MOISTURE RELATIONSHIPS OF PHOTOGRAPHIC FILM
P.Z. ADELSTEIN, J.-L. BIGOURDAN, & J.M. REILLY
ABSTRACT—The moisture content of photographic film is a critical feature that influences physical properties, mold growth, and chemical stability. It is characterized by the moisture equilibrium curve, which is a plot of the moisture content as a function of the relative humidity. The effect of temperature on the moisture equilibrium curve is the main thrust of this article, which discusses the practical implications of these data for accelerated incubation studies. The temperature effect on the rates of moisture conditioning on film in several practical storage configurations and enclosures is presented. Finally, data are given on the moisture protection afforded by different enclosures, leading to recommendations on the cold storage of photographic film.
TITRE—Recherches sur l'Humidit� des Pellicules Photographiques. R�SUM�—L'humidit� contenue dans une pellicule photographique est une donn�e primordiale, car elle a des effets sur les propri�t�s physiques de ce mat�riau, sur sa stabilit� chimique, et sur le d�veloppement de moisissures. On la r�presente � l'aide de la courbe d'�quilibre de l'humidit�, sur laquelle elle est fonction de l'humidit� relative. Cet article �tudie principalement les effets de la temp�rature sur la courbe d'�quilibre de l'humidit�, illustr�s lors d'exp�rimentations d'incubation acc�l�r�e. Les auteurs d�crivent les effets de la temp�rature sur l'humidit� contenue dans les pellicules en fonction de divers types d'emballages et conditions de stockage. Ils indiquent �galement le degr� de protection contre l'humidit� offert par diff�rents emballages, le r�sultat de leur test les amenant � recommander un stockage des pellicules a basse temp�rature.
T�TULO—Relaciones del contenido de humedad de la pel�cula fotogr�fica. RESUMEN—El contenido de humedad de la pel�cula fotogr�fica es un factor critico que influye sobre las propiedades fisicas, el crecimiento de mohos, y la estabilidad qu�mica. Est� caracterizado por la curva de equilibrio de humedad, la cual es una gr�fica del contenido de humedad como funci�n de la humedad relativa. El efecto de la temperatura sobre la curva de equilibrio de humedad es el punto principal de este art�culo, en donde se discuten las implicaciones pr�cticas de estos datos para estudios de incubaci�n acelerada. El efecto de la temperatura sobre la velocidad de acondicionamiento a la humedad de la pel�cula se presenta en varias configuraciones pr�cticas de almacenamiento y materiales cerrados para dep�sito. Finalmente, se dan datos sobre la protecci�n contra la humedad que ofrecen diferentes contenedores, los cuales llevan a recomendaciones para el almacenamiento en fr�o de la pel�cula fotogr�fica.
1 INTRODUCTION
The moisture content of photographic film is one of the most critical characteristics that determines physical properties and practical behavior (Calhoun 1944). If the moisture content is high, photographic film may be tacky, be susceptible to mold growth, and will have reduced chemical stability (Adelstein et al. 1992b). If the moisture content is low, the stability will be increased, but the film may have susceptibility to static, be brittle, and show high curl; if it has a weak bond, it may exhibit emulsion-base adhesion problems. Dimensional changes as well as distortions that can develop in film rolls (Carver et al. 1943) are strongly influenced by the moisture content.
Moisture content of films has always been evaluated and compared by the moisture equilibrium curve—that is, a plot of the weight percent of water as a function of the relative humidity (Calhoun 1944). Typical plots for the components of photographic products are shown in figure 1. Values for the photographic film laminate lie between the graphs for the emulsion and base layer and are very dependent upon the relative thicknesses of each. As Calhoun (1944) indicated, the important controlling factor in determining the moisture content of film is the relative humidity of the environment and not the absolute humidity. This relationship is identical to the characterization of moisture properties on many diverse materials (Young et al. 1965; Cargocaire Engineering Corp. 1990). However, it must be recognized that the moisture equilibrium curve is not completely independent of the temperature. Studies on grain (Hukill 1965), dry milk (Heldman et al. 1965), cotton (Urquhart and Williams 1924), paper (Ulm 1938), cornstarch (Hughes et al. 1965), and cellulose acetate (Newsome and Sheppard 1932) all show a similar behavior. At constant relative humidity, these materials hold slightly more water as the temperature is lowered. However, the influence of temperature is secondary to the influence of relative humidity and in no way invalidates the usefulness of the moisture equilibrium curve.
Fig. 1.
Typical moisture equilibrium curves for the components of photographic products (Adelstein 1973)
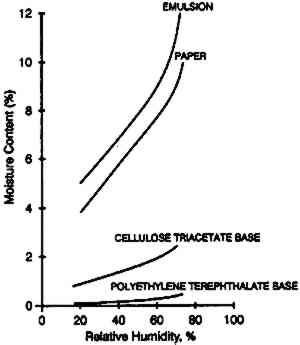 |
A main thrust of this article is a study of the effect of temperature on the moisture equilibrium curves of photographic film and its implications in both storage and laboratory situations. Limited data were reported by Adelstein et al. (1970) on a motion picture film on cellulose triacetate base. It was pointed out that although the moisture content of air at 49�C and 50% RH is 264 grains/lb. dry air and at 7�C and 50% RH is 22 grains/lb. dry air, the water content of this film was very similar despite the tenfold difference in water content of the air.
There have been several more recent studies on the temperature effect since the 1970 publication. Iwano (1994) presented data on gelatin to a subcommittee of the American National Standards Institute (ANSI). A gravimetric procedure was used experimentally. This information, reproduced in figure 2, also shows a relatively low temperature dependence on the percent moisture content.
Fig. 2.
Effect of temperature on moisture equilibrium curves of gelatin (Iwano 1994)
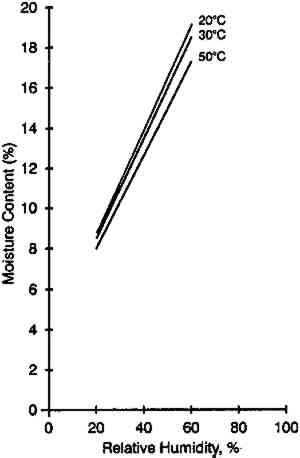 |
McCormick-Goodhart (1994) produced results of a similar study using a different laboratory procedure that was also presented at an ANSI meeting and subsequently published (1995, 1996). He first conditioned photographic-grade gelatin to various moisture equilibrium levels at room temperature. Five moisture levels were studied. They corresponded to an equilibrium humidity at 21�C, which varied from 28 to 73% RH. Subsequently each moisture-conditioned sample was sealed in a tightly sealed pressure cooker that contained a chilled-mirror hygrometer to measure the relative humidity of the air. The temperature of the chamber was varied, and the resulting relative humidity was determined. This experiment produced a series of five moisture content isolines—that is, lines of constant moisture content plotted against relative humidity and temperature. A typical moisture-content isoline is shown in figure 3 for gelatin that contained 14.4 wt% water. From a family of these moisture content isolines, the traditional moisture equilibrium curves were obtained at temperatures ranging from −20�C to 80�C. These data are consistent with the other studies (Adelstein et al. 1970;Iwano 1994) showing a shift to a higher equilibrium relative humidity for a given moisture level as the temperature is raised (fig. 4). However, the magnitude of the temperature effect as measured by McCormick-Goodhart (1994, 1995, 1996) is somewhat greater than that presented in the earlier studies.
The moisture content isolines also revealed additional information about the hygroscopic behavior of gelatin. It can be observed from figure 3 that there was a general linear relationship between the equilibrium relative humidity and the temperature up to a temperature of 40�C. Above this temperature, there was very little change in the equilibrium relative humidity level. The explanation for this behavior is that 40�C is the glass transition temperature (Tg) of this gelatin. (The glass transition temperature is the temperature at which there is a change in the physical characteristics of amorphous materials. Gelatin is glassy in behavior below Tg and rubbery above Tg.) This procedure was used to measure the glass transition temperature, and the values listed by McCormick-Goodhart (1994) in table 1 compare favorably with those previously reported in the literature (Rose 1977).
TABLE 1 MOISTURE PROPERTIES OF PHOTOGRAPHIC GELATIN
This article presents additional data on the temperature effect on the moisture equilibrium curve. However, unlike the work of Iwano (1994) and McCormick-Goodhart (1994, 1995, 1996), who studied the gelatin binder, this study presents information on the complete photographic film on cellulose triacetate base. Iwano interpreted his data as it pertains to the humidity inside sealed bags when used in accelerated incubation tests. McCormick-Goodhart addressed this application as well as implications for the cold storage of photographic film. Both aspects will be discussed here.
A consideration for cold storage of photographic film is the rate of moisture conditioning at low temperatures. Only limited data had been published in this area, and more comprehensive results are given in this article.
A very practical aspect of moisture effects in storage is the moisture protection provided by various types of enclosure materials such as bags, boxes, and cans. Comparisons were made at both room temperature and at −16�C.
2 EXPERIMENTAL RESULTS
2.1 EFFECT OF TEMPERATURE ON MOISTURE EQUILIBRIUM CURVE
The experimental technique used in this investigation was very similar to that employed by McCormick-Goodhart (1994, 1995, 1996). Recent rolls of 100 ft. � 35 mm color motion picture film on .0055 in. cellulose triacetate base were processed according to standard Eastman Color Print ECP-2 processing, then conditioned to five humidities between 20 and 75% RH at 20�C. They were then separately sealed in a 100 ft. metal film can along with a humidity sensor. The sensor was a Vaisala HMP 130Y Series (Humidity and Temperature Transmitter) electrically connected to an outside data logger. Since the volume of film is very large relative to the free air volume, and since air holds very little moisture compared to film, these measurements are at essentially constant moisture content. The temperature was varied between −16 and 60�C. The moisture content of the film was determined by gravimetric analysis, with moisture content being calculated from the film weight after drying the film at 105�C. The moisture content isolines are shown in figure 5. These data do not show the same marked plateau in equilibrium RH with increasing temperature as observed by McCormick-Goodhart (1994, 1995, 1996). However in those previous studies, only gelatin was studied, while in this study data were obtained for the complete film. From the relationships in figure 5, the moisture equilibrium curves in figure 6 were plotted.
Fig. 5.
Moisture content isolines for 35 mm color motion picture film on cellulose triacetate base
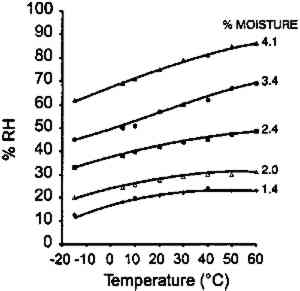 |
Fig. 6.
Effect of temperature on moisture equilibrium curve of 35 mm color motion picture film on cellulose triacetate base
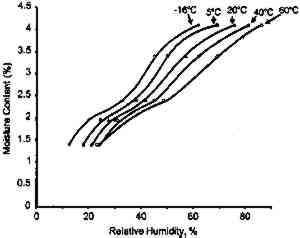 |
These data duplicate the general relationships previously given for the gelatin binder only. The essential feature is the shift to an increase in equilibrium relative humidity for a constant weight percent water with increasing temperature. This finding has been consistent for all studies (Adelstein et al. 1970; Iwano 1994; McCormick-Goodhart 1994, 1995, 1996).
2.2 RATES OF MOISTURE CONDITIONING
A basic consideration of temperature effects on the moisture properties of film is the rate of moisture conditioning. Although several comprehensive studies have been reported on room temperature (Calhoun 1944; Eastman Kodak Co. 1972), only very limited data have been published on subzero temperatures (Adelstein et al. 1970), and these pertained to film as a single sheet. To obtain information on current materials and on film in a variety of practical storage configurations and enclosures, more extensive conditioning studies have been completed. These featured moisture conditioning curves at −16�C for both motion picture roll film and sheet film.
The moisture equilibration rates for the roll films were obtained gravimetrically. Figure 7 illustrates the increase in percent moisture equilibrium expressed in percentage of the total moisture increase after complete equilibrium. The results are given for a 100 ft. � 35 mm motion picture film on .0055 in. cellulose triacetate base, originally conditioned at 21�C and 20% RH. One roll was then placed at 21�C and 50% RH, and the other in a frost-free freezer with air at approximately −16�C and 50% RH. The rolls were not in any enclosure but exposed to the air, with one plane lying on a flat surface. The time to reach 90% moisture equilibrium was 12 days at 21�C and 120 days at −16�C. This tenfold increase in conditioning time was considerably less than the 30X increase observed previously at −23�C for filmstrips (Adelstein et al. 1970). This difference can be attributed to the difference in temperature and film configurations. Since moisture diffusion takes place primarily through the width direction of the film, the data in figure 7 are valid for the larger rolls used in the motion picture industry. The graphs are expressed in percent moisture equilibrium, which is relatively independent of the relative humidity range used experimentally.
Fig. 7.
Rate of moisture conditioning for exposed 35 mm motion picture roll lying on its side. Film initially at 21�C and 20% RH and exposed to 50% RH
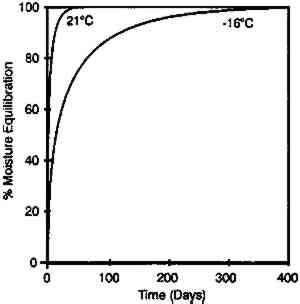 |
The moisture conditioning rate can be very dependent upon the type of film enclosure used. At both temperatures, the equilibration rates given in figure 7 were very similar if the film rolls were in moisture permeable enclosures such as a cardboard box, a vented plastic can with holes to allow escape of deleterious gases, or an open metal can. However, the situation was very different if the film was in an enclosure with limited access to the outside air. Figure 8 is a similar plot for film in a closed metal can. At 21�C, 90% moisture equilibrium was obtained in 3 months, while at −16�C the film had reached only 20% equilibrium after 1 year. Similar tests on plastic cans produced comparable results. However, the equilibration times are very dependent upon the tightness of the seal between the cover and the can.
Fig. 8.
Rate of moisture conditioning for 35 mm color motion picture roll in closed metal can. Film initially at 21�C and 20% RH and exposed to 50% RH
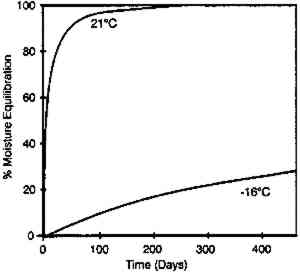 |
Moisture equilibrium studies were also done on a set of 150 (4 � 5 in.) sheet films on .008 in. cellulose acetate butyrate base that were conditioned to 21�C and 20% RH, enclosed in individual paper envelopes and then stored in a cardboard box. This box was then placed in a 21�C and 50% RH environment. Moisture conditioning was followed using an RH sensor that was inserted within the film pack. The inner sheets required 7 days for 90% moisture equilibration (fig. 9). This experiment was repeated using a metal box instead of the cardboard (fig. 10). While the metal box provided more moisture protection than the cardboard, it was still quite inadequate. The 90% equilibration time increased from 7 to 30 days, a difference not significant for extended times of storage.
Fig. 9.
Rate of moisture conditioning for stack of 150 sheet films in cardboard box. Film initially at 21�C and 20% RH and exposed to 50% RH. Measurements made on inner sheet
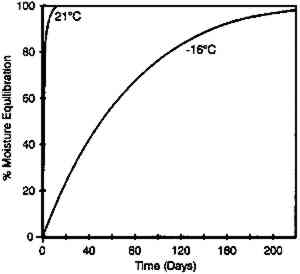 |
Fig. 10.
Rate of moisture conditioning for stack of 150 sheet films in cardboard and metal boxes. Film initially at 21�C and 20% RH and exposed to 21�C and 50% RH. Measurements made on inner sheets
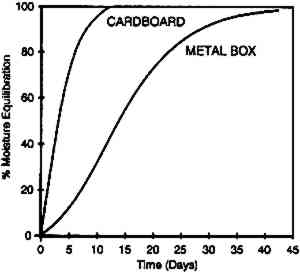 |
The effect of temperature on moisture conditioning was determined by placing the film at 21�C and 20% RH in a frost-free freezer at −16�C and 50% RH. Figure 9 shows that 90% moisture equilibration required 5 months at −16�C, contrasted to 1 week at 21�C, approximately a twentyfold difference. This finding is the same order of magnitude as obtained for the 35 mm motion picture roll film.
The low-temperature moisture conditioning results are replotted in figure 11, expressing the moisture content as the equilibrium RH of the film measured by the RH sensor imbedded within the film stack. The initial drop in RH from 20 to 10% RH is due to the initial temperature decrease, which is very rapid. This effect is best illustrated in figure 12 by the difference in moisture equilibrium curves at 20�C and −16�C. (Although the data shown in figure 12 were obtained on motion picture film on triacetate base rather than acetate butyrate base sheet film, i.e., the behavior is very similar.) The film is initially at point A, and as it is cooled it goes to point B, which is at a lower equilibrium relative humidity. Subsequent moisture conditioning at −16�C and 50% RH moves the film to point C. An essential feature of this plot is that film initially at point A has 1.4% water, and after final moisture conditioning at point C it has 3.5% water. This shift represents a significant increase.
Fig. 11.
Rate of moisture conditioning for stack of 150 sheet films in cardboard box. Film initially at 21�C and 20% RH and exposed to approximately −16�C and 50% RH. Measurements made on inner sheets
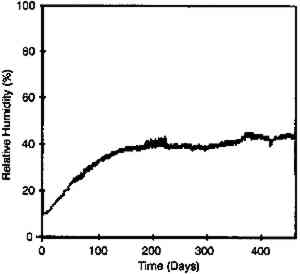 |
Fig. 12.
Moisture equilibrium curve of 35 mm color motion picture film on cellulose triacetate base
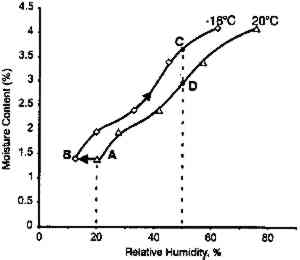 |
If film is just cooled without any change in equilibrium relative humidity it will also hold more water. Consider film at 20�C and 50% RH (point D). If it is cooled to −16�C at the same relative humidity, its water content will increase from 2.9 to 3.5%.
The key conclusion from this phase of the study is that the rate of moisture equilibration at subzero temperature is slow but still appreciable. Moreover, film holds more water at these low temperatures when in equilibrium with the same relative humidity.
2.3 EFFECT OF ENCLOSURES
The data reported in figures 7, 9, 10 and 11 were obtained on film that was completely exposed to the environment or had the very little moisture protection provided by a cardboard box. A very practical question is whether moisture conditioning can be minimized using more effective enclosures.
The effectiveness of enclosures was determined by measuring the weight increase of fresh molecular sieves inside enclosures that were placed in a 21�C and 95% RH environment. Molecular sieves are zeolites composed of metal aluminosilicates, marketed by the Eastman Kodak Co. to act as both a desiccant and a scavenger for acetic acid (Ram et al. 1994). They are sold in Tyvek (spun-bonded polyethylene) envelopes that facilitate handling. In this study their desiccant properties were of interest and are used only as an indicator of the moisture barrier of enclosures.
The molecular sieve envelopes were initially weighed, placed in various enclosures, and reweighed after 2 months at 21�C and 95% RH. Figure 13 illustrates that heat-sealable aluminum foil bags and taped metal film cans provide a reasonable moisture barrier. However, the common low-density 3 mil. polyethylene freezer bags and untaped 35 mm metal film cans do not offer sufficient protection for film storage. These experiments are still in progress in order to obtain the times required for complete moisture conditioning.
Fig. 13.
Moisture increase of molecular sieves in various enclosures after 2 months at 21�C and 95% RH
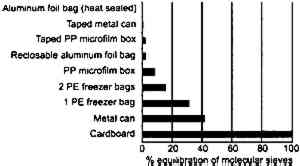 |
When this study was repeated at −16�C (fig. 14), the moisture gain was obviously much less. However, even at these low temperatures, untaped cans or boxes allow appreciable moisture take-up after only 4 months. They would not be recommended for extended long-term storage in an uncontrolled environment but are satisfactory if the humidity is controlled. This point is discussed further in section 3.2.
Fig. 14.
Moisture increase of molecular sieves in various enclosures after 4 months at approximately −16�C and 50% RH
 |
3 DISCUSSION
3.1 IMPLICATIONS FOR ACCELERATED INCUBATION TESTS
The standard method used to study the life expectancy of photographic film is to determine the rate of degradation under accelerated temperature/relative humidity conditions. The results obtained at these severe environments can then be extrapolated to practical storage environments using the Arrhenius treatment of the data (Anderson and Kopperl 1993; Reilly 1995). This approach is valid only if the degradation at elevated temperature proceeds by the same mechanism as at the storage temperature. Over the years this Arrhenius procedure has provided very valuable information and insight on the preservation of photographic materials.
Two experimental techniques have been used in these studies. The first consists of using ovens that are controlled to a constant temperature and relative humidity. However, as already pointed out, the moisture content of the material changes as the temperature changes. The resulting Arrhenius lines are projections made at constant relative humidity rather than at constant moisture content.
The second experimental technique consists of conditioning the samples at a known relative humidity and then sealing them in moisture-proof bags. This procedure ensures that the water content of the materials is constant at the different temperatures. Moreover, any degradation products produced during degradation remain confined, with possible catalytic effects on the reaction rate. However, since the moisture content of materials is dependent not only on the relative humidity but also on temperature, the relative humidity varies with temperature. In some cases incubation in sealed bags can cause the equilibrium relative humidity of the emulsion layer to increase above its glass transition temperature. This effect has resulted in aggregation changes of the dye in a color film and causes a dye loss that does not occur at lower temperatures (Meyer and Bermane 1983). This behavior has led to the recommendation by McCormick-Goodhart (1995) that Arrhenius tests on photographic materials be derived under conditions that are below the glass transition temperature of the gelatin.
This recommendation is correct for some photographic systems, as illustrated by the example cited above. However, it is not believed to be universally true, particularly when the degradation process is chemical and not physical. The glass transition temperatures obtained from moisture content isolines (table 1) were applied to a recent study on the stability of cellulose ester base photographic film (Adelstein et al. 1992a, 1992b) in which the sealed bag technique was used.
Analysis of the cellulose ester base film study was confined to two properties that reflect only characteristics of the emulsion layer. The first is the wet strength of the emulsion that is determined by the weight on a 15 mil. radius sapphire stylus required to rupture the surface when wet. It has been described in the literature as the “emulsion-mushiness test” (Parker and Sugden 1963; ANSI 1992). The second test is the emulsion melting point. It measures the temperature of an aqueous solution in which the emulsion dissolves (ANSI 1964). As the gelatin degrades, both the mushiness and the melting temperature are decreased.
Figure 15 shows Arrhenius plots of wet emulsion strength for a motion picture film. Linear plots were obtained when this material was sealed in moisture-proof bags at 21�C when in moisture equilibrium at both 50 and 60% RH. With the exception of the 50�C and 50% RH point, all data were obtained above the glass transition temperature (see table 1). It is estimated that at 50% RH, the glass transition temperature is approximately 52�C. At this temperature, the Arrhenius plot is still linear, suggesting that the glass transition temperature is not a critical parameter for this property. Figure 16 is a similar graph for a motion picture film from another manufacturer. Again there does not appear to be any discontinuity in the Arrhenius plot as the glass transition temperature is approached.
Fig. 15.
Arrhenius plots for wet emulsion strength of a motion picture film. Film specimens preconditioned to 21�C and heated in sealed bags
 |
Fig. 16.
Arrhenius plots for wet emulsion strength of a motion picture film. Film specimens preconditioned to 21�C and heated in sealed bags
 |
Analysis of the emulsion melting point for the film is given in figure 17. With this property, data are available at 20% RH as well as at higher humidities. It is estimated that 80�C and 20% RH is below but that 90�C and 20% RH, and 100�C and 20% RH, are above the glass transition temperature of gelatin. A linear Arrhenius relationship is still observed.
Fig. 17.
Arrhenius plots for emulsion melting point of a motion picture film. Film specimens preconditioned to 21�C and heated in sealed bags
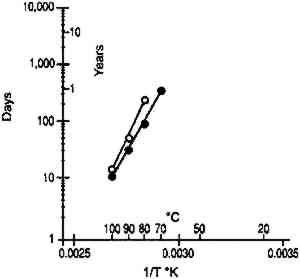 |
This analysis suggests that the change in some physical properties of gelatin at elevated temperatures is not significantly influenced by the glass transition temperature. This is not to imply that the degradation of these properties is not influenced by Tg, but that the effect is not large enough to invalidate the Arrhenius treatment of the data.
3.2 IMPLICATIONS FOR LOW-TEMPERATURE STORAGE
For more than 25 years it has been recognized that low-temperature storage will prolong the image life of chromogenic color films (Adelstein et al. 1970). It will also increase the stability of cellulose triacetate base (Adelstein 1992a, 1992b). This has been the repeated and consistent recommendation since that time (Kach 1978; Wilhelm 1978; Eastman Kodak Co. 1983; ANSI 1993). Two types of low-temperature storage environments have been recommended. For relatively small collections, the use of refrigerators or freezers, specifically frost-free units, are advised (Wilhelm 1978). However, for large collections, prolonging the image life has been accomplished using cold storage vaults that operate at moderate to low relative humidities (Goodrich 1980; Wilhelm 1993). Each will be discussed in turn.
3.2.1 FROST-FREE FREEZERS
The recommended procedure for using frost-free freezers is to seal the photographic material in a vapor-tight package at room temperature prior to putting it into a freezer compartment. It has also been recommended that the photographic contents be first moisture conditioned to about 50% RH before being sealed in the package. However, examination of figure 18 indicates that a moisture conditioning procedure is not necessary unless the room temperature humidity is greater than 60% RH. Film in a moisture-tight, sealed package at 20�C and 60% RH (point A in fig. 18) will have an equilibrium relative humidity around 50% RH if the temperature is lowered to −16�C (point B). However, if film is at a room-temperature moisture equilibrium much higher than 60% RH, this treatment could result in the Tg of the gelatin being exceeded during a warm-up period, particularly if the temperature is close to 40�C (table 1). When the gelatin is above Tg, the emulsion layer is more susceptible to physical damage such as ferrotyping or sticking.
Fig. 18.
Moisture equilibrium curve of 35 mm color motion picture film on cellulose triacetate base
 |
It would certainly be less labor-intensive and more user-friendly if photographic film could just be placed in a freezer without vapor-tight packaging. The argument for this approach is that many frost-free cold chambers maintain a moderate relative humidity, with only periodic excursions to a high humidity during a frost removal cycle when the temperature is increased. This cycle is illustrated in figure 19 for a Kenmore frostless freezer operating at approximately −16�C and is typical of many commercial products. While the frost-free feature prevents the relative humidity of the air from being at 100% RH for any appreciable time period, the average RH is approximately 50% RH at −16�C. This condition is at a borderline moisture level because of possible problems during the warm-up period as discussed above. Prolonged storage at a high water content is also potentially damaging as it could increase the possibility of mold growth or lessen the beneficial effects of cold storage. The obvious conclusion is that even with the use of frost-free freezers, packaging in moisture-proof enclosures is recommended (Adelstein et al. 1970; McCormick-Goodhart 1996). Closed but untaped containers may not provide adequate protection with many commercial chambers.
Fig. 19.
Humidity cycling of Kenmore frostless freezer as result of temperature cycling at −16�C (�2�C) and periodic defrost cycles
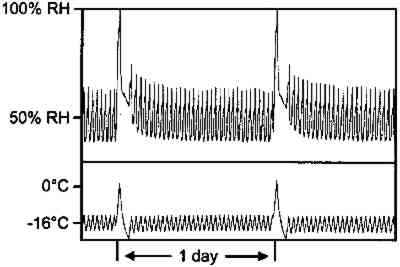 |
An additional benefit of enclosures is that they avoid the possibility of moisture condensation on the film during the warm-up period.
3.2.2 REFRIGERATORS
Refrigerators do not offer the same cost-benefit advantages as freezers. However, refrigerators above the freezing point (2 to 5�C) can be recommended because of the marked advantages they provide over room temperature storage.
Under normal operating conditions, high humidity should not be a concern. However, in commercial equipment with an attached freezer unit there is the possibility of high moisture levels in case of power failure and equipment malfunction. For this reason the photographic material should be enclosed in moisture-protective (but not necessarily moisture-proof) packaging such as cans or polyethylene bags. These will also prevent moisture condensation on the photographic material during the warm-up period. Cardboard boxes are not recommended.
3.2.3 COLD STORAGE VAULTS
The same factors that were considered for freezers also apply to cold storage vaults. However, the latter may be equipped with controls that ensure that the humidity does not increase to unacceptable levels. From the data presented in figure 18, it is recommended that the maximum relative humidity be set at 40% RH. During storage the enclosure needs only to provide physical protection. However, there must be some moisture protection during the warm-up period. An additional polyethylene bag should suffice.
4 CONCLUSIONS
- Experimental results on the effect of temperature on the moisture equilibrium curve of photographic film agree with published information.
- Recent studies of moisture equilibration rates at low temperatures provide more comprehensive data than had been available. These investigations indicate that while low-temperature equilibration is slow, it is still appreciable.
- The use of moisture-proof packing is recommended for storage in frost-free freezers or in cold storage vaults where the relative humidity exceeds 40%.
- Analysis of gelatin degradation studies at conditions both above and below the glass transition temperature show that linear Arrhenius relationships may be obtained, although situations have been reported where this is not true.
ACKNOWLEDGEMENTS
This work was done under a grant from the Office of Preservation, National Endowment for the Humanities.
REFERENCES
Adelstein, P. Z.1973. Physical properties of photographic materials. In Handbook of photographic science and engineering. New York: J. Wiley and Sons. Sec. 8 SPSE.
Adelstein, P. Z., C. L.Graham, and L. E.West. 1970. Preservation of motion-picture color films having permanent value. Journal of the Society of Motion Picture and Television Engineers79(November):1011–18.
Adelstein, P. Z., J. M.Reilly, D. W.Nishimura, and C. J.Erbland. 1992a. Stability of cellulose ester base photographic film. Part 1, Laboratory testing procedures. Journal of the Society of Motion Picture and Television Engineers101(May):336–46.
Adelstein, P. Z., J. M.Reilly, D. W.Nishimura, and C. J.Erbland. 1992b. Stability of cellulose ester base photographic film. Part 2, Practical storage considerations. Journal of the Society of Motion Picture and Television Engineers101(May):336.
Anderson, S., and D.Kopperl. 1993. Limitations of accelerated image stability testing. Journal of Imaging Science and Technology37(July/August): 363–73.
ANSI. 1964. American National Standard method for determining the melting point of a nonsupport layer of films, plates and papers, PH4.11-1964. New York: American National Standards Institute.
ANSI. 1992. American National Standard method for determining the resistance of photographic emulsions to wet abrasion, IT9.14-1992. New York: American National Standards Institute.
ANSI. 1993. American National Standard for imaging media, processed safety photographic films, storage, IT9.11-1993. New York: American National Standards Institute.
Calhoun, J. M.1944. The physical properties and dimensional behavior of motion picture film. Journal of the Society of Motion Picture Engineers43(October): 227.
Cargocaire Engineering Corp.1990. The dehumidification handbook. 2d ed.Amesbury, Mass.: Cargocaire Engineering Corp.
Carver, E. K., R. H.Talbot, and H. A.Loomis. 1943. Film distortions and their effect upon projection quality. Journal of the Society of Motion Picture Engineers41(July):88.
Eastman Kodak Co. 1972. Physical properties of Kodak aerial films. Report M-62. Rochester, N.Y.: Eastman Kodak Co.
Eastman Kodak Co. 1983. The book of film care. Report H-23. Rochester, N.Y.: Eastman Kodak Co.
Goodrich, A. B.1980. Audiovisual records at the John F. Kennedy Library: A profile. Picturescope28(3):6–7.
Heldman, D. R., C. W.Hall, and T. I.Hedrick. 1965. Equilibrium moisture contents and moisture adsorption rates of dry milks. In Humidity and moisture. Vol. 2, Applications, ed.A.Wexler. New York: Reinhold Publishing Corp.
Hughes, F. J., J. L.Vaala, and R. B.Koch. 1965. Rapid measurement of moisture in flour by hygrometry. In Humidity and moisture. Vol. 2, Applications, ed.A.Wexler. New York: Reinhold Publishing Corp.
Hukill, W.1965. Moisture in grain. In Humidity and moisture. Vol. 2, Applications, ed.A.Wexler. New York: Reinhold Publishing Corp.
Iwano, H.1994. Estimation of relative humidity in the sealed film can. Report presented to ANSI/NAPM Subcommittee IT9-3. October.
Kach, D.1978. Photographic dilemma: Stability and storage of color materials. Industrial Photography27(August):28–50.
McCormick-Goodhart, M.1994. Moisture content isolines. Report presented to ANSI/NAPM Subcommittee IT9-3. April.
McCormick-Goodhart, M.1995. Moisture-content isolines of gelatin and the implications for accelerated aging tests and long-term storage of photographic materials. Journal of Imaging Science and Technology39(March/April): 157.
McCormick-Goodhart, M.1996. The allowable temperature and relative humidity range for the safe use and storage of photographic materials. Journal of the Society of Archivists17(1):7–21.
Meyer, A., and D.Bermane. 1983. The stability and permanence of Cibachrome images. Journal of Applied Photographic Engineering9:121–25.
Newsome, P. T., and S. E.Sheppard. 1932. The sorption of water vapor by cellulose and its derivatives. Part 3, The heat of adsorption of water vapor by cellulose acetates. Journal of Physical Chemistry36:930.
Parker, J. T,. and L. J.Sugden. 1963. Determining the resistance of photographic emulsions to damage during processing. Photographic Science and Engineers7(January): 41–47.
Ram, A. T., D. F.Kopperl, R. C.Sehlin, S.Masaryk-Morris, J.Vincent, and P.Miller. 1994. The effects and prevention of the “vinegar syndrome.”Journal of Imaging Science and Technology38(3):249–61.
Reilly, J. M.1995. Accelerated aging tests. Paper presented at the Symposium on Research Techniques in Photographic Conservation. Copenhagen, Denmark.
Rose, P. I.1977. Gelatin. In The theory of the photographic process, ed.T. H.James. 4th ed.New York: Macmillan.
Ulm, R.W. K.1938. Influence of atmospheric humidity and temperature on the moisture content of paper board. Paper Trade Journal8:108.
Urquhart, A. R., and A. M.Williams. 1924. The moisture relations of cotton: The effect of temperature on the absorption of water by soda-boiled cotton. Journal of the Textile Institute15:T559.
Wilhelm, H.1978. Storing color materials. Industrial Photography27(October):32.
Wilhelm, H.1993. The permanence and care of color photographs. Grinnell, Ia.: Preservation Publishing Co.687–726.
Young, J. H., J. M.Bunn, and W. H.HensonJr. 1965. Humidity and moisture problems associated with the handling and storage of cured tobacco. In Humidity and moisture. Vol. 2. Applications. ed.A.Wexler. New York: Reinhold Publishing Corp.
AUTHOR INFORMATION
PETER Z. ADELSTEIN received a B. Eng. in chemistry from McGill University, Canada, in 1946 and a Ph. D. in chemistry from the same university in 1949. He was employed at the Eastman Kodak Co. from 1949 to 1986, retiring as unit director of a product development laboratory. Since that time he has been a senior research consultant at the Image Permanence Institute. He chairs national and international committees on the permanence of imaging materials. Address: Image Permanence Institute, Rochester Institute of Technology, Rochester, N.Y. 14623.
JEAN-LOUIS BIGOURDAN obtained a B.S. in chemistry in 1973, a diploma in chemistry from the Institut Universitaire de Technologie, Poitiers, France, in 1979, and a diploma in museum studies in 1986 from the Ecole Nationale de la Photographie, Arles, France. He received an M.S. in art conservation from the Institut Fran�ais de Restauration des Oeuvres d'Art, Paris, in 1993. Since 1994 he has been studying the effect of enclosures on film storage at the Image Permanence Institute. Address as for Adelstein.
JAMES M. REILLY graduated with a B.A. from Franklin and Marshall College in 1968 and an M.A. from the State University of New York at Buffalo in 1972. He has continued his education in science at the Rochester Institute of Technology. In 1984 he was appointed director of the Photo Preservation Laboratory at the Rochester Institute of Technology, and the following year he organized and was made director of the Image Permanence Institute at the same institution. He is also a full professor in the School of Photographic Arts and Science. Address as for Adelstein.
Section Index |