ULTRASONIC MISTING. PART 1, EXPERIMENTS ON APPEARANCE CHANGE AND IMPROVEMENT IN BONDING
STEFAN MICHALSKI, & CAROLE DIGNARD
ABSTRACT—Ultrasonic misting is a method of applying consolidation solutions to powdery surfaces that was developed at the Canadian Conservation Institute in 1989. The method was tested with four different consolidants, with water and ethanol carriers, and on binderless powdery paints of seven different pigments. Measurements were made of change in appearance after 1, 4, and 10 applications. Changes in cohesion and adhesion of the paint were measured on three of the pigments. Pigment, solvent, and consolidant all played a significant role in the results. Unacceptable color change can occur after wetting and drying by water alone in clay-type pigments. Gelatin in water gave the widest range of success: acceptable results in all but carbon black.
TITRE—N�bulisation Ultrasonique. 1�re Partie, Exp�riences sur le Changement d'Aspect et l'Am�lioration de l'Adh�sion. R�SUM�—La n�bulisation ultrasonique est une technique qui a �t� mise au point par l'Institut Canadien de Conservation en 1989 et qui est employ�e pour appliquer des consolidants liquides sur des surfaces pulv�rulentes. La technique a �t� test�e avec quatre consolidants diff�rents, en solution dans de l'eau ou de l'�thanol, sur des peintures pulv�rulentes sans liant, compos�es de sept pigments diff�rents. On a mesur� le changement d'aspect apr�s une, quatre, et dix applications. Les changements d'adh�sion et de coh�sion de la peinture on �t� mesur�s sur trois des pigments. Pigment, dissolvant et consolidant ont tous jou� un r�le dans les r�sultats. Un changement de couleur inacceptable peut se produire apr�s s�chage si l'on applique simplement de l'eau par n�bulisation sur les pigments � base d'argile. Une solution aqueuse de g�latine a fourni les meilleurs r�sultats qui sont acceptables pour tous les pigments sauf le noir de charbon.
TITULO—Nebulizaci�n por ultrasonido. Primera parte. Experimentos sobre cambios de aspecto y mejoramiento de la uni�n. RESUMEN—La nebulizaci�n por ultrasonido es un m�todo para aplicar soluciones consolidantes a superficies pulverulentas, desarrollado en el Instituto Canadiense de Conservaci�n en 1989. El m�todo fue probado con cuatro consolidantes diferentes, disueltos en agua y etanol, y sobre pinturas pulverulentas de siete pigmentos diferentes. Se midieron los cambios en el aspecto despu�s de una, cuatro y 10 aplicaciones. En tres de los pigmentos se midieron cambios en la cohesi�n y adhesi�n de la pintura. Tanto el pigmento como el solvente y el consolidante tienen un papel importante en los resultados. En pigmentos de tipo arcilloso puede ocurrir un cambio de color inaceptable luego de humedecer con agua �nicamente y secar. El rango mas amplio de resultados favorables se obtuvo con gelatina en agua, con resultados aceptables en todos los casos excepto para negro de humo.
1 INTRODUCTION
The ultrasonic mister for consolidation of powdery surfaces was first demonstrated by Stefan Michalski at the Getty Conservation Institute training course on Consolidation of Ethnographic Painted Artifacts in 1989. The assembly of the device and its method of use have been described elsewhere (Weidner 1993; Maheux and McWilliams 1995; Dignard et al. 1997; Michalski et al. forthcoming). Since development of the ultrasonic method, Arnold (1996) has extensively researched both pneumatic and ultrasonic nebulizers used for respiratory medications and identified several ready-to-use devices.
The main problem with consolidation of powdery paints is color change. Feller and Kunz (1981) tested a variety of binders mixed with ultramarine pigment and showed that the critical variable was not the type of binder but simply its amount. This amount—conventionally expressed by “pigment volume concentration” (PVC)—is the total microscopic volume of pigment particles expressed as a percent of the total microscopic volume of particles plus binder. Since this study is concerned with small amounts of binder, we have coined the parallel term “binder volume concentration” (BVC), so that a paint with very little binder can be referred to, for example, as 1% BVC, instead of 99% PVC. Feller and Kunz showed that any increase in binder in ultramarine caused measurable reduction in reflectance at red wavelengths (i.e., saturation of the blue color), but the relation between perceptible color differences and low BVC was not studied. Some pigments are definitely known to tolerate high BVC with no noticeable change in color. For example, in earlier work by one of the authors on the mechanical properties of gessoes (Michalski 1991), it was noted (as every worker in gesso will have realized) that there is no noticeable color difference between pure (0% BVC) whiting (CaCO3) and workable gesso recipes from soft (2% BVC) to hard (10% BVC). It is only with a jump to clearcole recipes (used as a base coat to ensure a good bond between the gesso and the wood) that a very noticeable darkening occurs. Clearcole recipes are about 40% BVC (Michalski 1991), so at some BVCs greater than 10% but less than 40%, gelatin binder causes a noticeable color change when added to a white pigment like whiting. Thus a preliminary answer to the question of how much binder can be added to a powdery paint before there is a perceptible change in color is that the amount appears to vary significantly with pigment, and whiting appears very tolerant.
Within the various treatment options for powdery paints reviewed by Hansen et al. (1993, xlvii), ultrasonic misting aims at improving penetration of the resin through the use of multiple applications of dilute solutions and the avoidance of excess consolidant delivery. Only nonviscous solutions can mist. For example, our apparatus could mist gelatin in water at no more than 1%, or methylcellulose of 400 cps at 0.5% in water, so dilute solutions are intrinsic to the technique. A dilute solution has low viscosity so it flows quickly and continues to flow even as some solvent is lost to evaporation. Although each application results in only small increases in bonding, it also results in little to no visual change. Thus one can consolidate in subtle increments and stop before appearance changes unacceptably.
Some consolidation techniques deliver too much consolidant too fast, leading to flooding of the surface and subsequent formation of a film or dark layer. However, the mister's small droplets, slow air stream, and narrow jet provide excellent control of delivery rate. Slow delivery also matches slow capillary penetration and thus avoids flooding of the surface.
Pneumatic sprayers can displace particles by impact of the large droplets or by the high-velocity air stream. In comparison, the ultrasonic mister's air stream velocity is low and adjustable to zero without affecting droplet production. (Trying to lower air velocity of pneumatic sprayers by reducing the air supply simply results in malfunction of the sprayer.) Mist droplets are very small: both ultrasonic devices (Shoh 1983) and the pneumatic nebulizer (Hudson 1995) produce their droplets in the range of 1–10 μm diameter. As illustrated in figure 1, mister droplets, unlike the enormous sprayer droplets (Fair 1984), are close in size to pigment particles. They are also similar in size to the fibers and pores in paper and much smaller than pigment clusters in pastel and chalk, hence their success in treatments by paper conservators (Weidner 1993).
With pneumatic sprayers, droplets are mixed with air completely free of solvent vapor so they evaporate rapidly as they travel toward the artifact. Thus the solution reaching the artifact is much more viscous than the prepared solution. In comparison, the ultrasonic mister delivers its droplets along with an air stream saturated with solvent vapor. The region directly under the nozzle is at saturated vapor conditions.
Proper misting technique involves holding the nozzle approximately 1 cm from the artifact and keeping it stationary until as much mist has wicked in as is required to just saturate the porous layer. Then the nozzle is moved just fast enough to maintain this saturating behavior.
Ultrasonic misting has the following disadvantages. Viscous solutions cannot be misted. Not all solvents work well: solutions in water, ethanol, or mineral spirits do, but some solutions in fast-evaporating solvents such as acetone do not. Drops of solution may form within the delivery tube and fall on the artifact unless precautions are taken. Although the central component, the ultrasonic humidifier, is commercially available, essential peripheral components must be handmade and often fine-tuned to complete the device (Michalski et al. forthcoming). Finally, consolidation treatment is slow.
This paper presents the results of consolidation tests using the ultrasonic mister on various powdery paints, determining changes in appearance, the formation of skins, and changes in cohesion or adhesion. A companion paper (part 2) describes the apparatus and a variety of treatment case histories.
2 APPEARANCE CHANGE EXPERIMENTS
2.1 APPEARANCE CHANGE: MATERIALS TESTED
The following modern pigments were tested: chrome yellow, ultramarine, ivory black, raw umber, natural red ochre, and green earth, all commercially ground pigments, and commercially ground calcium carbonate. Each pigment was mixed with water to form a slurry, then drawn down on glass plates. No binder was present. The resulting paints were opaque, with a thickness ranging from 30 μm to 120 μm depending on the pigment (table 1). Thickness was measured at the edge of the losses caused by the colorimeter stand (see the circles in fig. 4) using a microscope with a fine-focus knob calibrated in 1 μm increments.
Fig. 4.
Color change for (a) green earth (thickness 40–70 μm) and (b) raw umber (thickness 30–70 μm), drawn on glass, after 1,4, and 10 applications of water, 0.5% gelatin, 0.5% Rhoplex AC-33, and 0.5% methylcellulose. The lint and dust on the AC-33 treated area are stuck. The samples had been left for several months on an open shelf after the experiment ended, and loose dust was blown away before photography.
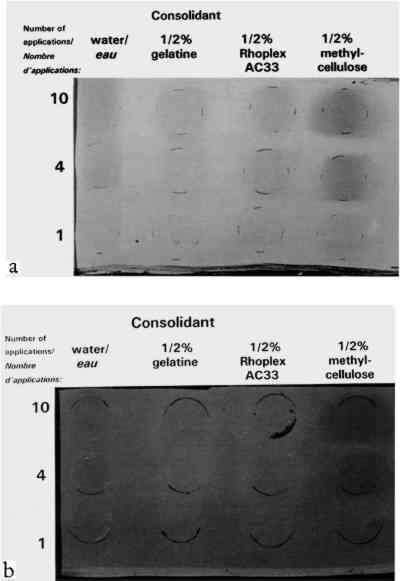 |
TABLE 1 THICKNESS OF PAINT FILMS ON GLASS SUBSTRATE
The dried paints were cohesive compared to their bulk pigment powder, and no pigment fell away when the plates were turned upside down. Most were, however, very vulnerable to contact or abrasion damage. Thus they represented the most extreme form of powdery paints encountered in museum artifacts, such as those devoid of binder or those in which surface binder has been disintegrated by UV exposure.
The solvents and solutions tested were water, ethanol (95%), gelatin in water at 0.5% w/v, Rhoplex AC-33 acrylic colloidal dispersion in water at 0.5% w/v, methylcellulose of low substitution (400 cP) in water at 0.5% w/v, and Acryloid B-72 acrylic resin in 95% ethanol at 0.5% w/v and at 0.125% w/v.
Powdery paint samples were misted with 1, 4, or 10 applications of the consolidant or solvent. Using the misting technique described in the previous section, consolidant mist was applied continuously to one area of paint until the solution reached the bottom of the paint layer and flooding of the surface began, then the nozzle was moved to an adjacent area of paint. The result was a slow scanning application of mist across the paint layer, the speed determined by the imminence of flooding. Treatments did not involve precise volumes of solution; the intent was simply to apply as much consolidant as would wick in, as in practical treatments. The penetration of the solution to the bottom of the paint layer was monitored using a mirror to view the bottom of the glass plate. Samples were allowed to air-dry between applications.
2.2 APPEARANCE CHANGE: METHODS OF MEASUREMENT
Color change was measured using a Minolta Chromameter model CR200 with its companion data processor unit. This instrument averages reflectance from a 1 cm diameter area. Before-treatment readings can be stored by the device, and in conjunction with after-treatment readings, a full range of conventional colorimetric parameters are tabulated. These are briefly outlined below.
Probably the most familiar color system to conservators is the Munsell Color System (Billmeyer and Saltzmann 1981, plates I–III). It is derived directly from visual experience and uses three coordinates. These are hue (red, green, etc.), value (lightness), and chroma (the variation from maximum color to a gray of the same lightness, as in the chroma adjustment on television sets). The preferred color system in the scientific literature, however, is L∗a∗b∗, derived from fundamental data on the color receptors in the human eye (Billmeyer and Saltzmann 1981, plate IV). The L∗ coordinate, “lightness,” is straightforward and corresponds to the Munsell coordinate “value.” The coordinate a∗ corresponds to redness-greenness, and coordinate b∗ to blueness-yellowness. Since the L∗a∗b∗ coordinates are unfamiliar to many, it can be helpful to transform them to coordinates analogous to Munsell's: hue, H∗; lightness, L∗; and chroma, C∗. Color change can be reported as the change in each of these coordinates, or as a total color difference, ΔE∗, calculated from the L∗a∗b∗ coordinates.
In this investigation, the change in lightness ΔL∗ and change in color ΔE∗ are reported. Changes in hue ΔH∗ and chroma ΔC∗ were monitored but are reported only in a few necessary instances. Lightness proved the most useful coordinate overall and corresponds to the darkening, or occasionally lightening, a conservator might expect from experience with consolidation. With optimum presentation of an optimum discrimination test, a just perceptible color difference is about 0.25–0.5 units in these scales, depending on the region of color space (Billmeyer and Saltzmann 1981, 106). The definition of “acceptable” color change has been endlessly debated in various industrial applications. In the gray scale for evaluating color change used by International Organization for Standardization (ISO) lightfastness tests, for example, the first full step in the scale, GS4, is ΔL∗ or ΔE∗ = 1.6, and the second full step, GS3, is ΔL∗ or ΔE∗ = 3.2. Purely for the sake of discussion, and recognizing that practical judgments will depend on color and circumstance, the step defined by GS4 for “noticeable” color change, ΔE∗ greater than 1.6, and GS3 for “unacceptable” color change, ΔE∗ greater than 3.2, has been adopted. “Imperceptible” color change has been reserved for ΔE∗ below 0.5.
The formation of a visibly distinct consolidant layer or “skin” on or near the top of the paint was determined by eye. The boundary between treated and untreated areas of paint was viewed from the top of the paint layer and compared to the same boundary viewed from the bottom of the paint layer (the glass sample plate was turned upside down). There was no ambiguity: skins were readily apparent as a large color change above and little to no color change below. Skins were further judged as matte or glossy by holding the glass plate and tilting it freely, looking for any specular reflection of ceiling fluorescent lighting from the paint surface. As with color change interpreted as skin formation, there was no ambiguity in the gloss/matte results. A glossy skin was deemed an unacceptable change in appearance, even if the measured color change alone did not warrant this categorization.
During visual inspection, we also looked for any change in surface texture between treated and untreated areas.
2.3 APPEARANCE CHANGE: RESULTS
The change in lightness, ΔL∗, is reported in figure 2, and the color difference, ΔE∗, in figure 3. The presence of a glossy skin is noted by a white square, a matte skin by a black square. No single treatment caused noticeable skinning.
Fig. 2.
Change in lightness, ΔL∗, of the paints as a function of the number of applications of consolidant or solvent. Legends at the top apply to all graphs below. The presence of a glossy skin is noted by a white square, a matte skin by a black square. The individual plots are labeled with the consolidant name only if they formed a skin.
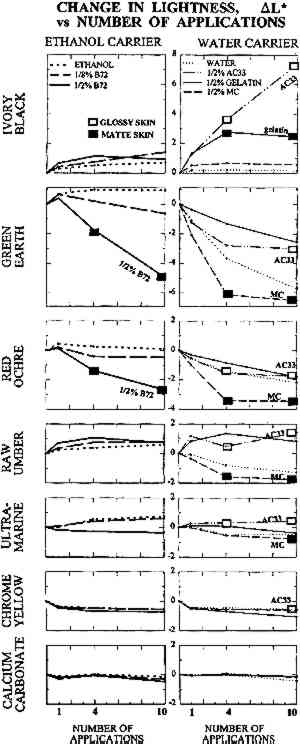 |
Fig. 3.
Color difference, ΔE∗, of the paints as a function of the number of applications of consolidant or solvent. Legends at the top apply to all graphs below. The presence of a glossy skin is noted by a white square, a matte skin by a black square. The individual plots are labeled with the consolidant name only if they formed a skin. The markers on the vertical scale indicate the color change chosen as “noticeable” (small double arrowhead) and “unacceptable” (large double arrowhead) for this study. (Just noticeable or perceptible is only about 0.25–0.5 units.)
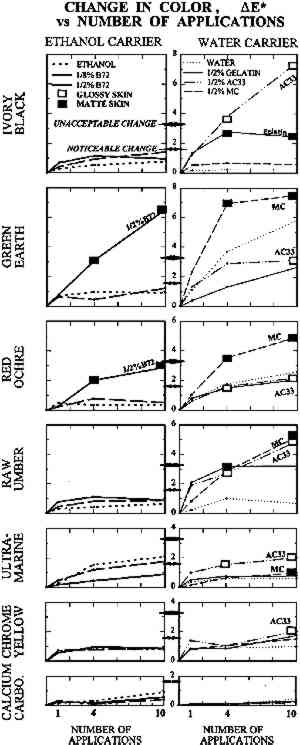 |
Despite important practical differences among consolidants discussed later, the most important experimental variable in color change was the pigment. Results will be discussed by pigment, from the most problematic to the least.
None of the pigments showed any noticeable change in texture, such as “orange peel” or raindrop effect, or a change from velvet to smooth, or a ridge of dislodged powder at the edge of the treated area.
2.3.1 Ivory Black
AC-33 and gelatin caused noticeable lightening with one application. The effect of 4 and 10 applications of gelatin was similar. Color change (lightening) remained just within the acceptable range, but the formation of a matte skin resulted in an unacceptable change in appearance. Four applications of AC-33, however, resulted in unacceptable lightening and a glossy skin. Ten applications doubled the color change. Methylcellulose did not cause noticeable change even at 10 applications. Ethanol and the B-72 solutions also did not reach noticeable change at 10 applications.
2.3.2 Green Earth
The green earth paint was the most vulnerable to color change across all consolidant systems, as is evident in figure 4a. After one application, the effects of all solvents and consolidants, except gelatin, were noticeable. Only gelatin remained acceptable at 10 applications; water alone darkened the paint twice as fast, reaching the unacceptable level by 4 applications. Methylcellulose and B-72 were both unacceptable after 4 applications and remarkably so by 10 applications. Repeated applications of ethanol alone caused lightening rather than darkening. One application of ethanol solutions also caused lightening, but subsequent applications caused darkening.
2.3.3 Red Ochre
As with green earth, red ochre was darkened by most treatments, except for initial slight lightening with one application of ethanol and its B-72 solutions. As with green earth, a dark skin formed with 0.5% B-72 at 4 and 10 applications, but color change remained just within the acceptable range at 10 applications. All aqueous solutions darkened the paint and caused almost noticeable gains in chroma. Only gelatin remained acceptable at 10 applications, causing less change than water alone.
2.3.4 Raw Umber
Some results from the misting of raw umber with water and the aqueous consolidants are shown in figure 4b. Water alone darkened umber noticeably after four applications. Of the aqueous consolidants, gelatin and AC-33 lightened this paint, while methylcellulose darkened it. In terms of color change, AC-33 and methylcellulose became unacceptable at 10 applications, but the formation of skins at 4 applications placed them in the unacceptable appearance category. Change in chroma and hue contributed significantly to the total color change. Unlike green earth and red ochre, umber responded well to ethanol and the B-72 solutions, the noticeable change occurring on 1 application and essentially no further change after 4 and 10 applications.
2.3.5 Ultramarine and Chrome Yellow
Of the colored pigments, the ultramarine and chrome yellow gave the smallest color changes. Like white, they were very bright to begin with. Most darkened (except ethanol and .125% B-72 in ultramarine), but the darkening alone would have been almost imperceptible. It was the accompanying shift in hue and chroma that made total color change noticeable by 10 applications of most consolidants. Glossy skin that developed made AC-33 unacceptable at 4 and 10 applications.
2.3.6 Calcium Carbonate
Although this white showed darkening when wetted by any of the solutions, as expected for a white pigment with poor scattering power in any binder, the darkening disappeared once the consolidant solutions dried, even at 10 applications. This finding demonstrates that whiting can be a very misleading pigment to use when testing a subtle consolidation process, because it is the least sensitive to color change.
3 STRENGTH IMPROVEMENT: COHESION AND ADHESION
3.1 STRENGTH IMPROVEMENT: MATERIALS TESTED
The initial strength of the seven pigments under study, when drawn as films with no binder, varied markedly. Green earth and natural red ochre were found to have very strong initial cohesion. They could be touched or lightly stroked by finger without any mechanical harm, and needed to be scratched by fingernail to be damaged. Raw umber also had good initial cohesion, although less than green earth or red ochre. Ivory black and ultramarine were powdery. They transferred pigment particles when touched but had some resistance to blowing by mouth. Calcium carbonate and chrome yellow were easily blown away by mouth.
Strength was tested on only three pigments, chosen to cover a range of initial cohesion from moderate to very weak: raw umber (moderate), ultramarine (weak), and calcium carbonate (very weak). There was little to be gained from testing pigments that formed paints stronger than one would ever consider consolidating. The pigments were tested on a wood (pine) substrate, in case a porous substrate affected the results. The 0.5% solutions of the four consolidants described in section 2 were misted as a band through the middle of the samples and were applied once on the proper right side and 4 times on the proper left side of the samples. The thickness of the films are given in table 2.
TABLE 2 RESULTS OF THE RESISTANCE OF CONTACT TRANSFERENCE AND RESISTANCE TO BRUSHING ABRASION TEST
3.2 STRENGTH IMPROVEMENT: METHOD OF MEASUREMENT
Two tests were developed: contact transference and brushing abrasion. In the contact test, blotting paper was laid on the surface (black paper for white paint), then a felt cushion, and the sandwich passed through a steel roller mill (used normally for flattening silver). The paper was then inspected for differences in transference, since the treated and untreated areas were side by side. This test would identify not only improvement in surface cohesion but also deficiencies in penetration, since the consolidated layer (skin) would lift away from unconsolidated powder. As noted, each paint had very different initial cohesion before consolidation, so the pressure required to provoke some change also had to be different: raw umber, which was inherently well bonded, required a setting of 3; ultramarine, 12; calcium carbonate, 17 (higher numbers are weaker settings of the spring squeezing the rollers).
The brushing abrasion test used an automatic draw-down device for paint, modified to draw a stiff, coarse-bristle brush across the paint samples at a constant speed and pressure. The number of passes and the weight were adjusted for each paint to discover differences between treatments, since the initial abrasion resistance of each pigment varied so much. The samples were then inspected for differences in the losses caused by the brush, since treated and untreated areas were side by side.
Thus both tests, contact and abrasion, were not absolute comparisons across the three pigments. Absolute comparisons would have required hundreds of tests and yielded trivial results, e.g., the appropriate test for umber would demolish calcium carbonate even at 4 and 10 treatments. These tests measured, for each paint, relative changes in cohesion and adhesion resulting from treatment, given very different initial strengths.
3.3 STRENGTH IMPROVEMENT: RESULTS
The samples are shown in figure 5: at the top are the blotting papers from the contact tests, where transfer of pigment can be observed in some instances; at the bottom are the paints after the brushing abrasion test. The visual assessments are compiled in table 2. Contact tests are given by C, abrasion by A. Two letters (CC or AA) indicate significant improvement to contact or abrasion; one letter means noticeable improvement; and a dash means no noticeable improvement.
Fig. 5.
Results of contact transference and brushing abrasion tests for (a) raw umber, (b) calcium carbonate, and (C) ultramarine. The blotting papers at the top are from the contact transference test. At the bottom are the samples on wood after the brushing abrasion test. The center of each sample was treated, once on the left, four times on the right.
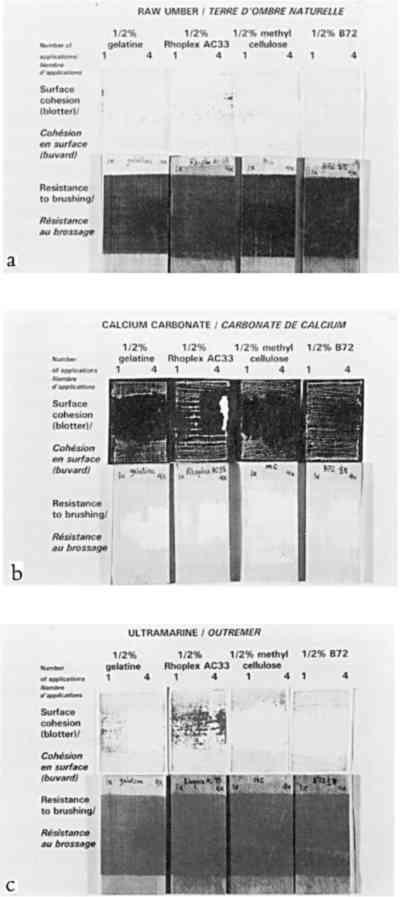 |
Exploratory tests used to set the severity of the brush and contact studies demonstrated that calcium carbonate, the weakest of the unconsolidated pigments chosen in this study, though stronger after 4 treatments with the best consolidant, was still more fragile than unconsolidated raw umber.
AC-33 was not a successful consolidant. On the blotter contact test, the skin stuck to the blotter and peeled off; with the brush abrasion test, the skin flaked off, leaving a powder residue on the wood. One application of 0.5% gelatin or B-72 gave too weak a bond for two of the three pigments. One application of 0.5% methylcellulose gave fair results, being on average as good as 4 applications of B-72. Within the range studied, all pigments improved the most with 4 applications of either 0.5% gelatin or 0.5% methylcellulose.
Some consolidants could weaken a pigment's initial strength: for example, it was found that 4 applications of B-72 on raw umber gave a weaker bond than the unconsolidated raw umber.
4 DISCUSSION OF ALL RESULTS: APPEARANCE AND STRENGTH
4.1 THE SOLVENT SENSITIVITY PROBLEM
All the inorganic pigments darkened measurably by water alone, and all but white and chrome yellow lightened with ethanol. These color changes were not noticeable, or even perceptible, in many pigments tested, but 4 applications of water caused noticeable darkening in red ochre, noticeable darkening in raw umber, and unacceptable darkening in green earth. Green earth also had the highest initial cohesion (it did not need consolidation at all). The one pigment in this water-sensitive group tested for strength improvement, raw umber, actually weakened after application of ethanol solutions.
These findings suggest a strong coalescing effect on these pigments by wetting and drying with water, and a reversal by ethanol. We believe the effect is caused by intermeshing of points of contact between clay particles. Fortunately, green earth has recently been characterized in detail (Grissom 1986). It is of the clay mineral family. The primary mineral constituent, glauconite, has four groups, one of which is “an interlayered clay-glauconite pellet possessing swelling clay properties.” Furthermore, “because of the absorbency of this clay mineral, it has long been a substrate for dyes.” Red ochre and raw umber have not been recently characterized, but Gettens and Stout (1966) characterize both as “containing clays.” None of the other pigments tested contain clays.
We suggest that on drying of a water suspension of these clay pigments, some slight degree of intermeshing of the clay particles takes place. On rewetting the paint, but without flooding, the particles do not go back into suspension but remain bonded. They swell and push into each other further. Some, though not all, of this new coalescence is maintained after drying, hence there is more light absorption and a darker appearance. Each wetting-drying cycle can result in additional coalescence. (As in other paint shrinkage phenomena, volume loss is achieved primarily by thickness loss.) Ethanol can reverse this effect. The clay is initially at equilibrium with moderate relative humidity. It would then be dewatered by the ethanol. Then, as the ethanol evaporated, the clay would shrink enough to tear coalesced particles apart.
Green earth was so pronounced in its sensitivity to water that it was selected for examination by scanning electron microscopy (SEM). Electron micrographs were taken of a single area before and after water treatment, at various magnifications, and of cross sections of different areas before and after water treatment. No changes could be noticed in the SEM images (three observers, one with 15 years of experience with SEM). If the darkening is the result of changes in the interparticle geometry, or changes in the microstructure of each particle, such changes are too subtle to be seen by visual examination of SEM images.
4.2 COLOR CHANGE AND STRENGTH CHANGE WHEN DEPOSITION IS UNIFORM
Aside from solvent effects and skin effects, 4 and 10 mister applications of consolidant proved visually acceptable, sometimes even imperceptible, with the paints studied. For a porous, binderless paint saturated with solution of binder, one can show that after drying, BVC = (solution concentration)/(binder specific gravity)�porosity/(1-porosity), where concentration is expressed in the conventional weight/volume manner. Assuming paint layers (powders) of 30–50% porosity, assuming each application saturates the pores but does not flood the surface, and assuming a resin specific gravity of about unity, then each application of 0.5% solution, after drying, will have added binder of (0.2–0.5)% BVC. Since this added binder decreases the porosity negligibly, the next few applications will deposit the same amount. Therefore 4 applications deposit (0.8–2.0)% BVC, and 10 applications deposit (2–5)% BVC.
Figure 6 shows a diagram of the estimated bonding geometry for powders at 1 application and 4 applications. The width of the bonds in the figure was based on an initial idealized model of juxtaposed spheres, assuming an average of six bonds per sphere. Standard equations for the volume of a disc and a segment of a sphere were used to calculate the volume of the binder bonds. These equations, in turn, were transformed into a relation between BVC and bond-particle diameter ratio. Calculations of a pyramidal point and a flat surface approached similar values once the pyramid angle was shallow. Expressed as a decimal fraction of particle diameter, and given the range in porosity (30–50%) and resultant BVC estimates above, calculation yields bond diameters of 0.20 � 0.03 for 1 application, 0.30�0.03 for 4, and 0.38�0.05 for 10. This initial climb at 1 application (0.2) and leveling off between 4 (0.3) and 10 applications (0.38) is a simple result of bond geometry: the central region of the bond takes much less binder volume than the edges. This plateauing was borne out in the color change results (figs. 2, 3).
Fig. 6.
Concentration of consolidant, color change, and bonding; the approximate scale of bonding after treatments with 0.5% solution, followed by uniform drying (based on author's calculations with close-packed uniform spheres)
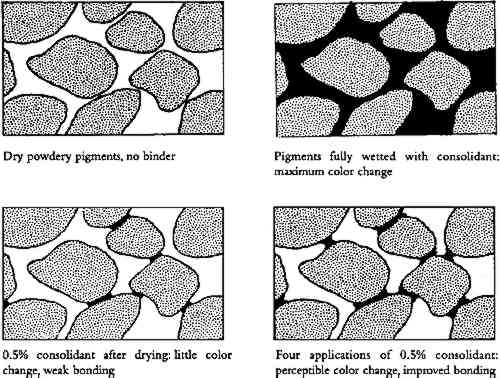 |
Tensile strength is known in gesso studies to be negligible until BVC reaches 7%. Then it climbs rapidly to almost its maximum by 15% BVC (Mecklenburg 1991), but Vicker hardness tests (Leitz 1972) on gessoes (Michalski 1990) did show significant strengths in mixtures with less than 7% BVC. The Vicker hardness value at 10% BVC was 12.5 (units kg/mm2), which is about the hardness of lead (Leitz 1972). Hardness dropped to 6 at 6% BVC, leveled out at 5.5 at 4% BVC, and was 3.2 at 2% BVC. At 1% BVC (the softest recipe tested), hardness fell to 1.5, half the value of double the binder, indicating simple proportionality in the range 0–2% BVC. Such hardness tests were not feasible on paints as thin as those of the present study. In the weak (ultramarine) or very weak (calcium carbonate) powdery paints tested, consolidation by 4 applications of consolidant (0.8–2% BVC) always gave noticeable or significant improvements in contact and abrasion resistance (aside from the glossy skinning AC-33). The gesso study also showed measurable hardness in this BVC range, about 1.2–3.2%. Below 2% BVC, the gesso study suggested one-quarter the hardness for one-quarter the consolidant and, indeed, compared to 4 applications, 1 application did result in a categorical drop in contact and abrasion resistance for most, though not all, of the initially weak paint samples.
Thus 4 applications (0.8–2% BVC) of nonskinning consolidant always improves paint cohesion and was expected to do so based on gesso results. These BVCs, however, are well below the 7% BVC needed to begin to develop tensile strength, i.e., resistance to flaking or crumbling. Such levels of BVC, unfortunately, would take all the colored pigments well beyond unacceptable changes in color.
Figure 6 also helps explain why white changes so little, compared to the colored pigments. White pigments reflect all wavelengths; scattering is their primary purpose. A small binder bond will not change scattering much, because the particle-air interface has not diminished much. Colored pigments depend on light absorption as well as scattering. A small bond will increase absorption significantly compared to no bond, because light absorption depends on the particle-binder interface.
4.3 UNEVEN DEPOSITION: THE SKIN PROBLEM
Aside from solvent sensitivity, the sole cause of unacceptable change in paint appearance was the formation of a skin (i.e., a top layer of paint that contained most, if not all, of the solute applied), despite solution travel all the way to the bottom (confirmed visually). These skins also led to easy delamination of the paint when tested for contact or abrasion resistance. This skinning implies a segregation of consolidant from its solvent. Segregation by a powder can occur by sieving: the movement of micelles or macromolecules are blocked by pores too small to allow passage. Segregation by a powder can occur by adsorption, also called the chromatography effect: macromolecules in solution are adsorbed by the surfaces of the particles rather than staying in solution. Finally, segregation in a powder can occur by reverse migration of the solution during drying of the wet paint: the solution wicks back to the surface to feed the evaporation front and brings solute with it. This latter mechanism mimics the production of efflorescence on a porous stone that has been saturated with a salt solution and then dried.
Particle and pore diameters play a role in at least two of these mechanisms. To a first approximation, pores in a powder can be modeled as those between close-packed spheres, which yield pore diameters of about 0.2 particle diameter. The pores in a powder with a mix of particle sizes are determined by the smaller particles, which fill the spaces between larger particles. Pigment particle sizes were not measured, but “medium” size has been categorized for pigments as 1 μm–3 μm, “fine” as 0.3 μm–1 μm, and “very fine” as below 0.3 μm (Feller and Bayard 1986). It is reasonable to suppose many of our pigments, given modern grinding, contained a significant fraction of at least fine particles. These particles would suggest pores of about 0.06 μm to 0.2 μm diameter. A powder whose smallest particles were “medium” would suggest pores of 0.2 μm to 0.6 μm.
With the emulsion AC-33, the effect is sieving. As Koob (1981) pointed out, an emulsion like AC-33 is not a sensible choice as a consolidant for materials with pores no bigger than the micelles, which act essentially as particles on the order of 0.1 μm diameter. These would be sieved immediately by fine particles and would even clog medium particles, since pores would only be a few micelles across. Sieving would create a clear, glossy film on top, and AC-33 was the only consolidant to produce a glossy skin.
Skins produced by the solutions (rather than the emulsion) were all matte. Since solutions of these consolidants form glossy films when evaporated in isolation, the matte surface suggests consolidant did deposit below the surface of the paint, even though it did not deposit at the bottom of the paint. This finding suggests that deposition was affected by adsorption or reverse migration. Reverse migration can be eliminated for the following reasons. A detailed physical model (Michalski unpublished) leads to the conclusion that reverse migration depends on the balance between solution flow toward the surface and solute diffusion back away from the surface. This rate balance reduces to solvent evaporation rate versus solute diffusion through the solvent. This balance cannot give rise to a reversal of behavior just because the type of powder changes, as occurred for gelatin and methylcellulose when the powder changed from carbon black (organic) to any of the other pigments (inorganic.)
Such dependence on solute and powder, particularly among two chemical classes of powder (i.e., organic versus inorganic), strongly suggests an adsorption mechanism. Adsorption is a specific interaction among consolidant molecule, solvent, and pigment particle surface. It can explain why methylcellulose and gelatin have reversed skin-forming tendencies in the organic pigment (ivory black) as compared to their tendencies in all the inorganic pigments.
Given a typical macromolecule adsorption layer of 6�10minus;7 kg/m2(Ash 1973; Vold and Vold 1983) and an idealized powder of close-packed spheres (porosity 25%), calculation (Michalski unpublished) shows that an application of 0.5% solution that wicks to the bottom of the powder, assuming adsorption of the resin from the solution, will see the adsorption band reach only 15% penetration from the top of the powder for 0.3 μm diameter particles, 35% for 1 μm particles, 65% for 3 μm particles, and 85% for 10 μm particles. Thus for the 0.5% solutions tested, 4 applications would push the adsorption band well down to the bottom of the powder in fine and medium particles but only halfway down in very fine particles. In other words, adsorption can be expected to cause segregation problems in consolidation of even thin layers, such as our samples, if a large percentage of the particles are very fine. Two other phenomena can interact with this adsorption problem, one to mitigate it, one to exacerbate it.
A phenomenon that mitigates the adsorption band problem (besides selection of a nonabsorbing solute/solvent combination) we call “adsorption lag.” Calculations (Michalski unpublished) based on equations for diffusion rate of macromolecules toward the pore walls from the middle of the pore channel (Vermeulen et al. 1984) combined with the equation for rate of capillary flow in a powder (Patton 1979) predict that solution will wick into a powder some distance before the macromolecules have time to move from the solution to the adsorption sites. For midweight macromolecules, this adsorption lag distance is about 100 μm in 3 μm particles, 20 μm in 1 μm particles, and 4 μm in 0.3 μm particles. These distances all triple for very high molecular weight (MW = 7�106). Thus the adsorption lag estimate suggests that while it would play a beneficial role in mitigating adsorption band problems in the paints we tested (30-70 μm thick) if the particles are medium in size and only one or two applications were tested, it does nothing to expand the adsorption bands expected in fine to very fine particles.
A phenomenon exacerbating adsorption is a problem we refer to as “choking,” whereby the adsorbed layer on the particles reduces the pore diameters significantly, so that sieving can occur. For example, while still in solution, linear polymers adsorb as a fuzz of kinked strands, in the range of 0.01–0.1 μm depending on molecular weight (Vold and Vold 1983; Hedgus and Kamel 1993). Additionally, macromolecules in solution can reach 0.5 μm diameter if the molecular weight reaches 7.5�106(Chaveteau 1986). Thus both the adsorbed layer and the swollen macromolecule become too big even for me-dium pigments with 0.2 μm pores if the molecular weight is very high.
None of the resins tested were in this very high molecular weight range. For example, the grade of methylcellulose used here (400 cP) was not as high as this, but perhaps it was still too high. This resin is available in several grades of lower viscosity, lower molecular weight, and these will be explored in other experiments. Methylcellulose has a further peculiar problem that would exacerbate its tendency to skin—the tendency for its macromolecules to form clusters in solution above 10�C. Combined with an initial adsorbed layer, this tendency would lead to formation of even thicker layers. In the oil industry, Chaveteau (1986) has explored such choking and clustering problems when dilute aqueous solutions of resin are used to displace oil in porous stone.
4.4 ELUTION AND GELLING: THE AVOIDANCE OF SKIN FORMATION
In a first approach to the adsorption problem, it is normal to think of the powder/solute interaction. In chromatography, however, the prevention of adsorption of solute on the powder column is known to depend also on the powder/solvent interaction. A strongly “eluting” solvent, one that lifts adsorbed solute back into solution, is a solvent that has stronger affinity for the powder than does the solute. Affinity has been modeled in chromatography literature as an empirical list of “strength” or by solubility parameters such as polarity, but models of acid/base interaction have been shown to best explain highly variable adsorption of polymethylmethacrylate on silica and calcium carbonate from different organic solvents (Fowkes and Mostafa 1978).
The simple conclusions from our data are that water will elute gelatin, but not methylcellulose, through the inorganic pigments green earth, red ochre, umber, ultramarine, chrome yellow, and calcium carbonate. On the other hand, water will elute methylcellulose, but not gelatin, through the organic pigment ivory black. Ethanol will elute B-72 through some carbon black and some inorganic pigments, but not through the clays green earth and red ochre.
One can now understand much of the traditional success of dilute glue or gelatin solutions in many consolidation treatments. Gelatin is successfully eluted by water through inorganic powders including whiting; it gels at low concentrations (unlike resins), thereby preventing any reverse migration; and it has excellent wetting abilities.
5 CONCLUSIONS
The first, and unexpected, result from this study was that some pigments achieve remarkable cohesion as paints when applied without any binder, especially the clay-containing pigments. These did not need consolidation, and, besides, they discolored the most when any consolidation treatment was used. Thus good mechanical strength in a matte paint cannot be taken as any indication of the presence of binder. If anything, it could be taken as a sign of color sensitivity to water. A painting technology based on natural earth colors could certainly produce artifacts of adequate strength without recourse to any binders at all.
For weak paints, however, ultrasonic misting can deliver small quantities of consolidant at low impact with no noticeable change to surface texture. It is definitely possible to improve paint strength without noticeable change in color (given a quite stringent criterion) and sometimes without even a just-perceptible change. Such levels of consolidation, 1–5% BVC, do not create robust paints, but they do improve the ability of weak paints to withstand vibration, handling, gentle contact, or gentle dusting. If the only powdery paint being treated is calcium carbonate (white) or, as is often the case, the greatest expanse of paint is calcium carbonate, and it is thick enough to maintain opacity, then levels of consolidation approaching 10% BVC would remain visually acceptable and produce a robust film. One would have to use caution so as not to apply such levels to adjacent colored areas, however, since they would cause unacceptable color change.
To avoid skinning, practitioners should try to vary not only the consolidant but also the solvent. The notion of elution applies not only to initial application of solution mist but also to application of solvent alone to push undesirable skins and tidemarks down into the paint or porous substrate. Some practitioners call this, appropriately enough, the application of a “chaser” solvent.
A particular form of treatment vulnerability that emerged from this study was the effect of water itself on claylike minerals, particularly green earth. These natural earth colors have a long history of use in many cultures, so they can be expected to occur frequently. As noted already, such clays have high initial strength and may not need consolidation. Whether this initial strength is lost with age (humidity fluctuations?) is not known. At the opposite extreme, calcium carbonate and ultramarine have no claylike characteristics and low initial strength. The fortunate coincidence is that the same lack of water sensitivity that makes them weak also makes their color tolerant of aqueous treatments.
If one had to select the best consolidant system overall from our limited study, it would be gelatin in water. Given the darkening role of each wetting and drying cycle on the claylike pigments, the fewer the number of gelatin treatment cycles, the better. Thus the greater mist concentrations possible with the nebulizer technology described by Arnold (1996) would have another advantage besides speed: it would reduce the number of wet-dry cycles and hence darkening. One must establish first that the higher viscosity would not inhibit penetration. In the consolidation of carbon black, however, results with gelatin were dismal; successful consolidants in carbon black were methylcellulose in water or B-72 in ethanol.
SOURCES OF SUPPLIES
CONSOLIDANTS:GelatinDr. Oetker Gelatin, 33617 Bielefeld, Germany Methocel A4CR methylcellulose, 400 cPsDow Chemical, Midland, Mich. 48640 Rhoplex AC-33R acrylic dispersion (ethyl acrylate/methyl methacrylate copolymer) and Acryloid B-72R acrylic resin (methyl acrylate/ethyl methacrylate copolymer)Rohm and Haas Canada, 2 Manse Rd. West Hill, Ontario M1E 3T9 Canada
PIGMENTS:Calcium carbonateAnachemia Chemicals, 255 Norman, Ville St-Pierre, Montreal, Qu�bec H8R 1A3 Canada Natural red ochre, green earth, raw umber, chrome yellow, ivory black, and ultramarineWilhelm D�ll, Erhardt-Strasse 10/2, Munich 5 Germany
REFERENCES
Arnold, D.1996. Recent advances in mist consolidation of powdery surfaces. AIC abstracts, American Institute for Conservation 24th Annual Meeting, Norfolk, Va. Washington, D.C.: AIC. 102.
Ash, S. G.1973. Polymer adsorption at the solid/liquid interface. In Colloid science, vol. 1, ed.D. H.Everett. London: Chemical Society. 103–20.
Billmeyer, F. W., and M.Saltzmann. 1981. Principles of color technology. 2d ed.New York: John Wiley.
Chaveteau, G.1986. Fundamental criteria in polymer flow through porous media and their importance in the performance differences of mobility-control buffers. In Water soluble polymers, ed.J. E.Glass. Advances in Chemistry series 213. Washington D.C.: American Chemical Society. 227–68.
Dignard, C., R.Douglas, S.Guild, A.Maheux, and W.McWilliams. 1997. Ultrasonic misting. Part 2, Treatment applications. Journal of the American Institute for Conservation36:127–41.
Fair, J. R.1984. Liquid-gas systems. In Perry's chemical engineer's handbook. 6th ed., ed.R.H.Perry and D. W.Green. New York: McGraw Hill. 18–52.
Feller, R. L., and M.Bayard. 1986. Terminology and procedures used in the systematic examination of pigment particles with the polarizing microscope. In Artists' pigments: A handbook of their history and characteristics, ed.R. L.Feller. Washington, D.C.: National Gallery of Art. 285–98.
Feller, R. L., and N.Kunz. 1981. The effect on pigment volume concentration on the lightness or darkness of porous paints. AIC preprints, American Institute for Conservation 9th Annual Meeting, Philadelphia. Washington D.C.: AIC. 66–74.
Fowkes, F. M., and M. A.Mostafa. 1978. Acid-base interactions in polymer adsorption. Industrial Engineering Chemistry Product Research Development17(1):3–7.
Gettens, R.J., and G. L.Stout. 1966. Painting materials. New York: Dover.
Grissom, C. A.1986. Green earth. In Artists' pigments: A handbook of their history and characteristics, ed.R. L.Feller. Washington, D.C.: National Gallery of Art. 141–68.
Hansen, E.F., S.Walston, and M. H.Bishop. 1993. Matte paint, its history and technology, analysis, properties and conservation treatments, with special emphasis on ethnographic objects. Bibliographic supplement to Art and archaeology technical abstracts 30. Marina del Rey, Calif.: Getty Conservation Institute and International Institute for Conservation.
Hedgus, C. R., and I. L.Kamel. 1993. Adsorption layer thickness of poly(methyl methacrylate) on titanium dioxide and silica. Journal of Coatings Technology65(821):49–61.
Hudson, R.C.I.1995. 1730 Nebulizer technical data sheet.
Koob, S.P.1981. Consolidation with acrylic colloidal dispersions. AIC preprints, American Institute for Conservation 9th Annual Meeting, Philadelphia. Washington, D.C.: AIC. 86–94.
Leitz. 1972. Instructions: Miniload hardness tester.
Maheux, A., and W.McWilliams. 1995. The use of the ultrasonic mister for the consolidation of a flaking gouache painting on paper. In Book and Paper Group annual14. Washington, D.C.: AIC. 19–25.
Mecklenburg, M.1991. Some mechanical and physical properties of gilded gesso. In Gilded wood, ed.D.Bigelow et al. Madison, Conn.: Sound View Press. 163–70.
Michalski, 1990. Vicker hardness of soft gesso. Canadian Conservation Institute, Ottawa, Ontario, Canada.
Michalski, S.1991. Crack mechanisms in gilding. In Gilded wood, ed.D.Bigelow et al. Madison, Conn.:Sound View Press. 171–84.
Michalski, S., C.Dignard, L.van Handel, and D.Arnold. Forthcoming. Ultrasonic mister: Application to consolidation treatments of powdery paint on wooden artifacts. In Painted wood: History and conservation. Proceedings of the symposium in Williamsburg, Va., September 11–14, 1994.
Michalski, S.Unpublished.Consolidation of granular material: A general physical model.
PattonT. C.1979. Paint flow and pigment dispersion. 2d ed.New York: John Wiley.
Shoh, A.1983. Ultrasonics (high power). In Kirk-Othmer encyclopedia of chemical technology. 3d ed.New York: John Wiley. 23:469.
Vermeulen, T., M. D.Levan, N. K.Hiester, and G.Klein. 1984. Adsorption and ion exchange. In Perry's chemical engineers' handbook. 6th ed.New York: McGraw Hill. 16/22.
Vold, R., and M.Vold. 1983. Colloid and interface chemistry. London: Addison-Wesley. 138–48.
Weidner, M. K.1993. Treatment of water-sensitive and friable media using suction and ultrasonic mist. Book and Paper Group annual 12. Washington D.C.: AIC. 75–84.
AUTHOR INFORMATION
STEFAN MICHALSKI graduated in 1972 with an honors B.Sc. in physics and math from Queen's University, Kingston, Ontario. From 1977 to 1979, he trained as an artifacts conservator in the master of art conservation program of Queen's University. Since 1979, he has been employed at the Canadian Conservation Institute, currently as senior conservation scientist. He is consultant to Canadian museums, libraries, and archives on preventive conservation and a researcher in physical aspects of deterioration and conservation processes. Address: Canadian Conservation Institute, 1030 Innes Road, Ottawa, Ontario, K1A OM5 Canada.
CAROLE DIGNARD graduated from the University of Ottawa with a B.Sc. in physics and Italian in 1981 and an honors B.A. in classical studies in 1983. She received a master's degree in art conservation from Queen's University in 1986, specializing in the conservation of objects. In 1987, she was a J. Paul Getty Fellow at the Peabody Museum of Archaeol-ogy and Anthropology, Harvard University. She has been working in the objects section of the Canadian Conservation Institute since 1988. Address: As for Michalski.
Section Index |