equipado con una esfera integradora y un filtro de flourescencia. Los resultados mostraron que el bloqueo de la radiaci�n UV var�a entre un m�nimo de 61% (nylon Cerex no tejido) y un m�ximo de 99.9% (nylon negro/ tejido spandex). La muselina no blanqueada bloque� m�s efectivamente la radiaci�n UV que la muselina blanqueada. Las caracter�sticas de las telas que m�s afectaron la trasmitancia fueron su masa, grosor y color.
1 1. INTRODUCTION
Among the many agents that damage museum objects, light is regarded as one of the most pervasive and harmful. The UV region is noteworthy because many materials absorb light within this region. The light-absorbing chromophores they contain often enter into or stimulate undesirable reactions such as photochemical oxidations and reductions and the cleavage and formation of bonds (Reinert et al. 1994). All organic objects are at risk when exposed to light, including objects made from textiles, paper, wood, parchment, leather, and feathers. Because the action of light is cumulative, reducing the illuminance and exposure time and screening out the most harmful UV rays may slow light damage to objects. For storage areas, Garry Thomson (1986) suggests that a good UV filter should transmit less than 1% of the incident radiation between 320 and 380 nm. Fabric covers are sometimes used to protect objects from UV, visible light, and dust in conservation laboratories and museums. Black textile covers are used in the textile conservation laboratory at the Metropolitan Museum of Art. At the Isabella Stewart Gardner Museum in Boston, decorative textiles cover many glass display cases, and sheer fabrics on the windows act as light screens. Fabric covers on display cases are moved aside by visitors when they want to view the objects. In historic homes, textile covers protect upholstered furniture when visitors are absent. Window blinds and draperies are used to control the illuminance because window glass filters some but not all UV radiation between 300 and 400 nm (Thomson 1986).
Although textile covers are routinely used for protection against damage by light, how well various fabrics screen UV radiation has not been reported in the conservation literature. The principal research objective of the present study was to determine how effectively various textiles and tissues typical of those used for this purpose in a conservation laboratory or museum block the transmission of UV radiation. A second objective was to measure the fiber, yarn, and fabric characteristics that contribute to the blocking of UV radiation and to determine the relative importance of each in that regard.
2 2. EXPERIMENTAL
2.1 2.1 FABRICS
A variety of fabrics, both woven and nonwoven, was selected for this study. Most undyed fabrics were obtained from a commercial source. Woven and knitted fabrics made of 100% cotton, 65/35 polyester/cotton, 50/50 polyester/cotton, and 87/13 nylon spandex were purchased locally. Nonwoven textiles included Cerex spunbonded (SB) nylon, Reemay spunbonded polyester, and Tyvek spunbonded polypropylene. For comparison with the synthetic nonwovens, an acid-free tissue was also included. Fabrics were tested as received from the suppliers.
2.2 2.2 METHODS
Fabrics were characterized by fiber type, fabric mass, fabric count, thickness, level of delustering, structure, and cover. Fiber type was confirmed through microscopic analysis and chemical solubility tests (American Association of Textile Chemists and Colorists 1996). Fabric structure was identified as woven, knitted, or spunbonded. Canadian General Standards Board test methods (1990) were used to determine fabric weight, the mass per unit area (g/m2), fabric count (the number of yarns/cm or wales and courses/cm), and fabric thickness (Custom Scientific thickness tester [CS-55-225], foot of 28.7 mm diameter, pressure of 1 kPa). Level of delustering was determined qualitatively by examining the appearance of manufactured fibers under a transmitted-light microscope at 400x and estimating the relative amount of pigment in specimens by comparison with photomicrographs in AATCC (1996) Test Method 20–1990 (Fiber Analysis: Qualitative). Fibers classified as bright (B) had little or no titanium dioxide (TiO2). Semidull fibers (S-D) were lightly pigmented (approximately 0.3% by weight TiO2) and dull (D) fibers were highly pigmented (approximately 2% by weight TiO2) (fig. 1). Titanium dioxide is added as a fine white powder to reduce the shine of manufactured fibers (Saunders 1988).
Fig. 1.
Relative amount of TiO2 pigment in fibers (left to right): bright, semidull, and dull (after AATCC [1996] method 20, figs. 35, 24, and 33, respectively)
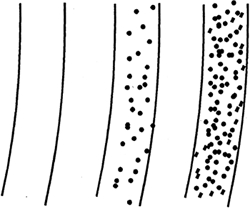 |
2.2.1 2.2.1 Fabric Cover
Fabric cover, defined as the percentage of a given fabric area covered by yarns or fibers, was determined by image analysis. A microscope with a 4x objective magnified the fabric, and a video camera resolved the image, which was digitized and then processed by a computer. The video image consisted of 256 x 256 pixels, each having a value between 0 and 255 representing the brightness of that pixel. For each fabric, the illumination was adjusted such that the peak pixel values, corresponding to open areas in the fabric, had an average value of approximately 250. A cutoff intensity value of 125 was used to separate the pixels representing open areas from those representing areas covered by yarns or fibers. The number of pixels falling above and below this cut-off value was determined and then the percentage of covered area was calculated. Reported cover percentages are based on the average of five images per fabric. The choice of an intensity value of 125 to define the edge of the fibers is based on the assumption that the transmission of light through the fibers themselves is negligible, which is not always the case. The interlaced structure of the fibers results in only a fraction of the fibers observed in a given microscope image being in sharp focus with clearly defined edges. Fibers that are out of focus will have blurred edges, making it difficult to precisely determine the edge position. Based on these limitations, the estimated accuracy of the cover measurement was on the order of several percent due to dependence on the choice of the threshold value separating dark from light pixels.
2.2.2 2.2.2 UV Transmission
UV transmission through fabrics selected for the study was measured on a Varian Cary 2415 UV-Vis-NIR spectrophotometer fitted with an integrating sphere to collect forward-scattered and transmitted light. The wavelength range recorded was 280–380 nm, and a monochromator filtered radiation to a 2 nm bandwidth. Fluorescence was eliminated by means of a 3-mm-thick UG-11 fluorescence-blocking filter adapted for the spectrophotometer. From each sample, five random specimens were cut. Each woven specimen had different warp and weft yarns, and each knitted specimen had different lengthwise ribs and crosswise courses. For the UV transmission measurements, four specimens of each fabric were glued on washers with a diameter of 5 cm and a circular opening of 2 cm. The UV transmission was calculated by averaging the measured values over the range of 280–380 nm. Davis (1995) determined that normally four specimens per sample must be scanned to ensure that the estimated mean UV transmission of the sample will have a standard error no greater than 5% of the mean.
3 3. RESULTS AND DISCUSSION
When incident radiation contacts a fabric (fig. 2), part of that radiation is scattered from the surface and the remainder is absorbed by or penetrates through the fabric. A fraction of the radiation passes through the fibers and spaces between the yarns. The absorbed radiation is taken up by the chromophores in the fibers as well as by other materials present (dyes, delustrants, optical brighteners, finishes). A fabric's ability to protect museum objects or items from ultraviolet light in conservation laboratories, historic houses, storage areas, or limited-view exhibits can be increased by selecting fibers, dyes, and finishes that have excellent absorption of UV radiation. The effect of a variety of fiber types, fabric constructions, dye, and pigment on the transmission of UV is reported in table 1. Fabrics 1 to 9 in table 1 vary in fiber content, but all are undyed, plain-weave fabrics made from spun yarns. It should be noted that the UV transmission values represent the “worst case” or maximum values because they are obtained by passing UV rays in a direction perpendicular to the plane of the cloth. Incident light during use may not be perpendicular to the fabric surface, but may contact it at an angle, thereby increasing the scattering and effective cover.
Table 1. UV Transmission and Characteristics of Fabrics, Spunbonded Webs, Acid–free Tissue
Fiber Type | Fabric Descriptiona | Massb (g/m2) | Fabric ThicknessbC (mm) | Coverb (%) | % UV Transmissionbd 280–380 nm |
Undyed Plain Wegve Fabrics, Spun Yarns |
1. Cotton | unbleached | 160 | 0.46 | 98 | 6 |
2. Cotton | unbleached | 117 | 0.29 | 90 | 7 |
3. Cotton | blsached | 106 | 0.28 | 93 | 27 |
4. Linen | blsached | 107 | 0.26 | 77 | 19 |
5. Rayon | bright | 140 | 0.33 | 92 | 23 |
6. Wool | unbleached | 118 | 0.37 | 88 | 16 |
7. Acrylic | semidull | 131 | 0.41 | 89 | 15 |
8. Polyester | semidull | 133 | 0.35 | 90 | 12 |
9. Nylon | semidull | 125 | 0.36 | 86 | 22 |
Spunbonded Webs and Acid-Free Tissue |
10. Cerex nylon | bright | 53 | 0.14 | 83 | 39 |
11. Reemay polyester | semidull | 45 | 0.24 | 82 | 20 |
12. Tyvek polypropylene | dull | 42 | 0.18 | 98 | 2 |
13. Acid-free tissue | N/A | 19 | 0.05 | 92 | 19 |
Dyed Woven and Knit Fabrics |
14. Print cloth 65/35poly/ cotton | black | 91 | 0.20 | 92 | 6.1 |
15. Cotton muslin | black | 131 | 0.36 | 97 | 1.7 |
16. Double knit 50/50 poly/cotton | black | 192 | 0.62 | 99 | 1.1 |
17. Rib knit cotton | black | 234 | 0.76 | 98 | 0.3 |
18. Stretch knit 87/13 nylon/spandex | black | 210 | 0.60 | 99 | 0.1 |
a a Bright, semidull, and dull refer to amount of the TiO2 delustering agent in manufactured fibers.
bbbb b Mass, cover, thickness, and UV transmission, average of five measurements
C c Thickness under pressure of lkPa
d d Refers to transmission through the yarns and the spaces between
|
Fig. 2.
Process of light transmission through fabric. Radiation incident upon a fabric (Io) is either scattered (Is), absorbed (IA), transmitted through openings (ITO) or transmitted through the fibers (ITF). The total transmission (IT) is equal to the sum of ITF and ITO. Transmitted rays through the fibers and openings may damage the object beneath.
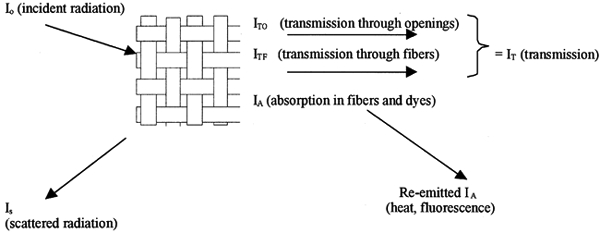 |
3.1 3.1 EFFECT OF FABRIC PARAMETERS ON UV TRANSMISSION
The fiber composition of a fabric affects UV transmission. The poly (ethylene terephthalate) polyester fabric (#8) listed in table 1 had an average transmission of 12% over the 280–380 nm range and was a more effective UV blocker than all but the unbleached cotton muslins. Poly (ethylene terephthalate)-type polyester contains phenyl ester groups that are known to exhibit a very strong UV absorption below 310 nm. Synthetic fibers spun from aliphatic polymers such as nylon, acrylic, and polypropylene block little UV radiation unless they are delustered or dyed. The presence of titanium dioxide delustering pigment in the acrylic, polyester, nylon, Reemay polyester, and Tyvek polypropylene samples contributed to the blocking of UV radiation by these fabrics. Titanium dioxide absorbs about 90% of the incident radiation uniformly across the UV region 280–380 nm (Thomson 1986), and has an absorption maximum at approximately 350 nm (Reinert et al. 1997).
Fabric mass and cover also affect UV transmission. Among the fabrics shown in table 1, those with the highest mass transmitted the least UV radiation; for example, black fabrics nos. 16, 17, and 18 transmitted 1.1, 0.3, and 0.1% UV, respectively. Their low transmission was also a function of their color, thickness, and high cover (98-99%). Other researchers (Berne and Fischer 1980; Pailthorpe 1993) have also noted that high mass and cover resulted in low UV transmission values. Davis (1995) found that the relationship between UV transmission and cover is not as strong as mass. A fabric with high cover does not necessarily block UV rays effectively because some transmission occurs through the fibers or yarns as well as the spaces between them; for example, fabric #10, an undelustered Cerex nonwoven, has a cover of 83% and an open area of 17%. The UV transmission of this fabric is 39%, showing that UV radiation is not only passing through the open area but also penetrating the fibers.
3.2 3.2 UV TRANSMISSION THROUGH TYPICAL MUSEUM FABRICS AND ACID-FREE TISSUE
The UV transmission and other physical characteristics of fabrics frequently used as light and dust covers are presented in table 1, entries 1–3 and 10–15. Cotton is known to be a weak absorber of UV once the natural pigment is removed by bleaching (Pailthorpe 1993; Reinert et al. 1997). This observation is consistent with data obtained for UV transmission by two unbleached muslins (nos. 1 and 2), which, though they differ substantially in mass, had almost identical transmissions of 6% and 7%, respectively. The much greater (27%) UV transmission of fabric no. 3, a bleached cotton muslin, even though it has a cover and mass similar to those of muslin no. 2, is also in agreement with Reinert's suggestion. Figure 3 shows the difference in transmission of UV through bleached, unbleached, and dyed-black cotton muslin.
Fig. 3.
Percent transmission of UV through unbleached cotton muslin, bleached cotton, and black cotton muslin
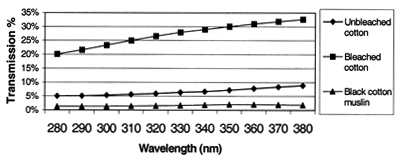 |
Cerex nylon spunbonded web (no. 10) is typically used to separate textiles in storage, to line trays, and to protect items from dust. It provides little protection from UV radiation, however, transmitting about 40% of the incident radiation. Figure 4 shows the variation in transmission of UV with wavelength through Cerex and Reemay fabrics, SB polyester, and acid-free tissue. Other researchers have noted the permeability of aliphatic nylons to UV radiation over the whole UV region (Reinert et al. 1997).
Fig. 4.
Percent transmission of UV through Cerex nylon, Reemay polyester, Tyvek polypropylene, and acid-free tissue
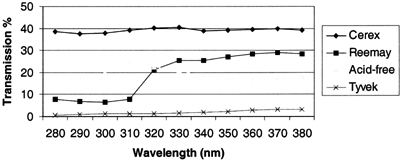 |
Among the spunbonded nonwovens made of nylon, polyester, and polypropylene, the most effective UV screen was the Tyvek SB polypropylene (no. 12), which transmitted 2% of the UV radiation. This spunbonded nonwoven is a water-resistant, flexible material typical of the “synthetic paper” that is used in the conservation laboratory as a cover for rolled textiles in storage, curtains in storage areas, and covers for objects that are undergoing conservation treatments. The reason Tyvek blocks the transmission of UV radiation so well is its fiber content, its high cover (98%), and the heavy pigmentation of the fibers.
3.3 3.3 DYED FABRICS
Our research (Davis 1995; Davis et al. 1997) and that of many others (Pailthorpe 1993; Gies et al. 1994; Pailthorpe 1994; Reinert et al. 1997) has shown that fabrics dyed dark shades or saturated colors rather than pastels are very effective at screening UV radiation. Five black fabrics are shown in table 1 to illustrate this finding. The black fabric that transmitted the most UV (6.1%) was a low-mass, thin 65/35 polyester/cotton fabric (no. 14), and yet it blocked as much UV as the most effective UV-blocking fabric in the undyed group, a heavy unbleached muslin (no. 1). Three of the knitted black fabrics in this group (nos. 16–18) transmitted only 1% of the UV, thereby meeting Thomson's criterion for an effective UV filter (Thomson 1986). Their effectiveness is a combination of color as well as high thickness, mass, and cover, resulting from the knit structure. If black fabrics are used in a conservation laboratory or museum setting to protect objects from light, they should first be tested for colorfastness to crocking and water.
4 4. CONCLUSIONS
If fabric is chosen carefully, textile covers are an inexpensive, reusable, and effective means of reducing the exposure of museum objects to UV radiation. The fabric characteristics that most influence UV transmission are mass, thickness, and color: the higher the mass and thickness and darker or more saturated the color, the less UV radiation transmitted through the fabric. Three fabrics reported in this study met Thomson's (1986) criterion of blocking 99% UV, a black 50/50 polyester/cotton double knit and a black stretch knit of 87% nylon and 13% spandex. Tyvek transmits 2% of the UV radiation, but has the important advantage in the museum context of being both waterproof and much lighter in weight than either the nylon/spandex or the double knit. The natural pigment in unbleached cotton muslin makes it a more effective UV screen than bleached cotton. Spunbonded webs with low cover (Cerex and Reemay) transmit 39% and 20% of the UV, respectively, and so are not useful covers for light-sensitive objects.
ACKNOWLEDGEMENTS
This research was funded in part by the Endowment Fund for the Future at the University of Alberta. The Government of Canada Summer Career Placement program also provided support for two undergraduate students of human ecology. We recognize the significant contributions of Jennifer Moroskat and Tannis Grant to this research project.
REFERENCES
AATCC. 1996. Fiber analysis: Qualitative: Test Method 20–1990. In Technical manual of the AATCC, vol. 69. Research Triangle Park, N.C.: American Association of the Textile Chemists and Colorists. 47–62.
Berne, B., and T.Fischer. 1980. Protective effects of various types of clothing against UV radiation. Acta Dermato-Venereologica60:459–60.
CGSB. 1990. Textile test methods, Can/CGSB-42. Ottawa: Canadian General Standards Board.
Davis, S.1995. Relationship of fiber type, mass, and cover to the sun protection factor of fabrics. Master's thesis, University of Alberta, Edmonton, Alberta, Canada.
Davis, S., L.Capjack, N.Kerr, and R.Fedosejevs. 1997. Clothing as protection from ultraviolet radiation: Which fabric is most effective? International Journal of Dermatology36:374–79.
Gies, H. P., C. R.Roy, G.Elliott, and W.Zongli. 1994. Ultraviolet radiation protection factors for clothing. Health Physics67:131–39.
Pailthorpe, M. T.1993. Textile parameters and sun protection factors. In Proceedings of the Textiles and Sun Protection Conference, ed. M. T. Pailthorpe. Kensington, NSW, Australia: Society of Dyers and Colorists of Australia and New Zealand. 32–53.
Pailthorpe, M. T.1994. Textiles and sun protection: The current situation. Department of Textiles Technology, University of New South Wales, Sydney, NSW. 20–21.
Reinert, G., E.Schmidt, and R.Hilfiker. 1994. Facts about the application of UV-absorbers on textiles. Melliand Textilberichte7–8:E151–E163.
Reinert, G., F. Fuso, R.Hilfiker, and E.Schmidt, E.1997. UV-protecting properties of textile fabrics and their improvement. Textile Chemist and Colorist12:36–43.
Saunders, J. H.1988. Polyamides, fibers. In Encyclopaedia of polymer science and engineering, ed. J. I.Kroschwitz et al.New York: Wiley. 422.
Thomson, G.1986. The museum environment 2d ed. London: Butterworths.
FURTHER READING
Capjack, L., N.Kerr, S.Davis, R.Fedosejevs, K.Hatch, and N.Markee. 1994. Protection of humans from ultraviolet radiation through the use of textiles: A review. Family and Consumer Sciences Research Journal23:198–218.
Hilfiker, R., W.Kaufmann, G.Reinert, and E.Schmidt. 1996. Improving sun protection factors of fabrics by applying UV-absorbers. Textile Research Journal66:61–70.
SOURCES OF MATERIALSIn Table 1, fabrics that were purchased fromTestfabrics, Inc.
P.O. Box 420
Middlesex, N.J. 08846
are listed here with their style numbers: 3. #400 bleached cotton print cloth; 4. #L-61 handkerchief linen; 5. #266 spun viscose rayon challis; 6. #530 worsted wool challis; 7. #981 Spun Creslan acrylic type 31; 9. #361 spun nylon 6,6 Dupont type 200. Cerex spunbonded nylon by MonsantoHTC Laboratories
5575 Casgrin Ave.
Montreal, Quebec H2T 1Y1
Canada Tyvek spunbonded polypropylene Type 1422ABury Media and Supplies Ltd.
B-5-4255 Arbutus St.
Vancouver, British Columbia V6J 4R1
Canada Reemay spunbonded polyester #2014Carr McLean Ltd.
461 Horner Ave.
Toronto, Ontario M8W 4X2
Canada
AUTHOR INFORMATION
NANCY KERR has a B.Sc. in home economics from the University of Guelph, an M.Sc. in clothing and textiles from the University of California at Davis, and a Ph.D. in fiber and polymer science from North Carolina State University. She is a professor in the Department of Human Ecology at the University of Alberta. Her teaching and research focus on textile science and conservation topics. Current projects include UV transmission through textile materials, degradation and stabilization of historic textiles, and industrial hemp. Address: Department of Human Ecology, 302 Human Ecology Building, University of Alberta, Edmonton, Alberta T6G 2N1, Canada
LINDA CAPJACK received her M.Sc. in clothing and textiles from the University of Alberta. She is associate chair and associate professor in the Department of Human Ecology at the University of Alberta. Her research interests include the functional design process and environmental protective clothing, focusing recently on clothing to protect from ultraviolet radiation. She has worked collaboratively with Dr. Nancy Kerr and Dr. Robert Fedosejevs on identifying fabric characteristics that contribute to decreased transmission of UV. Address as for Kerr
ROBERT FEDOSEJEVS received his B.Sc. and Ph.D. degrees from the University of Toronto, Canada, in 1973 and 1979. He subsequently was a research associate at the Max Planck Institute in Germany. He joined the Department of Electrical and Computer Engineering at the University of Alberta as associate professor in 1982 and currently holds the position of C. R. James/MPBT/NSERC Senior Industrial Research Chair in the Application of Laser and Spectroscopic Techniques to the Natural Resources Industry. His research interests include the study of laser-plasma interactions and the application of laser and spectroscopic techniques to the characterization of materials. Address: Department of Electrical and Computing Engineering, 238 Civil/Electrical Engineering Building, University of Alberta. Edmonton, Alberta T6G 2G7, Canada
Received for review July 25, 1999. Revised manuscript received June 1, 2000. Accepted for publication May 16, 2000.
Section Index |