ULTRASONIC MISTING. PART 1, EXPERIMENTS ON APPEARANCE CHANGE AND IMPROVEMENT IN BONDING
STEFAN MICHALSKI, & CAROLE DIGNARD
4 DISCUSSION OF ALL RESULTS: APPEARANCE AND STRENGTH
4.1 THE SOLVENT SENSITIVITY PROBLEM
All the inorganic pigments darkened measurably by water alone, and all but white and chrome yellow lightened with ethanol. These color changes were not noticeable, or even perceptible, in many pigments tested, but 4 applications of water caused noticeable darkening in red ochre, noticeable darkening in raw umber, and unacceptable darkening in green earth. Green earth also had the highest initial cohesion (it did not need consolidation at all). The one pigment in this water-sensitive group tested for strength improvement, raw umber, actually weakened after application of ethanol solutions.
These findings suggest a strong coalescing effect on these pigments by wetting and drying with water, and a reversal by ethanol. We believe the effect is caused by intermeshing of points of contact between clay particles. Fortunately, green earth has recently been characterized in detail (Grissom 1986). It is of the clay mineral family. The primary mineral constituent, glauconite, has four groups, one of which is “an interlayered clay-glauconite pellet possessing swelling clay properties.” Furthermore, “because of the absorbency of this clay mineral, it has long been a substrate for dyes.” Red ochre and raw umber have not been recently characterized, but Gettens and Stout (1966) characterize both as “containing clays.” None of the other pigments tested contain clays.
We suggest that on drying of a water suspension of these clay pigments, some slight degree of intermeshing of the clay particles takes place. On rewetting the paint, but without flooding, the particles do not go back into suspension but remain bonded. They swell and push into each other further. Some, though not all, of this new coalescence is maintained after drying, hence there is more light absorption and a darker appearance. Each wetting-drying cycle can result in additional coalescence. (As in other paint shrinkage phenomena, volume loss is achieved primarily by thickness loss.) Ethanol can reverse this effect. The clay is initially at equilibrium with moderate relative humidity. It would then be dewatered by the ethanol. Then, as the ethanol evaporated, the clay would shrink enough to tear coalesced particles apart.
Green earth was so pronounced in its sensitivity to water that it was selected for examination by scanning electron microscopy (SEM). Electron micrographs were taken of a single area before and after water treatment, at various magnifications, and of cross sections of different areas before and after water treatment. No changes could be noticed in the SEM images (three observers, one with 15 years of experience with SEM). If the darkening is the result of changes in the interparticle geometry, or changes in the microstructure of each particle, such changes are too subtle to be seen by visual examination of SEM images.
4.2 COLOR CHANGE AND STRENGTH CHANGE WHEN DEPOSITION IS UNIFORM
Aside from solvent effects and skin effects, 4 and 10 mister applications of consolidant proved visually acceptable, sometimes even imperceptible, with the paints studied. For a porous, binderless paint saturated with solution of binder, one can show that after drying, BVC = (solution concentration)/(binder specific gravity)�porosity/(1-porosity), where concentration is expressed in the conventional weight/volume manner. Assuming paint layers (powders) of 30–50% porosity, assuming each application saturates the pores but does not flood the surface, and assuming a resin specific gravity of about unity, then each application of 0.5% solution, after drying, will have added binder of (0.2–0.5)% BVC. Since this added binder decreases the porosity negligibly, the next few applications will deposit the same amount. Therefore 4 applications deposit (0.8–2.0)% BVC, and 10 applications deposit (2–5)% BVC.
Figure 6 shows a diagram of the estimated bonding geometry for powders at 1 application and 4 applications. The width of the bonds in the figure was based on an initial idealized model of juxtaposed spheres, assuming an average of six bonds per sphere. Standard equations for the volume of a disc and a segment of a sphere were used to calculate the volume of the binder bonds. These equations, in turn, were transformed into a relation between BVC and bond-particle diameter ratio. Calculations of a pyramidal point and a flat surface approached similar values once the pyramid angle was shallow. Expressed as a decimal fraction of particle diameter, and given the range in porosity (30–50%) and resultant BVC estimates above, calculation yields bond diameters of 0.20 � 0.03 for 1 application, 0.30�0.03 for 4, and 0.38�0.05 for 10. This initial climb at 1 application (0.2) and leveling off between 4 (0.3) and 10 applications (0.38) is a simple result of bond geometry: the central region of the bond takes much less binder volume than the edges. This plateauing was borne out in the color change results (figs. 2, 3).
Fig. 6.
Concentration of consolidant, color change, and bonding; the approximate scale of bonding after treatments with 0.5% solution, followed by uniform drying (based on author's calculations with close-packed uniform spheres)
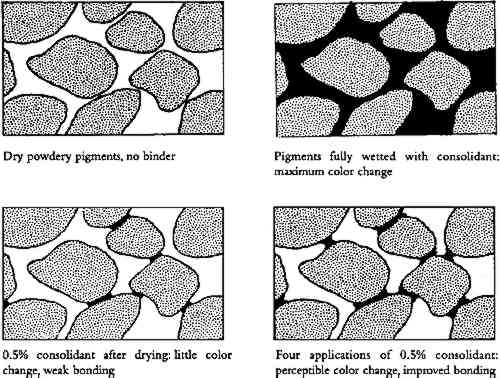 |
Tensile strength is known in gesso studies to be negligible until BVC reaches 7%. Then it climbs rapidly to almost its maximum by 15% BVC (Mecklenburg 1991), but Vicker hardness tests (Leitz 1972) on gessoes (Michalski 1990) did show significant strengths in mixtures with less than 7% BVC. The Vicker hardness value at 10% BVC was 12.5 (units kg/mm2), which is about the hardness of lead (Leitz 1972). Hardness dropped to 6 at 6% BVC, leveled out at 5.5 at 4% BVC, and was 3.2 at 2% BVC. At 1% BVC (the softest recipe tested), hardness fell to 1.5, half the value of double the binder, indicating simple proportionality in the range 0–2% BVC. Such hardness tests were not feasible on paints as thin as those of the present study. In the weak (ultramarine) or very weak (calcium carbonate) powdery paints tested, consolidation by 4 applications of consolidant (0.8–2% BVC) always gave noticeable or significant improvements in contact and abrasion resistance (aside from the glossy skinning AC-33). The gesso study also showed measurable hardness in this BVC range, about 1.2–3.2%. Below 2% BVC, the gesso study suggested one-quarter the hardness for one-quarter the consolidant and, indeed, compared to 4 applications, 1 application did result in a categorical drop in contact and abrasion resistance for most, though not all, of the initially weak paint samples.
Thus 4 applications (0.8–2% BVC) of nonskinning consolidant always improves paint cohesion and was expected to do so based on gesso results. These BVCs, however, are well below the 7% BVC needed to begin to develop tensile strength, i.e., resistance to flaking or crumbling. Such levels of BVC, unfortunately, would take all the colored pigments well beyond unacceptable changes in color.
Figure 6 also helps explain why white changes so little, compared to the colored pigments. White pigments reflect all wavelengths; scattering is their primary purpose. A small binder bond will not change scattering much, because the particle-air interface has not diminished much. Colored pigments depend on light absorption as well as scattering. A small bond will increase absorption significantly compared to no bond, because light absorption depends on the particle-binder interface.
4.3 UNEVEN DEPOSITION: THE SKIN PROBLEM
Aside from solvent sensitivity, the sole cause of unacceptable change in paint appearance was the formation of a skin (i.e., a top layer of paint that contained most, if not all, of the solute applied), despite solution travel all the way to the bottom (confirmed visually). These skins also led to easy delamination of the paint when tested for contact or abrasion resistance. This skinning implies a segregation of consolidant from its solvent. Segregation by a powder can occur by sieving: the movement of micelles or macromolecules are blocked by pores too small to allow passage. Segregation by a powder can occur by adsorption, also called the chromatography effect: macromolecules in solution are adsorbed by the surfaces of the particles rather than staying in solution. Finally, segregation in a powder can occur by reverse migration of the solution during drying of the wet paint: the solution wicks back to the surface to feed the evaporation front and brings solute with it. This latter mechanism mimics the production of efflorescence on a porous stone that has been saturated with a salt solution and then dried.
Particle and pore diameters play a role in at least two of these mechanisms. To a first approximation, pores in a powder can be modeled as those between close-packed spheres, which yield pore diameters of about 0.2 particle diameter. The pores in a powder with a mix of particle sizes are determined by the smaller particles, which fill the spaces between larger particles. Pigment particle sizes were not measured, but “medium” size has been categorized for pigments as 1 μm–3 μm, “fine” as 0.3 μm–1 μm, and “very fine” as below 0.3 μm (Feller and Bayard 1986). It is reasonable to suppose many of our pigments, given modern grinding, contained a significant fraction of at least fine particles. These particles would suggest pores of about 0.06 μm to 0.2 μm diameter. A powder whose smallest particles were “medium” would suggest pores of 0.2 μm to 0.6 μm.
With the emulsion AC-33, the effect is sieving. As Koob (1981) pointed out, an emulsion like AC-33 is not a sensible choice as a consolidant for materials with pores no bigger than the micelles, which act essentially as particles on the order of 0.1 μm diameter. These would be sieved immediately by fine particles and would even clog medium particles, since pores would only be a few micelles across. Sieving would create a clear, glossy film on top, and AC-33 was the only consolidant to produce a glossy skin.
Skins produced by the solutions (rather than the emulsion) were all matte. Since solutions of these consolidants form glossy films when evaporated in isolation, the matte surface suggests consolidant did deposit below the surface of the paint, even though it did not deposit at the bottom of the paint. This finding suggests that deposition was affected by adsorption or reverse migration. Reverse migration can be eliminated for the following reasons. A detailed physical model (Michalski unpublished) leads to the conclusion that reverse migration depends on the balance between solution flow toward the surface and solute diffusion back away from the surface. This rate balance reduces to solvent evaporation rate versus solute diffusion through the solvent. This balance cannot give rise to a reversal of behavior just because the type of powder changes, as occurred for gelatin and methylcellulose when the powder changed from carbon black (organic) to any of the other pigments (inorganic.)
Such dependence on solute and powder, particularly among two chemical classes of powder (i.e., organic versus inorganic), strongly suggests an adsorption mechanism. Adsorption is a specific interaction among consolidant molecule, solvent, and pigment particle surface. It can explain why methylcellulose and gelatin have reversed skin-forming tendencies in the organic pigment (ivory black) as compared to their tendencies in all the inorganic pigments.
Given a typical macromolecule adsorption layer of 6�10minus;7 kg/m2(Ash 1973; Vold and Vold 1983) and an idealized powder of close-packed spheres (porosity 25%), calculation (Michalski unpublished) shows that an application of 0.5% solution that wicks to the bottom of the powder, assuming adsorption of the resin from the solution, will see the adsorption band reach only 15% penetration from the top of the powder for 0.3 μm diameter particles, 35% for 1 μm particles, 65% for 3 μm particles, and 85% for 10 μm particles. Thus for the 0.5% solutions tested, 4 applications would push the adsorption band well down to the bottom of the powder in fine and medium particles but only halfway down in very fine particles. In other words, adsorption can be expected to cause segregation problems in consolidation of even thin layers, such as our samples, if a large percentage of the particles are very fine. Two other phenomena can interact with this adsorption problem, one to mitigate it, one to exacerbate it.
A phenomenon that mitigates the adsorption band problem (besides selection of a nonabsorbing solute/solvent combination) we call “adsorption lag.” Calculations (Michalski unpublished) based on equations for diffusion rate of macromolecules toward the pore walls from the middle of the pore channel (Vermeulen et al. 1984) combined with the equation for rate of capillary flow in a powder (Patton 1979) predict that solution will wick into a powder some distance before the macromolecules have time to move from the solution to the adsorption sites. For midweight macromolecules, this adsorption lag distance is about 100 μm in 3 μm particles, 20 μm in 1 μm particles, and 4 μm in 0.3 μm particles. These distances all triple for very high molecular weight (MW = 7�106). Thus the adsorption lag estimate suggests that while it would play a beneficial role in mitigating adsorption band problems in the paints we tested (30-70 μm thick) if the particles are medium in size and only one or two applications were tested, it does nothing to expand the adsorption bands expected in fine to very fine particles.
A phenomenon exacerbating adsorption is a problem we refer to as “choking,” whereby the adsorbed layer on the particles reduces the pore diameters significantly, so that sieving can occur. For example, while still in solution, linear polymers adsorb as a fuzz of kinked strands, in the range of 0.01–0.1 μm depending on molecular weight (Vold and Vold 1983; Hedgus and Kamel 1993). Additionally, macromolecules in solution can reach 0.5 μm diameter if the molecular weight reaches 7.5�106(Chaveteau 1986). Thus both the adsorbed layer and the swollen macromolecule become too big even for me-dium pigments with 0.2 μm pores if the molecular weight is very high.
None of the resins tested were in this very high molecular weight range. For example, the grade of methylcellulose used here (400 cP) was not as high as this, but perhaps it was still too high. This resin is available in several grades of lower viscosity, lower molecular weight, and these will be explored in other experiments. Methylcellulose has a further peculiar problem that would exacerbate its tendency to skin—the tendency for its macromolecules to form clusters in solution above 10�C. Combined with an initial adsorbed layer, this tendency would lead to formation of even thicker layers. In the oil industry, Chaveteau (1986) has explored such choking and clustering problems when dilute aqueous solutions of resin are used to displace oil in porous stone.
4.4 ELUTION AND GELLING: THE AVOIDANCE OF SKIN FORMATION
In a first approach to the adsorption problem, it is normal to think of the powder/solute interaction. In chromatography, however, the prevention of adsorption of solute on the powder column is known to depend also on the powder/solvent interaction. A strongly “eluting” solvent, one that lifts adsorbed solute back into solution, is a solvent that has stronger affinity for the powder than does the solute. Affinity has been modeled in chromatography literature as an empirical list of “strength” or by solubility parameters such as polarity, but models of acid/base interaction have been shown to best explain highly variable adsorption of polymethylmethacrylate on silica and calcium carbonate from different organic solvents (Fowkes and Mostafa 1978).
The simple conclusions from our data are that water will elute gelatin, but not methylcellulose, through the inorganic pigments green earth, red ochre, umber, ultramarine, chrome yellow, and calcium carbonate. On the other hand, water will elute methylcellulose, but not gelatin, through the organic pigment ivory black. Ethanol will elute B-72 through some carbon black and some inorganic pigments, but not through the clays green earth and red ochre.
One can now understand much of the traditional success of dilute glue or gelatin solutions in many consolidation treatments. Gelatin is successfully eluted by water through inorganic powders including whiting; it gels at low concentrations (unlike resins), thereby preventing any reverse migration; and it has excellent wetting abilities.
|