THE ROLE OF CLAYS IN THE DECAY OF ANCIENT EGYPTIAN LIMESTONE SCULPTURES
CARLOS RODRIGUEZ-NAVARRO, ERIC HANSEN, EDUARDO SEBASTIAN, & WILLIAM S. GINELL
ABSTRACT—One type of Egyptian limestone from Naga el-Deir (Abydos/Thebes region) exhibits an ongoing problem of deterioration typified in the form of continued delamination of the surface in a stela from Naga el-Deir acquired from archaeological investigations carried out in the early 20th century. Previous testing of this limestone type indicated the presence of sodium chloride and sodium nitrate. The sculptures have been treated and desalinated either by immersion in water or by aqueous poulticing, but the decay process was not halted, and major loss of surface stone was still noticeable after storage for a period of years.Mineralogical and petrographic data (x-ray diffraction [XRD], scanning-electron microscopy [SEM], and optical microscopy) indicate that this stone has a high proportion of clays (up to 10% by weight). Laboratory tests suggest that the clays, concentrated along bedding planes, are largely responsible for the type of deterioration noted. The role of clay minerals in the decay of this type of limestone was demonstrated by performing a series of experiments, including wetting/drying cycles and relative humidity changes, thermomechanical analysis, and accelerated decay tests using water and ethylene glycol. One conclusion of this study is that in some instances, desalination procedures can induce more deterioration than can rigid environmental control. Another conclusion is that attribution of deterioration to the presence of salts may be insufficient, and further petrographic analysis should be initiated prior to desalination of clay-rich limestones. Unconventional methods for possible stabilization of the clay structure (by means of ion exchange and/or surfactant treatment) are also discussed.
TITRE—Le R�le des Argiles dans le D�composition des Anciennes Sculptures �gyptiennes en Calcaire. R�SUM�—Un type de calcaire �gyptien provenant de Naga el-Deir (r�gion d'Abydos/ Th�bes) est confront� � un probl�me permanent de d�t�rioration. On le voit bien en observant la d�squamation progressive de la surface d'une st�le de Naga el-Deir trouv�e pendant des fouilles arch�ologiques au d�but du XX�me si�cle. Des analyses ant�rieures effectu�es sur ce type de calcaire signalent la pr�sence de chlorure de sodium et de nitrate de sodium. Les sculptures avaient �t� trait�es et d�ssal�es soit par immersion dans l'eau, soit par un cataplasme aqueux, mais le processus de d�t�rioration ne fut pas arr�t� et l'on a pu constater que des pertes �taient toujours consid�rables apr�s de nombreuses ann�es en r�serve.Les donn�es min�ralogiques et p�trographiques (analyse en diffraction, microscope �lectronique � balayage) indiquent que cette pierre contient une haute proportion d'argiles (jusqu'a 10% en poids). Les essais en laboratoire sugg�rent que les argiles, concentr�es le long des couches de stratification, sont en grande partie responsables de ce genre de d�t�rioration. Le r�le des min�raux d'argile dans le d�composition de ce type de calcaire a �t� d�montr� par une s�rie d'exp�riences comprenant plusieurs cycles de mouillage/s�chage et des changements d'humidit� relative, une analyse thermom�canique et des tests de d�t�rioration acc�l�r�e en employant de l'eau et du glycol �thyl�ne. Une des conclusions de cette �tude est que les proc�dures de dessalement peuvent, dans certaines circonstances, provoquer plus de dommage que ne le ferait un contr�le strict des conditions climatiques; un deuxi�me point est que l'attribution de la d�t�rioration � la pr�sence de sels n'est pas suffisante et que davantage d'analyses p�trographiques devraient �tre effectu�es avant le dessalement des calcaires riches en argile. En outre, des m�thodes peu conventionnelles pour une possible stabilisation des min�raux d' argile (par les moyens d'un �change d'ions et/ou un traitement par surfactant) sont aussi examin�es.
TITULO—El papel de las arcillas en el deterioro de antiguas esculturas egipcias de piedra caliza. RESUMEN—Un tipo de piedra caliza egipcia de Nagael-Deir (regi�n de Abydos/Tebas) exhibe un problema de deterioro progresivo que se manifiesta en la delaminaci�n continua de la superficie en una estela de Nagael-Deir adquirida en unas investigaciones arqueol�gicas llevadas a cabo a principios del siglo XX. Pruebas previamente realizadas en este tipo de piedra caliza indicaron la presencia de cloruro s�dico y nitrato s�dico. Las esculturas fueron tratadas y desalinizadas ya sea mediante inmersi�n en agua o con emplasto acuoso, sin embargo el proceso de deterioro no pudo ser detenido y la perdida apreciable de material superficial era aun notable despu�s de un per�odo de almacenamiento de varios a�os.Los datos mineral�gicos y petrogr�ficos (DRX, SEM y microscop�a �ptica) indican que esta piedra tiene una elevada proporci�n de arcillas (hasta un 10% de su peso). Los ensayos de laboratorio sugieren que las arcillas, concentradas a lo largo de los planos estratigr�ficos, son en gran parte responsables por el tipo de deterioro observado. El papel de los minerales de la arcilla en el deterioro de este tipo de piedra caliza fue demostrado realizando una serie de experimentos, que incluyeron ciclos de humedad/sequedad y cambios de humedad relativa, an�lisis termomec�nico, y pruebas de envejecimiento acelerado usando agua y etil�n glicol. Una de las conclusiones de este estudio es que en algunos casos los procedimientos de desalinizaci�n tienen el potencial de inducir mayor deterioro que un r�gido control ambiental. Otra de las conclusi�n es que la atribuci�n del deterioro a la presencia de sales podr�a ser insuficiente y se deber�an iniciar an�lisis petrogr�ficos adicionales antes de desalinizar las piedras calizas ricas en arcilla. Son discutidos ademas algunos m�todos no convencionales para la posible estabilizaci�n de la estructura de las arcillas (por medio de intercambio ionico y/o tratamiento con tensoactivos).
1 INTRODUCTION
Damage created by swelling clays commonly found as minor components in ornamental and building stone is a major problem in the conservation of cultural heritage (Kuhnel et al. 1994; Ruiz de Argando�a et al. 1995; Brattli and Broch 1995). It has been reported that structural damage to building and sculptural materials can occur even when the clay fraction in a particular stone is quite small (Dunn and Hudec 1966; Delgado-Rodrigues 1976; McGreevy and Smith 1984; Wendler et al. 1991; Snethlage et al. 1995). Water penetrating within the pore system of the stone can produce swelling of the clays, and the resulting swelling pressures can damage the internal stone structure. Repeated cycles of wetting and drying can lead to almost complete destruction of the stone.
Much of the limestone commonly used for building and sculptural purposes usually contains a small proportion of clay (Caner and Seeley 1978). However, the clay fraction in micritic and biomicritic limestones can reach values of more than 10% (Folk 1962). It has been reported that limestone used as building stone or for sculptures is prone to rapid decay when in contact with water if the stone contains more than 5% clay (Rodriguez-Navarro 1994). This rapid decay is associated with clay that is concentrated along bedding planes, because, as reported by Dunn and Hudec (1966), some limestones containing more than 30% clay homogeneously distributed within the stone are very sound and stable.
In the work reported here, the composition and textural arrangement of the clay minerals in samples of Egyptian limestone were studied. The samples were obtained from a micritic limestone sculpture that had sustained major structural damage while stored in a museum environment. Typically, the damage consisted of splitting and spalling, which resulted in loss of the surface details. The samples were taken from a stela that was excavated from a Second- to Fourth-Dynasty (2720–2150 B.C.) cemetery in Naga el-Deir in the early 20th century (Phoebe Hearst Museum of Anthropology, Berkeley, California).
In previous studies carried out on Thebes-Abydos limestone sculptures, it was concluded that most of the observed damage was due to the presence of salts (sodium chloride and sodium nitrate) (Oddy et al. 1976; Helms 1977; Charola et al. 1983; Hanna 1984; Bradley and Middleton 1988; Middleton and Bradley 1989; Miller 1992; Nunberg et al. 1996). In fact, many sculptures were routinely immersed in water shortly after excavation to extract salts (Winlock 1921; Oddy et al. 1976). However, in most cases the decay process was not stopped by this treatment (or other salt-extractive treatments such as poulticing), as evidenced by major loss of surface stone after storage for a period of years (Miller 1992). Barton and Blackshaw (1976) and Bradley and Middleton (1988) pointed out that the mineralogy, especially the clay content, should play an important role in making this stone susceptible to decay, but further studies on the contribution of clays to the decay of these ancient Egyptian sculptures were not performed. It should be mentioned, however, that salt damage can be enhanced in the presence of clays (Harvey et al. 1978; Fookes and Poole 1981). McGreevy and Smith (1984) proposed a model in which decay problems related to clays could be enhanced by the presence of soluble salts.
It has been observed that these Egyptian sculptures, as well as many others fabricated from Thebes-Abydos limestone and stored in different museums, developed the same pattern and degree of decay, even though they were not exposed to an outdoor environment (Charola et al. 1983; Bradley and Middleton 1988; Miller 1992). In fact, in a museum environment these limestone sculptures experienced a varied loss of surface relief while being subjected only to relative humidity and temperature changes in the storage areas. This damage, in many cases, is not explained solely by the action of salts.
To demonstrate the role of clay minerals in the decay of the Naga el-Deir Egyptian limestone stela, experiments were performed that included a thermomechanical analysis of clay swelling behavior in the presence of distilled water, cyclic wetting/drying (using distilled water and ethylene glycol), and relative humidity (RH) cycling decay tests.
2 MATERIALS AND METHODS
Samples were taken from the completely defaced Egyptian stela, which now has no remaining surface details. Mineralogical, compositional, and petrographic analyses were performed using optical microscopy, x-ray diffraction (XRD), inductively coupled plasma mass spectrometry (ICPMS), ion chromatography (IC), and scanning-electron microscopy (SEM).
The clay fraction in the stone samples was extracted using the following experimental protocol. Fifty grams of crushed limestone were treated with 0.2 N HCl, and the acid insoluble residue was washed repeatedly with distilled water. The clay fraction was then separated by centrifugation. Oriented clay aggregates (air-dried, ethylene glycol [EG]–solvated, and heated to 550�C) were prepared. XRD analysis of the oriented clay aggregates was performed with a Philips PW-1710 diffractometer equipped with graphite monochromator and automatic slit using CuKα radiation. Elemental silicon was used as the internal standard. The clay content was calculated from the weight of the clay fraction following drying at 90�C for 24 hours.
To evaluate the swelling behavior of the clays within the stone, the following experiments were carried out:
- Samples (2 � 2 � 10 cm) of Naga el-Deir limestone were partially immersed in a beaker filled with distilled water. The water surface was then covered with paraffin wax to allow capillary migration of the water only through the stone pore system. Because this stone was obviously laminated, the samples were oriented with the bedding planes perpendicular to the bottom. Macroscopic damage was recorded photographically, and efflorescence, when present, was analyzed afterward.
- To identify the damage due to swelling of the clay and to distinguish it from damage due to salt growth (if any), stone pieces (2 � 2 � 5 cm) of Naga el-Deir limestone were placed in a vacuum desiccator, impregnated with ethylene glycol (well known as a material that swells clays), and placed in an oven at 60�C for 12 hours. Once the pieces were removed from the desiccator, they were dried at 100�C for 12 hours. This process was repeated three times. It was observed that no heat-related damage took place when control limestone samples were heated to 100�C (3 cycles). Damage created by expansion of the clay structure due to ethylene glycol solvation was recorded photographically. Changes in porosity and pore size distribution were measured by mercury intrusion porosimetry (MIP). Stone samples were also submitted to the experimental protocol described above using distilled water to swell the clays (wetting/drying cycles).
- Damage created by expansion of clays due to relative humidity changes was evaluated by placing limestone samples (5 � 3 � 2 cm) in a climate-controlled chamber (Micro Climate Technology, model 92MCG-TC) at constant temperature (20�C) and cycling RH (40–90%). Each cycle took 8 hours. Eighty-four cycles were performed. At the end of the experiment, the total weight lost (scales that fell off) was determined. Total weight loss was expressed as a percentage of the original weight of the samples.
- Total expansion of the stone due to water absorption was estimated using thermomechanical analysis (TMA). Limestone samples (5 � 5 � 5 mm) were placed in the TMA sample holder (Mettler TMA, model TA300), with the bedding planes parallel to the bottom, at constant temperature (30�C) and relative humidity (50%). When the system was stabilized (typically after 30 minutes from the start of the experiment), distilled water was added, and the isothermal linear expansion of the samples was recorded (scan rate: 6 measurements/minute) for both the initial wetting and the final drying. When maximum expansion was reached, subsequent drying was achieved, and contraction of the sample was recorded. Two wetting/drying cycles were performed for each sample.
- High-magnification study of clay morphology and distribution within the limestones was performed using a scanning electron microscope.
3 RESULTS
The Egyptian limestones studied belong to the upper member of the early Eocene Thebes formation(Said 1962, 1990; Harrell 1992) and were quarried at Naga el-Deir (Abydos/Thebes region, Egypt) (fig. 1). Optical microscopy revealed these stones to be micritic limestones containing mostly micritic calcite with a small amount of dispersed bioclasts (mostly foraminifera). A few dolomite rhombohedra were also seen dispersed within the matrix. Bedding planes appear clearly marked by parallel fractures and bands of dark color, probably due to the high concentration of clays and iron oxides deposited along these planes (fig. 2).
Fig. 1.
Map of Egypt showing the locations of the Nile Valley limestone quarries and the provenance city of the studied stela
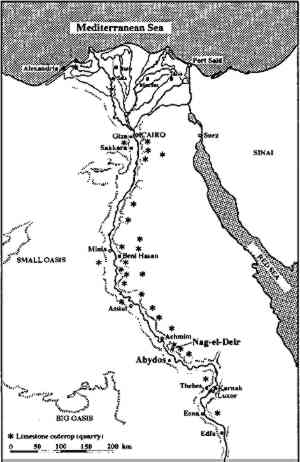 |
Fig. 2.
Optical micrograph of the Egyptian micritic limestone: (a) with bedding planes evident due to crack development where the clay is concentrated; D = dolomite (b) with dolomite rhombohedra visible through the limestone matrix Cl = clay; Fe = iron oxides
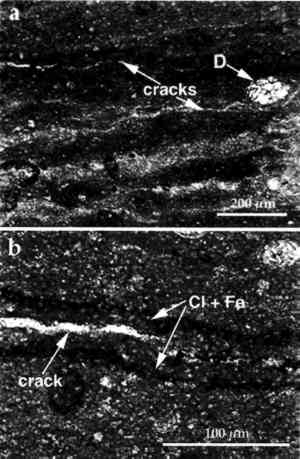 |
Chemical analyses were carried out to determine the salt content of the limestone. Table 1 shows the result of the ICPMS analysis for major cations as well as the result of the IC analysis for the major anions. MgO and SiO2 are present at levels consistent with magnesium-rich, clay-bearing limestone. These data also reflect the presence of dolomite together with the clays. The soluble salt content of the limestone is <1% and consists mainly of chlorides and nitrates.
TABLE 1. EGYPTIAN LIMESTONE SCULPTURE (NAGA EL-DEIR STELA) CHEMICAL COMPOSITION (WEIGHT PERCENT)
Powder XRD analyses of the bulk samples (fig. 3a) showed that calcite was the major phase (70%), with dolomite (<20%), clay minerals (<10%), and quartz (<5%) as minor phases. Mineral contents were calculated from XRD data. Due to the error of this technique (�5%), concentration of minor phases (clay and quartz) was also evaluated by weighing the acid-insoluble residue, which for the Naga el-Deir limestone was 15% by weight. Separation of the clay minerals (particle size <20 μm, the silt and clay fraction) from the quartz (particle size >20 μm, the sand fraction) was performed by centrifugation. The clay plus silt fraction was 10% of the original sample weight and the sand fraction 5%.
Fig. 3.
XRD difractograms (Cu Kα radiation; λ=1.5405 �) of: (a) powder XRD pattern of the bulk sample; (b) air-dried, EG-solvated, and heated to 550�C, oriented aggregates
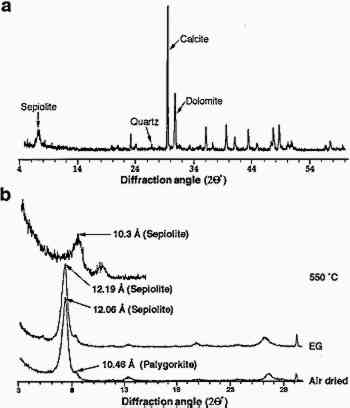 |
The fraction with particle size <2 μm was separated by centrifugation, as mentioned earlier, and an XRD analysis of the oriented clay aggregates was performed. Fig. 3b shows the diffractograms of oriented clay aggregates. An intense peak appears at 12.06 �, which changes to 12.19 � after treatment with ethylene glycol. This peak disappears after heating to 550�C and is transformed into a broad peak at 10.30 �. It was assigned to the (110) reflection peak of sepiolite. A minor peak at 10.46 �, corresponding to the palygorskite (110) reflection, was also detected.
Partial immersion in distilled water resulted in the complete disintegration of the immersed part of the stone. Above the water surface, salt efflorescence growth was detected. Whiskerlike halite (NaCl) crystals were deposited above the capillary fringe, but no damage was observed in this area. A minor amount of soda niter (NaNO3) also was detected. XRD analysis of the efflorescence confirmed the presence of halite (major salt) and soda niter (minor proportions). From IC chemical analysis data, the total salt content was found to be ∼ 1% by weight. Nevertheless, no damage was detected where salt growth took place.
After three ethylene glycol cycles, all samples suffered complete disintegration by fracture parallel to the bedding planes. The same type of damage was also achieved by wetting/drying cycles using distilled water. After immersion in distilled water, samples were thoroughly fractured along bedding planes. In many cases no second run was possible since total destruction of the samples was achieved in only one cycle. Extensive damage was also apparent after completion of a four-week relative humidity cycling test in an environmental chamber. Spalling and delamination of superficial scales (with thickness up to 4 mm) took place following RH cycling. An average weight loss of up to 7.17% was achieved after 84 cycles. No efflorescence growth was detected while performing this test.
Sample porosities were found to be 23.1%, 25.4%, and 27.3% for the control, the sample after EG, and the sample after water immersion (wetting/drying) cycles, respectively. It was concluded that macroporosity changes were due to damage created by cyclic expansion and contraction of the clays, which affected the internal microstructure of the stone, as shown by the change in pore size distribution (fig. 4). These changes were also detected by SEM observation and were evidenced by the formation of large fractures parallel to the bedding planes (fig. 5).
Fig. 4.
Pore size distribution diagram of Egyptian limestone comparing a blank and samples after cyclic wetting/drying using distilled water and ethylene glycol. P=porosity. Data from mercury intrusion porosimetry (MIP)
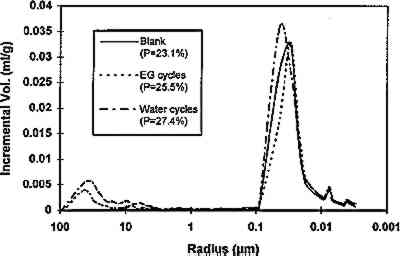 |
Fig. 5.
SEM micrographs of Egyptian limestone: (a) clays concentrated along bedding planes; (b) fracture developed parallel to the bedding planes after a wetting/drying cycle
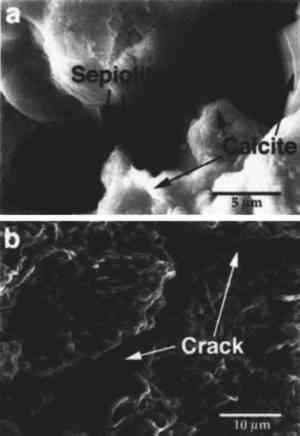 |
The results of the thermomechanical analysis are shown in figure 6. When immersed in distilled water (30�C) for less than 1 hour, the stone swelled about 3% in the direction perpendicular to the bedding planes. After the first wetting/drying cycle was completed, a 0.3% permanent mechanical deformation was noted. A second wetting/drying cycle produced a faster and greater expansion of the sample (up to 4%), which can be interpreted to mean that the structural damage created in the first wetting/ drying cycle enhances the damage produced in the following ones.
Fig. 6.
Thermomechanical analysis of Egyptian limestone: (a) first wetting/drying cycle; (b) second wetting/drying cycle
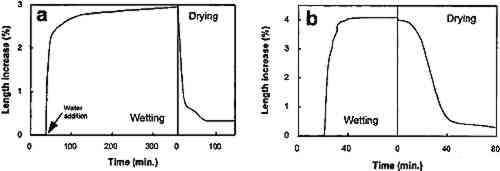 |
4 DISCUSSION
Mineralogical, petrographic, and chemical analyses confirmed the presence of large amounts of sepiolite and palygorskite concentrated along the bedding planes of the Egyptian limestone studied. The presence of salts (NaCl and NaNO3) was also confirmed. Immersion in distilled water, as well as wetting/drying cycles using distilled water and ethylene glycol, demonstrated that this limestone suffered severe damage when exposed to these liquids. No damage was observed where efflorescent growth took place. Relative humidity cycling also produced spalling and loosening of scales. From the results of the thermomechanical analysis, the damage appears to be due to the expansion of the stone perpendicular to the bedding planes where the clay minerals are concentrated. After some wetting/drying cycles, the expansion was shown to be partly irreversible. In fact, after immersion in water, the stone samples suffered the same damage that had been observed in the Naga el-Deir stela (delamination and massive loss of material), damage that was induced by relative humidity cycling in an environmentally controlled chamber. These experimental results lead us to consider that the presence of clays is a major contributor to the damage observed in the limestone. The damage mechanism involves the fixation of water molecules to the clay faces and results in eventual swelling.
Two kinds of clay swelling processes have been described (Marshall 1949; van Olphen 1977; Madsen and Muller-Vonmoos 1989): (1) crystalline swelling (short-range particle interaction, according to van Olphen [1977]), which is due to hydration of the exchangeable cations of the clay; and (2) osmotic swelling (long-range particle interaction, according to van Olphen [1977]), which is due to the large difference in the ion concentration close to the clay surface and the pore water. This last type of swelling mostly depends on ionic concentration, type of exchangeable ion (counter-ion), pH of the pore water, and type of clay.
Crystalline swelling of clays is a common phenomenon in expandable clays such as smectite and vermiculite (Norrish 1954). Nevertheless, although sepiolite and paligorskite are normally considered to be nonexpandable, they have been reported to undergo minor crystalline swelling in the presence of polar liquids such as ethylene glycol (Jones and Galan 1988). However, the extent of this swelling is small, and the swelling does not explain the large expansion observed in the limestones studied. Swelling processes have been described in nonexpandable clays, such as illite (McEwan and Wilson 1980) and kaolinite (Morris and Shepperd 1982) and are reported to be due solely to electrostatic forces (osmotic swelling). Thus, it can be hypothesized that, in this case, osmotic swelling of sepiolite and palygorskite is ultimately responsible for the expansion of and subsequent damage to the Naga el-Deir Egyptian limestone when in contact with water. The negatively charged surface of the clay can adsorb polar liquids (water) as well as various ions present. In this case, the presence of NaCl and NaNO3 within the stone offers a supply of sodium ions to the solution formed when water (either as vapor or as liquid phase) enters the pore system of the limestone. The sodium ions, in turn, become hydrated and produce an initial swelling of single fibrous clay crystals (crystalline swelling).
Then, osmotic swelling (interparticle swelling) due to electrostatic repulsion forces between nearby sepiolite and palygorskite particles, which are close-packed in very well-defined layers, as shown by SEM analyses, can create sufficient pressure to damage the layered structure of the stone. Repeated wetting/drying cycles can create irreversible deformation of the stone structure and, eventually, the loss of stone flakes and scales. In fact, this damage is the same type observed in the samples and in the stones that are used for sculptural purposes.
In this process, relative humidity cycling can lead to water condensation and swelling of the clays, promoting the damage observed in the microclimatic chamber as well as in the museum samples. It should be noted that the presence of salts within the pore system of the stone should promote condensation of water at relative humidity values lower than 100%. In the case of NaCl and NaNO3, deliquescence takes place when RH is above 75% (Arnold 1981), thus enhancing the problem due to clay expansion even when the RH changes are not very high.
Additionally, the presence of salt mixtures (nitrates and chlorides) tends to reduce the equilibrium relative humidity (RHeq) of the salts (Price and Brimblecombe 1994). Under these conditions, liquid water can be present within the pore system of the stone at relative humidity values below 75%. Hence, salts in this context are not directly responsible for the large damage suffered by this stone (no salt crystallization damage was detected); nevertheless, they contribute indirectly to the damage by reducing the RHeq (Arnold 1981; Price and Brimblecombe 1994) and by providing ions (sodium) that promote the osmotic swelling of the clays.
These results explain many observations indicating that damage of clay-rich stones is enhanced in the presence of salts (Fookes and Poole 1981; McGreevy and Smith 1984). In conclusion, all the data presented confirm that the swelling of the clays, enhanced by the presence of salts, is responsible for the observed damage in this type of Egyptian limestone.
To minimize these effects, Charola et al. (1983) and Bradley and Middleton (1988) reported that the Egyptian limestones were treated with surface coatings such as wax, but these treatments were found to be ineffective in most cases. One possible approach to stabilizing the stone could involve an unconventional method, such as reducing the swelling capacity of the clay by replacing sodium ions with calcium and/or magnesium ions (less hydrated) through cation exchange methods. Many studies indicate that clays containing exchangeable calcium ions do not swell as much as sodium clays (Norrish 1954; Chatterji et al. 1979; van Olphen 1987; Sridharan and Satyamurty 1996). Due to the high ionic exchange capacity of the clays, it should be possible to replace sodium ions (supplied by the salts) with divalent cations and reduce clay swelling (Marshall 1949; Norrish 1954; McEwan and Wilson 1980; van Olphen 1987). In fact, lime washes have been used extensively to stabilize expansive clay-rich soils (Grim 1962; Basma and Tuncer 1991), since calcium from the Ca(OH)2 can replace other highly hydrated cations (i.e., Na or K) in the clay structure (or adsorbed on the clay surface), thus reducing both the crystalline and the osmotic swelling capacity of the clays.
Another possible unconventional conservation method for reducing the swelling capacity of the clays could involve treatment with surfactants. Surfactants have been reported to influence both the rheological and the swelling properties of clays (van Olphen 1977; Permien and Lagaly 1995) because the adsorbed surfactant affects the ion distribution on the clay surface. Surfactants also have been reported to reduce the swelling capacity of clays by forming hydrophobic coatings on the clay surfaces (Theng 1974). The practical use of surface-active compounds as antiswelling agents for clay-rich ornamental stones has been reported by Snethlage et al. (1995) and Wendler et al. (1996). Using a surfactant, Wendler et al. (1996) were able to reduce the swelling capacity of the clay-rich tuff used in the carved statues (Moai) on Easter Island.
Nevertheless, much further work needs to be done in both areas before conservation treatment of ornamental stone is attempted. A much less invasive conservation measure would be to impose rigorous environmental controls (temperature and relative humidity) on the limestone storage areas to minimize cyclic changes and reduce clay swelling.
5 CONCLUSIONS
Limestone samples from a Naga el-Deir stela, which shows a high level of decay due to spalling and delamination in a museum environment, have been found to contain more than 10% by weight of clay minerals that are concentrated along the bedding planes. The mineralogy, morphology, and distribution of the clay were determined with optical microscopy, XRD, and SEM. These data confirm the presence of sepiolite as a major clay constituent, along with minor amounts of palygorskite. Cyclic wetting/drying laboratory tests using ethylene glycol and distilled water, relative humidity cycling, and thermomechanical analyses corroborate a working hypothesis that the swelling of sepiolite and palygorskite is the mechanism responsible for the observed fracture development and spallation damage. When water enters the pore system of the limestone, the clays expand primarily due to osmotic swelling and, to a minor extent, due to crystalline swelling promoted by the presence of sodium ions from halite and soda niter. Due to the presence of salts within the pore system of the limestone, water condensation takes place at RH values below 75%. In a museum environment, RH changes promote water condensation within the pore system of the limestone, resulting in eventual swelling of the clays. As demonstrated by the RH cycling within an environmental chamber, this condensation produces the cracking and the fracture development typically observed in decayed ancient Egyptian limestone sculptures.
To assure the future conservation of similar stone artifacts, a close environmental control of relative humidity and temperature within storage areas is recommended. Salt extraction using water, often a routine procedure, should be discouraged because this treatment promotes damage caused by clay swelling. Conventional treatments using hydrophobic coatings (wax, organic polymers, etc.) have not been found to be effective in most cases. It is proposed that clay stabilization studies involving replacement of existing sodium ions by calcium or magnesium, or by addition of surfactants, be initiated to control swelling. Nevertheless, prior to any conservation intervention, an accurate mineralogical, petrographical, and compositional study of the stone, as well as a complete laboratory testing to identify and evaluate the action of clays, should be developed.
ACKNOWLEDGEMENTS
This work was performed at the Getty Conservation Institute. Carlos Rodriguez-Navarro was supported by a postdoctoral fellowship from the Ministerio de Educaci�n y Ciencia Spain (no. PF94 24232705). Financial support was also provided by the Direccion General de Investigaci�n Cientifica y Tecuica (project no. PB 93/1090). We are indebted to George S. Wheeler for his critical review of the manuscript; Madeleine W. Fang of the Phoebe Hearst Museum of Anthropology, Berkeley, California, who supplied the Naga el-Deir stela samples; Eric Doehne for his help in performing the SEM analyses; and Michael Schilling, who performed the thermomechanical analysis.
REFERENCES
Arnold, A.1981. Nature of reactions of saline minerals in walls. In Conservation of Stone2, ed.M. R.Ross. Preprints of the international symposium. Bologna: Centro per la Conservacione delle Sculpture all Aperto. 13–23.
Barton, N. G., and S. M.Blackshaw. 1976. A statistical evaluation of the analyses carried out on Egyptian limestone. Lithoclastia2:11–16.
Basma, A. A., and E. R.Tuncer. 1991. Effect of lime on volume change and compressibility of expansive clays. Transportation Research Record1295:52–61.
Bradley, S. M., and A. P.Middleton. 1988. A study of the deterioration of Egyptian limestone sculpture. Journal of the American Institute for Conservation27:64–68.
Brattli, B., and E.Broch. 1995. Stability problems in water tunnels caused by expandable minerals: Swelling pressure measurements and mineralogical analysis. Engineering Geology39:151–69.
Caner, E. N., and A. P.Seeley. 1978. The clay minerals and the decay of limestone. In International Symposium on Deterioration and Protection of Stone Monuments. Paris: UNESCO/RILEM.
Charola, A. E., G. E.Wheeler, and R. J.Koestler. 1983. Treatment of the Abydos Reliefs: Preliminary investigations. In Proceedings of the 4th International Congress on the Deterioration and Preservation of Stone Objects, ed.K. L.Gauri and J. A.Gwinn. Louisville, Ky.: University of Louisville. 77–88.
Chatterji, S., P.Christensen, and G.Overgaard. 1979. Mechanisms of breakdown of natural stones caused by sodium salts. In Proceedings of the 3rd International Congress on the Deterioration and Preservation of Stone. Venice: Universita des li studi di Padoua.131–34.
Delgado-Rodrigues, J.1976. Estimation of the content of clay minerals and its significance in stone decay. In Deterioration of Building Stone, Second International Symposium. Athens: National Technical University. 105–8.
Dunn, J. R., and P. P.Hudec. 1966. Water, clay, and rock soundness. Ohio Journal of Science66:153–68.
Folk, R. L.1962. Spectral subdivision of limestone types. In Classification of carbonate rocks, ed.W. E.Than. Memoir of the American Association of Petroleum Geologists1:62–84.
Fookes, P. G., and A. B.Poole. 1981. Some preliminary consideration on the selection and durability of rocks and concrete materials for breakwaters and coastal protection works. Quarterly Journal of Engineering Geology14:97–128.
Grim, R. E.1962. Applied clay mineralogy. New York: McGraw-Hill.
Hanna, S. B.1984. The use of organo-silanes for the treatment of limestone in an advanced state of deterioration. In Adhesives and consolidants, ed.N.S.Brommelle et al. London: International Institute for the Conservation of Historic and Artistic Works. 171–76.
Harrell, J. A.1992. Ancient Egyptian limestone quarries: A petrological study. Archaeometry34(2):195–211.
Harvey, R. D., J.W.Baxter, G. S.Fraser, and C. B.Smith. 1978. Absorption and other properties of carbonate rock affecting soundness of aggregate. Geological Society of America: Engineering Geology Case Histories11:7–16.
Helms, G. M.1977.Conservation of Egyptian limestone: The Abydos reliefs. In Art Conservation Training Programs student papers third annual conference. Kingston, Ontario: Queen's University Art Conservation Program. 40–51.
Jones, B. F., and Galan, E.1988. Sepiolite and palygorskite. In Hydrous phyllosilicates, ed.S. W.Bailey. Michigan: Book Crafters, Inc.631–74.
Kuhnel, R.A., S. J.Van derGaast, J.Brych, G. J.Laan, and H.Kulnig. 1994. The role of clay minerals in durability of rocks: Observation on basaltic rocks. Applied Clay Science9:225–37.
Madsen, F. T., and M.Muller-Vonmoos. 1989. The swelling behavior of clays. Applied Clay Science4:143–56.
Marshall, C. E.1949.The colloid chemistry of the silicate minerals. New York: Academic Press.
McEwan, D.M.C., and J. J.Wilson. 1980. Interlayer and intercalation complexes of clay minerals. In Crystal structures of clay minerals and their x-ray identification, ed.G.W.Brindley and G.Brown. London: Mineralogical Society. 197–248.
McGreevy, J. P., and B. J.Smith. 1984. The possible role of clay minerals in salt weathering. Catena11: 169–75.
MiddletonA. P., and S. M.Bradley. 1989. Provenancing of Egyptian limestone sculpture. Journal of Archeological Science16:475–88.
Miller, E.1992. Current practice at the British Museum for the consolidation of decayed porous stones. Conservator16:78–83.
Morris, K. A., and C. M.Shepperd. 1982. The role of clay minerals in influencing porosity and permeability characteristics in the Bridgeport Sand of Wytch Farm, Dorset. Clay Minerals17:41–54.
Norrish, K.1954. The swelling of montmorillonite. Discussions of the Faraday Society18:120–35.
Nunberg, S., A.Heywood, and G.Wheeler. 1996. Relative humidity control as an alternative approach to preserving an Egyptian limestone relief. In Le dessalement des matriaux poreux. Poitiers: SFIIC. 127–35.
Oddy, W. A., M. W.Hughes, and S.Baker. 1976. The washing of limestone sculptures from Egypt and the Middle East. Lithoclastia2:5–10.
Permien, T., and G.Lagaly. 1995. The rheological and colloidal properties of bentonite dispersions in the presence of organic compounds. Part 5, Bentonite and sodium montmorillonite and surfactants. Clays and Clay Minerals43:229–36.
Price, C., and P.Brimblecombe. 1994. Preventing salt damage in porous materials. Preprints of the Congress on Preventive Conservation Practice Theory and Research, Ottawa. London: International Institute for Conservation. 90–93.
Rodriguez-Navarro, C.1994. Causas y mecanismos de alteraci�n de los materiales calc�reos de las Catedrales de Granada y Ja�n. Ph.D. thesis, University of Granada, Spain.
Ruiz de Argando�a, V. G., L.Calleja, and L. M.Suarez del Rio. 1995. Acoustic emission during swelling and contraction tests. Engineering Geology39:147–50.
Said, R.1962. The geology of Egypt. New York:Elsevier.
Said, R.1990. The geology of Egypt. Rotterdam: Balkema Publishers.
Snethlage, R., E.Wendler, and D. D.Klemm. 1995. Tenside im Gesteinsschutz-bisherige resultate mit einem neuen Konzept zur Erhaltung von Denkm�lern aus Naturstein. In Denkmalpflege und Naturwissenschaft, Natursteinkonservierung, ed.R.Snethlage. Berlin. 127–46.
Sridharan, A., and P.V.Satyamurty. 1996. Potential-distance relationships of clay-water systems considering the Stern Theory. Clays and Clay Minerals44:479–84.
Theng, B.1974. The chemistry of clay-organic reactions. New York: John Wiley and Sons.
vanOlphen, H.1977. An introduction to clay colloid chemistry. 2d ed.New York: John Wiley and Sons.
vanOlphen, H.1987. Dispersion and flocculation. In Chemistry of clays and clay minerals, ed.A.C.D.Newman. Mineralogical Society of London monograph 6. New York: John Wiley and Sons. 203–24.
Wendler, E, D. D.Klemm, and R.Snethlage. 1991. Contour scale on building facades: Dependence on stone type and environmental conditions. In Materials issues in art and archaeology, II, Materials Research Society Symposium Proceedings 185, ed.P. B.Vandiver et al. Pittsburgh: Materials Research Society. 265–71.
Wendler, E., A. E.Charola, and B.Fitzner. 1996. Easter Island tuff: Laboratory studies for its consolidation. In Proceedings of the Eighth International Congress on Deterioration and Conservation of Stone, ed.J.Riederer. Berlin: M�ller druck un Verlas Grumbh3:1159–70.
Winlock, H. E.1921. Bas-reliefs from the temple of Rameses I at Abydos. Metropolitan Museum of Art Papers1:54.
AUTHOR INFORMATION
CARLOS RODRIGUEZ-NAVARRO received his B.A. in geology and his M.S. in science and technology in the conservation and restoration of the cultural heritage from the University of Granada, Spain. In 1995, after receiving his Ph.D. in geology from the University of Granada, he accepted a postdoctoral research fellow position at the Getty Conservation Institute. He is currently working on research projects that deal with the mechanism of stone decay and conservation methodologies. The main topics of his present research are the role of salt and minor components (clays) in the alteration of building and sculptural materials and the testing and development of potential treatments for these problems. Address: Getty Conservation Institute, 1200 Getty Center Dr., Ste. 700, Los Angeles, Calif. 90049
ERIC F. HANSEN is a Fellow of AIC who received his M.S. in organic synthesis from the University of California, Irvine, and is a C. Phil. in the Archaeology Studies Program of the University of California, Los Angeles. He is currently an associate scientist at the Getty Conservation Institute, where he has been employed since 1985. His research interests include the consolidation of fragile painted objects, the deterioration of organic materials (including synthetic and natural substances), the effects of treatment parameters on the final physical properties of objects, the technological styles in the production of architectural sculpture of the ancient Maya, and the decay of ornamental porous calcareous materials. Address: as for Rodriguez-Navarro.
EDUARDO SEBASTIAN received his Ph.D. in geology from the University of Granada, Spain. He is currently an associate professor in the Department of Mineralogy and Petrology, University of Granada, and coordinator of the research group on stone conservation. His expertise includes clay mineralogy analysis, stone decay evaluation, nondestructive techniques for in situ stone decay diagnosis, conservation and analysis of lime mortar and plasters, and consolidation of natural stone, plasters, and mortars, as well as stabilization of earthen architecture. Address: Departamento de Mineraloga y Petrologa, Universidad de Granada, Fuente Nueva s/n, 18002 Granada, Spain.
WILLIAM S. GINELL received his Ph.D. in physical chemistry from the University of Wisconsin in 1949. Since then, he has held research positions at Brookhaven National Laboratory, Atomics International, Aerospace Corporation, and McDonnell Douglas Astronautics Company. He joined the Getty Conservation Institute in 1984 as head of materials science and is currently head of monuments and sites conservation research. His principal research interests are conservation in humid, tropical environments, stone and earthen materials conservation, seismic stabilization of historic structures, and architectural conservation. Address: as for Rodriguez-Navarro.
Section Index |