THE ROLE OF GELATIN IN PAPER PERMANENCE
TIMOTHY BARRETT, & CYNTHEA MOSIER
4 DISCUSSION
The primary findings of this study are summarized in table 1. The P-values (probability values) for all correlations reported in table 1 were less than .01. Statistically, this result is considered to mean there is less than 1 chance in 100 that the data distribution was a random or chance occurrence. The normal P-value threshold for considering a result “beyond chance” would be .05 or less. Results are also reported in table 2, which give an overview of all related data, and in figures 2, 3, and 4, where an association can be seen between higher L∗ value (lighter color) and higher pH, calcium content, and gelatin content.
TABLE 1 CORRELATION VALUES OF 35 HISTORICAL SPECIMENS
Fig. 2.
Scatter plot of L∗ dark-light values vs. surface pH readings (35 specimens)
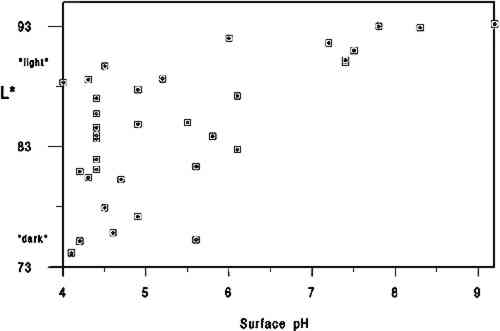 |
Fig. 3.
Scatter plot of L∗ dark-light values vs. PIXE ppm calcium determinations (35 specimens)
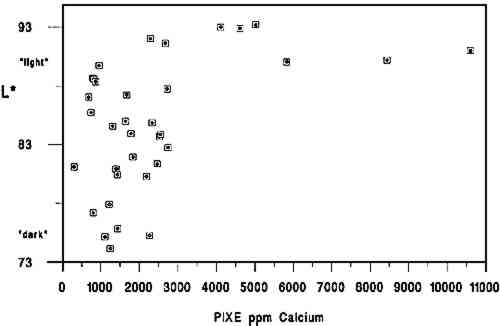 |
Fig. 4.
Scatter plot of L∗ dark-light values vs. weight percent gelatin values (35 specimens)
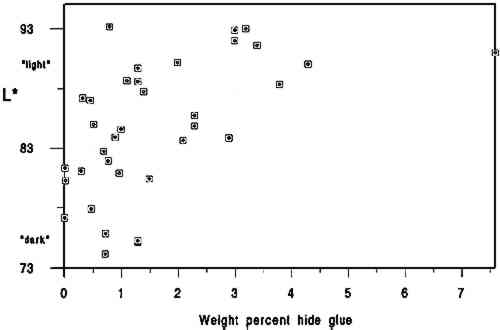 |
A brief introduction to interpretation of correlation values and their relative significance is warranted here for those readers unfamiliar with statistics. A perfect correlation between two variables would have a value of 1. The two variables would be considered to have a direct and systematic relationship with one another. A change in one variable correlates directly with changes in the other in a consistent manner. The correlation between the speed of a car and distance covered in one hour would obviously be 1, but the correlation between children's height and weight would be closer to .90. Data on two variables that are completely unrelated might have a correlation value of .1 or .2. In most carefully controlled scientific studies only values close to 1 would be considered to indicate a significant correlation. Lower values may be considered significant depending on the field of science, the material or variable being studied, and the nature of the sample pool. In a comparison of machine-made book papers subjected to accelerated aging in 1937 and later compared to 36 years of natural aging, Wilson and Parks (1980) considered correlation values from .75 to .98 fairly good to very good. However their sample pool consisted of machine-made papers, all made in 1937 at the same National Bureau of Standards experimental paper mill, while our 35 specimens were handmade, each in a different mill with different materials. In addition, they were all subjected to different storage conditions. We therefore feel that we can interpret the correlation values in table 2 as being more meaningful than might normally be the case.
We would summarize the results in table 1 by suggesting the correlation between L∗ and gelatin (.54) is strong enough to encourage further study of gelatin in paper permanence. The figures also suggest that no one variable is primarily responsible for the rise in L∗ value, but that they may all make a contribution. That is, the correlations between L∗ and pH (.64) and between L∗ and Ca (.48) also warrant more attention. Moreover, it appears that gelatin and pH, pH and calcium, and calcium and gelatin are all intercorrelated with one another with values of .45, .76, and .67 respectively. The three variables may turn out to be related in their contribution to paper permanence. One possible explanation is that calcium entered some papers when their gelatin sizes were made from parchment clippings, trimmings from limed skins, bones, and similar materials. Thus, gelatin might make a contribution to favorable pH in a manner separate from or in addition to the acid acceptor-buffering role suggested in the introduction.
In reviewing the figures and the data, we note that of the 10 lightest specimens, 8 are from the 10 highest in pH, 7 are from the 10 highest in calcium content, and 6 are from the 10 highest in gelatin content. Five of the 10 lightest specimens share top 10 values for pH, calcium, and gelatin, again indicating that the three variables may act together in promoting lighter paper color. Despite this clustering effect, the present data are not strong enough to support the notion that there are threshold values for each variable above which exceptional L∗ values or stability are evident.
Our data do not tell us the source of the gelatin, the methods used in its manufacture, or what impurities, such as fats, were present in the sizing solution. Nor do they tell us anything about the condition or length of the gelatin molecules, that is, whether excessive thermal or microbial degradation occurred either before or after the paper was sized. They simply give us a quantitative measurement of the amount of hydroxyproline present in the extracts of the papers, from which we have calculated gelatin content (estimated hide glue content).
An important question is whether the amounts of hydroxyproline measured in the extracts of the historical specimens are indicative of the amounts of gelatin originally applied. Could gelatin have been lost from the surface of the paper after sizing? We cannot know the storage conditions of the papers we tested, but they did not show signs of damage from water or microbes. Could deterioration of the gelatin molecule over the centuries have given inaccurate results? Deterioration might consist of breaking of peptide bonds. The strongest peptide bonds have been suggested to have a geologically short lifetime of 104 to 106 years (Hare 1969). Gelatin is hydrolyzed more readily and under less harsh conditions than many proteins (Leach and Eastoe 1977), such as wool or silk (Courts 1980). If this sort of deterioration has taken place, the chains of gelatin would be shorter but the amount of hydroxyproline present would still be the same.
Hydroxyproline itself is a moderately stable amino acid. It is not destroyed during 24-hour acid hydrolysis at 105�C, nor is it oxidized by heating at 105�C in the presence of oxygen for at least 8 hours. Free hydroxyproline has been found in the vicinity of fossilized collagenous materials 1 million years old (Ho 1965), although it is not known how long it had been liberated from the presumably protective collagen structure. All hydroxyproline had been lost from collagenous materials 150 million years old (Armstrong and Tarlo 1966). It seems unlikely to the investigators that deterioration of the hydroxyproline molecule under average storage conditions would be significant in the time span of our study, but there are no data to directly support this supposition.
It is known that gelatin becomes increasingly insoluble when heated at high temperatures or for prolonged periods (Leach and Eastoe 1977). In our own aging test (80 � 2�C, 0% RH for 2 months), we found the percent recovery of gelatin from the aged papers to be 6 � 2% lower than from unaged papers. Studies of gelatin films indicate that films conditioned at quite mild temperatures (45�C) and varying relative humidities become less soluble with increasing RH (Finch and Jobling 1977). These changes are attributed to either denaturation or cross-linking in the protein and may be related to the conditions under which the film was originally dried. If the solubility of the gelatin on the paper has decreased, its extraction from the paper will be incomplete and the results too low. We have shown that our recovery of gelatin was 96 � 5% in new paper reference standards dried at 105�C for 12 hours. Our results for the historic paper shown in table 2 are based on that figure. Unfortunately, we have no data to establish actual percent recovery from each historic specimen. Because it is unlikely that there are significant amounts of nitrogen from sources other than gelatin in these papers, a comparison of percent hydroxyproline recovered versus total nitrogen content via the Kjeldahl method (TAPPI 1991b) might be more useful in this regard than aging tests, whose results could not be applied with much certainty as a means of replicating the effects of long-term natural aging on gelatin in sized papers.
Assuming the hydroxyproline values reported here are indicative of the amounts of gelatin originally applied, our results suggest, as stated above, an association between lighter paper color and higher gelatin content. This finding would appear to contradict the tendency (mentioned in relevant literature above) of gelatin-sized papers to darken more than unsized counterparts during accelerated aging (Rasch 1929; Barrett 1989). The accelerated aging conditions used by these researchers may have caused reactions resulting in a large color change that would not occur during long-term natural aging. Since all of the darkest papers in the current study were characterized by their low gelatin content, this apparent discrepancy will remain until additional research can resolve it.
The key question at hand, from our perspective, is whether the chemical and physical systems of a historical leaf made with the characteristics we currently consider essential in a long-lasting paper (i.e., a neutral or alkaline pH, alkaline reserve, high alpha cellulose content, and low levels of iron and copper) could have been altered by the addition of high-quality gelatin in a manner that gave the paper significantly improved permanence. And if so, why? The answer to these and other questions related to the role of gelatin in paper permanence must be left for future work.
|