THE ROLE OF GELATIN IN PAPER PERMANENCE
TIMOTHY BARRETT, & CYNTHEA MOSIER
ABSTRACT—In William Barrow's classic study (1974) of historical paper specimens, he examined a number of factors that affect the stability of naturally aged papers, including cellulose fiber purity, alum content, amount of alkaline carbonate reserve, and pH. Barrow did not, however, investigate the effect of gelatin levels in his papers, and others have undertaken onlylimited research on the topic. In the study reported here, 40 historical paper specimens were analyzed to determine their gelatin content. Data from measurements of L∗ light-to-dark values, surface pH, and calcium content were compared with gelatin content. The data provided by these analyses show that both the appearance and the permanence of the papers may be affected by their gelatin content; avenues of further investigation in this area are outlined.
1 INTRODUCTION
Modern papermakers and conservation researchers have obtained useful data from the analysis of historical paper specimens. Most notably, William Barrow offered important new standards for permanent and durable machinemade papers based on his evidence that longer-lasting historical papers could be characterized by their alkaline pH, alkaline carbonate reserve, high-purity cellulose fiber, and freedom from highly acidic sizing materials (Barrow 1974).
Gelatin was commonly used as a sizing material between 1400 and 1800 to prevent the bleeding of ink. Added advantages were its ability to improve the strength, abrasion, and soiling resistance of the paper. Since gelatin was apparently common in paper of this period, we feel its role in paper permanence deserves research attention. It has been proposed that gelatin may act as an acid acceptor (Wilson and Parks 1983), meaning that component amino acids in gelatin act as a buffer (Courts 1980) and may make gelatin a significant buffer in paper (Erickson 1994; Swartz 1994). These proposed effects might give gelatin a role in protecting paper from acidic environmental pollutants and acidic degradation products. Other possible roles for gelatin include limiting oxygen or pollutant gas access to the cellulose or minimizing the negative effects of humidity cycling via a relative humidity buffering effect.
If gelatin is a factor in paper permanence, adjustments may need to be made in our current standards for permanent and durable papers. More important for conservators, we may need to pay closer attention to how much gelatin is being removed during conservation treatments and to consider whether or not it should be replaced.
Gelatin is a protein derived from the larger protein molecule collagen, which is a major constituent of hard and soft connective tissues in animals. When a tissue such as hide or bone is subjected to an alkali or acid pretreatment followed by extraction in heated water, chemical bonds in collagen are broken somewhat randomly, resulting in the shorter chains of amino acids we call gelatin. (We should point out that this is the present-day method of manufacture and that historically, gelatin may have been manufactured by extraction in heated water alone.) The length of these amino acid chains, which affects many of the properties of gelatin, can vary greatly within one batch, but in general a milder treatment will break fewer bonds and thus yield a larger number of longer molecules than will a more severe treatment.
Despite the variation in gelatin molecule lengths, the amino acid composition of all gelatins is distinguished by its relatively high content of 4-hydroxyproline, an amino acid found in large amounts in no other animal protein. Approximately one out of every 11 amino acids in gelatin is hydroxyproline. The percent hydroxyproline by weight in mammalian gelatins varies slightly depending on the source of the tissue, the method of manufacture, and the purity of the gelatin (Leach and Eastoe 1977); but as an example, a hide glue, the moderately pure gelatin similar to that likely used for paper sizing, contains about 12.6% hydroxyproline by weight (TAPPI 1991a).
There is an accurate method for determining the protein content of paper, but it is not specific for gelatin (TAPPI 1991b). If other proteins or nitrogenous compounds are not known to be absent, gelatin content is more accurately determined by measuring the hydroxyproline in the sample and using that value to calculate the amount of gelatin present. TAPPI Official Method T-504 (TAPPI 1991a), revised 1989, is a spectrophotometric procedure for the determination of glue or gelatin in paper by determining hydroxyproline. TAPPI states that this determination is not affected by the presence of glycerin, alum, or rosin. We used a modified microscale version of this procedure in our study, as described in section 3.
2 RELEVANT LITERATURE
In a study of factors influencing the stability (as determined by retention of folding endurance, tensile breaking strength, tearing strength, alpha cellulose content, and copper number after aging for 72 hours at 100�C) of experimental papers made from two different sulfite pulps, researchers reported that “all the [12] papers of the series were relatively low in stability with the exception of tub sizing run no. 225, a waterleaf sheet sized with glue containing no alum, which retained 71% of the initial folding endurance and decreased but 1 percent in alpha cellulose content after the accelerated aging test” (Rasch et al. 1933, 22).
In a study using identical aging conditions, results showed that seven gelatin sized papers retained an average 56% of their original fold endurance compared with four papers containing no gelatin size, which retained an average 16% (Rasch 1931). In a different study investigating wood fibers as a papermaking material, the same researcher noted that gelatin surface-size appeared to help “retard chemical degradation” in paper, as evidenced by superior retention of fold endurance and alpha cellulose, again after 72 hours of aging at 100�C (Rasch 1929, 500). In an investigation of highly purified wood fibers as papermaking material, workers noted in their conclusions that “surface sizing with glue or starch improved the resistance to the aging test [retention of folding endurance, bursting strength, tearing strength, tensile breaking strength, percent elongation at rupture, alpha cellulose content, and copper number after 72 hours at 100�C], especially with glue sizing” (Rasch et al. 1931, 782).
When humidity has been combined with elevated temperature in aging studies, again interesting but inconclusive results have been noted. In a Japanese study, when handmade papers were sized with traditional combinations of glue and alum and aged at 80�C and 80% RH, the size had no distinguishable effect on deterioration rate constants (Inaba and Sugisita 1988). The glue did, however, improve the mechanical properties of the paper. (It should be noted that the alum added during this study was 50% the weight of the dry gelatin—much more than would be recommended when and if alum is required during the production of handmade conservation papers). After moist heat aging at 80�C, papers sized with gelatin or gelatin and alum (alum added at 5% based on the weight of the dry gelatin) showed a higher rate of deterioration but superior mechanical properties when compared with unsized aged base sheets (Barrett 1989). After exposure to automobile exhaust followed by heat aging, gelatin-sized papers in another experiment showed a similar higher rate of strength loss, but again, better after-aging mechanical properties compared with aged controls free of gelatin (Barrett 1992).
Barrett (1989) noted an inclination for gelatin-sized papers to discolor more compared to their counterpart base paper without gelatin after moist accelerated aging at 80�C. Rasch (1929) made a similar observation after aging rag papers with and without gelatin sizing for 72 hours at 100�C but, as cited above, the same report also suggests gelatin surface size appeared to help “retard chemical degradation” in paper.
To the best of our knowledge, prior to the work we describe here, only one study of gelatin content in historical paper specimens has been mentioned in the literature (Barrow 1972). In it the author reports that 10 writing papers from the 17th and 18th centuries submitted to the National Bureau of Standards in about 1940 for testing contained between 2.6% and 5.8% glue. The method of determination is not stated. In our earlier qualitative analyses of historic papers, we observed higher levels of gelatin in papers considered to be in good condition compared with a second group considered to be in poor condition (Barrett 1992). In a continuation of that study, we have now made quantiative measurements of hydroxyproline in a different selection of naturally aged papers.
3 PROCEDURES
3.1 SELECTION OF SPECIMENS
More than 500 historical papers taken from printed books made between the 15th and the 18th century were available from an earlier study (Barrett 1989). Some of these sheets, particularly those from before 1500, may have originally been intended for use as writing papers. However, clear differences between printing and writing papers were not evident to us. From the 500-sheet pool we selected two sets of specimens: one of papers that seemed weaker and one of papers that seemed stronger. All the papers in each set were then arranged in a gradation from lightest to darkest in color. From each of these sets we selected 20 specimens that represented the full range of light to dark colors. The first 20 (weaker feel) we labeled group W, and the second 20 (stronger feel), group T (fig. 1). During the selection process we considered no other variables (such as paper thickness), nor did we incorporate other means to make the selection more objective (use of additional individuals during selection or use of blindfolds during the initial division of specimens into stronger and weaker paper sets). Nevertheless, we felt the 40-specimen final set met our goal of representing a range of apparent paper strength and condition. Following the selection process, the “W” and “T” designations were not considered variables in the study because instrument analyses were not used to gather data on actual paper strength.
Fig. 1.
Forty selected historical specimens prior to analysis.
 |
We used the darkness or lightness of the paper as our dependent variable and primary indicator of relative condition because of the common inclination to associate lighter-colored papers with those in better condition. While there are exceptions to this association (or that made between darker papers and poorer condition), for this project we assumed a dark-to-light scale to be a useful qualitative indicator of paper condition and probable strength. In his study of rag and wood pulp papers aged for 72 hours at 100�C, Rasch (1929, 501) commented that “in general, it is evident that papers which are impermanent in chemical or physical properties will also tend to discolor readily.” Destructive strength testing was not an option during this study due to the minimal area of paper specimens available.
3.2 L∗ DARK-TO-LIGHT DETERMINATIONS
All the 40 specimens were analyzed for Minolta Chroma Meter L∗ values by Irene Br�ckle at the Buffalo State College Art Conservation Department. The L∗ value gives an indication of where the value of the paper's color falls on a black-to-white scale. We interpreted L∗ data as relative “dark” (lower numbers) to “light” (higher numbers) values. Three sheets of Whatman no. 4 filter paper served as the background for all measurements. The instrument used was a Minolta Chroma. Meter CR-221 with a 45-degree illumination angle, 0-degree viewing angle, 3mm measuring area, calibrated against standard white (barium sulfate plate). Five measurements were made on each side of each specimen, taking care to choose the most even paper areas and avoiding print, dark inclusions, stains, or discolored paper edges. Determinations were made midway up the leaf whenever possible. More ideal L∗ values might have been obtained from multiple layers of paper cut from each specimen, prior to the hydroxyproline determinations. Using each specimen as its own background (instead of the Whatman no. 4 filter paper) would have helped overcome any transparency problems. This approach is recommended in similar future work. For the present, we consider the procedure used and the resulting L∗ values to be useful indicators of the relative “darkness” or “lightness” of the specimens tested.
3.3 CALCIUM AND pH
Calcium ppm values were from a single proton-induced x-ray emission (PIXE) determination per specimen, and surface pH values are the mean of 2 analyses per specimen. Both calcium and pH data were available from an earlier study (Barrett 1989). While there could have been some change in surface pH or L∗ values between 1989 and 1994, because of the relatively brief intervening period of natural aging of already old specimens, we have assumed any changes to be of minor significance. After selection of the 40 specimens and completion of L∗ and gelatin analyses it became apparent that 5 specimens lacked data either for pH or calcium, leaving complete data sets on 35 specimens.
3.4 GELATIN ANALYSIS METHOD
A microscale modification of the quantitative procedure given in TAPPI T-504 om-89, “Glue in paper (qualitative and quantitative determination)” (TAPPI 1991a), was used for the gelatin analysis.
3.5 SAMPLING
Samples were taken at three locations on each leaf. Three hydroxyproline determinations were made in separate batches for each location, and the nine values were averaged. Exceptions are noted in table 2. Areas that were stained, unusually discolored, or ink-covered were avoided. Preference in sampling was given in the following order: (1) inner margin; (2) head; (3) tail; (4) fore edge. Pieces of paper, about 3 mm2, were cut from a designated location until a roughly .04 g stock sample was accumulated. Each location stock sample was thoroughly mixed in a 1 dram snap-top vial, dried in a 105�C oven for 12 hours, placed in a desiccator containing anhydrous calcium sulfate, and capped after cooling. From the dried location stock sample, individual determination samples of 0.0025 g to 0.0100 g were rapidly weighed and the oven-dry weight recorded. Sample sizes were governed by the minimum amount of solution needed for the spectrophotometer cell (4 ml) and the necessity for the absorbance to fall between 0.2 and 1 for maximum accuracy.1
TABLE 2 DATA ON 35 HISTORICAL SPECIMENS
3.6 EXTRACTION
For an individual determination, a sample was weighted to the nearest 0.01 mg into a tared 1.5 ml polypropylene snap-top microcentrifuge tube. After the addition of HCl (1 ml, 0.1 M) to each tube in the batch, the rack of tubes was placed in a covered polypropylene box in a 100�C oven for 1 hour. After cooling, the liquid contents of each tube were quantitatively transferred using hot HCl (0.1M) to a hydrolysis tube (13 � 100 culture tube with Teflon-lined screw cap, Kimax Series 225-789). Liquid volume in the hydrolysis tube after transfer of extract and rinsing was 3 ml.2
3.7 HYDROLYSIS
HCl (3 ml, 12 M) was added to the 3 ml extract in each hydrolysis tube. The rack of tubes was placed in a covered polypropylene box in a 105�C oven for 24 hours.3
3.8 DECOLORIZATION & FILTRATION
Hydrolysates were decolorized by the addition of acid-washed activated charcoal (0.10 g) to the hydrolysis tube. HCl (200 ml, 12 M) was added to activated charcoal (25 g) with stirring. After settling, the supernatant was suctioned off and the charcoal was rinsed with deionized water (3 � 200 ml) by stirring, settling, and suctioning. The acid washing and water rinsing were repeated five times for one batch of charcoal. Final rinse was removed by vacuum filtration. The contents of each hydrolysis tube were then quantitatively transferred using deionized water to a microfunnel and vacuum-filtered through acid-hardened quantitative filter paper supported by a polyethylene frit directly into a 16 � 150 calibrated reaction tube (Teflon-lined screw-top culture tube calibrated by etching marks at approximately 4 and 8 ml, filling to the marks and calculating volumes based on the weight of water contained). Volume of liquid in the reaction tube after filtration was 10 ml.4
3.9 EVAPORATION
Reaction tubes were placed in a rack in a 100-105�C ethylene glycol bath and evaporated to dryness in a current of house air run through a trap containing cotton wool and distributed by a polyethylene manifold.5
3.10 PREPARATION OF CALIBRATION CURVE STANDARDS
Samples were read against a standard curve prepared from hydroxyproline (Sigma H 6002) solutions run with each batch. Aqueous solutions containing 6.5-120 μg/ml hydroxyproline were prepared in volumetric flasks by serial dilution and frozen in snap-top polypropylene tubes in 5 ml aliquots. Prior to a run, a 5 ml aliquot of each solution was brought to room temperature, and duplicate 13 � 100 calibrated reaction tubes (Teflon-lined screw-top culture tubes calibrated as above) were prepared by adding 0.5 ml solution to each tube. Final dilution of the calibration curve standards was 4 ml if the batch was a group of lightly sized papers and 8 ml if the batch was a group of heavily sized papers.6
3.11 HYDROXYPROLINE ANALYSIS
To each reaction tube containing the hydrolysate residue was added deionized water (0.5 ml, not added to calibration standard tubes), CuSO4 (0.3 ml, 0. 0.02 M), NaOH (0.3 ml, 2.5 M), and H2O2(0.3 ml, 4%, freshly prepared before use). Care was taken not to splash reagents high up on the walls of the tubes. Tube contents were mixed after each addition with a vortex mixer. Tubes were capped and kept at room temperature for 30 minutes. They were then placed in a 70�C water bath for 5 minutes to destroy excess H2O2, after which they were cooled to room temperature. Following the destruction of H2O2, each reaction tube was treated identically, i.e., the same amount of time elapsed between the addition of the color-forming reagents and the measurement of the absorbance of the solution in the spectrophotometer. To the cooled tube was added, with mixing after each addition, H2SO4 (0.8 ml, 1.5 M), and p-dimethylaminobenzaldehyde (Aldrich 15647-7, unrecrystallized) in 1-propanol (0.8 ml, 5%, freshly prepared before use). The tube was then capped and placed in a 70�C water bath for 50 minutes followed by cooling in a 20 � 2�C bath for 3 minutes. Within the next 2 minutes the tube contents were made up to the same volume as the calibration standards, the tube shaken; and the absorbance of the solution read in a Hewlett Packard Model 8452A diode array spectrophotometer at 560 nm using a reagent blank between every 10 readings (about once every 50 minutes).
3.12 SIZED REFERENCE STANDARDS
Sized reference standards were prepared from Whatman Chr4 chromatography paper, Barrett handmade text-weight flax paper, and Mosier handmade text-weight 98% cotton/2% flax paper. For each paper type, efforts were made to prepare standards that were lightly (under 1% gelatin), moderately (around 3% gelatin), and heavily (over 5% gelatin) sized. Each reference standard was prepared individually by applying, to an approximately 5mm2 piece of paper, a wt/wt aqueous photograde oven-dried gelatin (Rousselot) solution under conditions designed to minimize evaporation of the water. From the weight of the solution applied, the weight of the gelatin on the paper was calculated.
Nine paper reference standards were carried through the entire procedure with each batch of historical specimens run.7
3.13 OTHER TESTS
All of the papers tested negative for starch with iodine solution (Barrett 1989). No other tests (such as for thickness, fiber type, bluing, or bleaching) were undertaken.
3.14 STATISTICAL ANALYSIS OF DATA
Pearson correlations and their corresponding p-values were calculated using statistical analysis tools in Microsoft Excel.
4 DISCUSSION
The primary findings of this study are summarized in table 1. The P-values (probability values) for all correlations reported in table 1 were less than .01. Statistically, this result is considered to mean there is less than 1 chance in 100 that the data distribution was a random or chance occurrence. The normal P-value threshold for considering a result “beyond chance” would be .05 or less. Results are also reported in table 2, which give an overview of all related data, and in figures 2, 3, and 4, where an association can be seen between higher L∗ value (lighter color) and higher pH, calcium content, and gelatin content.
TABLE 1 CORRELATION VALUES OF 35 HISTORICAL SPECIMENS
Fig. 2.
Scatter plot of L∗ dark-light values vs. surface pH readings (35 specimens)
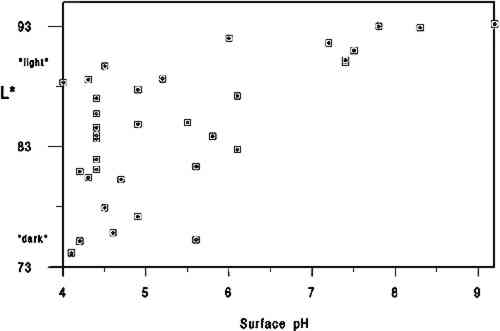 |
Fig. 3.
Scatter plot of L∗ dark-light values vs. PIXE ppm calcium determinations (35 specimens)
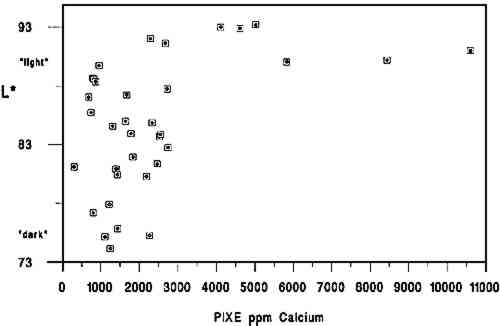 |
Fig. 4.
Scatter plot of L∗ dark-light values vs. weight percent gelatin values (35 specimens)
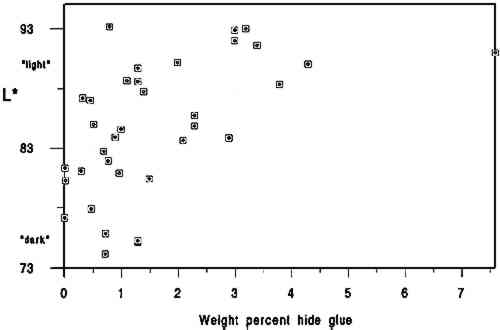 |
A brief introduction to interpretation of correlation values and their relative significance is warranted here for those readers unfamiliar with statistics. A perfect correlation between two variables would have a value of 1. The two variables would be considered to have a direct and systematic relationship with one another. A change in one variable correlates directly with changes in the other in a consistent manner. The correlation between the speed of a car and distance covered in one hour would obviously be 1, but the correlation between children's height and weight would be closer to .90. Data on two variables that are completely unrelated might have a correlation value of .1 or .2. In most carefully controlled scientific studies only values close to 1 would be considered to indicate a significant correlation. Lower values may be considered significant depending on the field of science, the material or variable being studied, and the nature of the sample pool. In a comparison of machine-made book papers subjected to accelerated aging in 1937 and later compared to 36 years of natural aging, Wilson and Parks (1980) considered correlation values from .75 to .98 fairly good to very good. However their sample pool consisted of machine-made papers, all made in 1937 at the same National Bureau of Standards experimental paper mill, while our 35 specimens were handmade, each in a different mill with different materials. In addition, they were all subjected to different storage conditions. We therefore feel that we can interpret the correlation values in table 2 as being more meaningful than might normally be the case.
We would summarize the results in table 1 by suggesting the correlation between L∗ and gelatin (.54) is strong enough to encourage further study of gelatin in paper permanence. The figures also suggest that no one variable is primarily responsible for the rise in L∗ value, but that they may all make a contribution. That is, the correlations between L∗ and pH (.64) and between L∗ and Ca (.48) also warrant more attention. Moreover, it appears that gelatin and pH, pH and calcium, and calcium and gelatin are all intercorrelated with one another with values of .45, .76, and .67 respectively. The three variables may turn out to be related in their contribution to paper permanence. One possible explanation is that calcium entered some papers when their gelatin sizes were made from parchment clippings, trimmings from limed skins, bones, and similar materials. Thus, gelatin might make a contribution to favorable pH in a manner separate from or in addition to the acid acceptor-buffering role suggested in the introduction.
In reviewing the figures and the data, we note that of the 10 lightest specimens, 8 are from the 10 highest in pH, 7 are from the 10 highest in calcium content, and 6 are from the 10 highest in gelatin content. Five of the 10 lightest specimens share top 10 values for pH, calcium, and gelatin, again indicating that the three variables may act together in promoting lighter paper color. Despite this clustering effect, the present data are not strong enough to support the notion that there are threshold values for each variable above which exceptional L∗ values or stability are evident.
Our data do not tell us the source of the gelatin, the methods used in its manufacture, or what impurities, such as fats, were present in the sizing solution. Nor do they tell us anything about the condition or length of the gelatin molecules, that is, whether excessive thermal or microbial degradation occurred either before or after the paper was sized. They simply give us a quantitative measurement of the amount of hydroxyproline present in the extracts of the papers, from which we have calculated gelatin content (estimated hide glue content).
An important question is whether the amounts of hydroxyproline measured in the extracts of the historical specimens are indicative of the amounts of gelatin originally applied. Could gelatin have been lost from the surface of the paper after sizing? We cannot know the storage conditions of the papers we tested, but they did not show signs of damage from water or microbes. Could deterioration of the gelatin molecule over the centuries have given inaccurate results? Deterioration might consist of breaking of peptide bonds. The strongest peptide bonds have been suggested to have a geologically short lifetime of 104 to 106 years (Hare 1969). Gelatin is hydrolyzed more readily and under less harsh conditions than many proteins (Leach and Eastoe 1977), such as wool or silk (Courts 1980). If this sort of deterioration has taken place, the chains of gelatin would be shorter but the amount of hydroxyproline present would still be the same.
Hydroxyproline itself is a moderately stable amino acid. It is not destroyed during 24-hour acid hydrolysis at 105�C, nor is it oxidized by heating at 105�C in the presence of oxygen for at least 8 hours. Free hydroxyproline has been found in the vicinity of fossilized collagenous materials 1 million years old (Ho 1965), although it is not known how long it had been liberated from the presumably protective collagen structure. All hydroxyproline had been lost from collagenous materials 150 million years old (Armstrong and Tarlo 1966). It seems unlikely to the investigators that deterioration of the hydroxyproline molecule under average storage conditions would be significant in the time span of our study, but there are no data to directly support this supposition.
It is known that gelatin becomes increasingly insoluble when heated at high temperatures or for prolonged periods (Leach and Eastoe 1977). In our own aging test (80 � 2�C, 0% RH for 2 months), we found the percent recovery of gelatin from the aged papers to be 6 � 2% lower than from unaged papers. Studies of gelatin films indicate that films conditioned at quite mild temperatures (45�C) and varying relative humidities become less soluble with increasing RH (Finch and Jobling 1977). These changes are attributed to either denaturation or cross-linking in the protein and may be related to the conditions under which the film was originally dried. If the solubility of the gelatin on the paper has decreased, its extraction from the paper will be incomplete and the results too low. We have shown that our recovery of gelatin was 96 � 5% in new paper reference standards dried at 105�C for 12 hours. Our results for the historic paper shown in table 2 are based on that figure. Unfortunately, we have no data to establish actual percent recovery from each historic specimen. Because it is unlikely that there are significant amounts of nitrogen from sources other than gelatin in these papers, a comparison of percent hydroxyproline recovered versus total nitrogen content via the Kjeldahl method (TAPPI 1991b) might be more useful in this regard than aging tests, whose results could not be applied with much certainty as a means of replicating the effects of long-term natural aging on gelatin in sized papers.
Assuming the hydroxyproline values reported here are indicative of the amounts of gelatin originally applied, our results suggest, as stated above, an association between lighter paper color and higher gelatin content. This finding would appear to contradict the tendency (mentioned in relevant literature above) of gelatin-sized papers to darken more than unsized counterparts during accelerated aging (Rasch 1929; Barrett 1989). The accelerated aging conditions used by these researchers may have caused reactions resulting in a large color change that would not occur during long-term natural aging. Since all of the darkest papers in the current study were characterized by their low gelatin content, this apparent discrepancy will remain until additional research can resolve it.
The key question at hand, from our perspective, is whether the chemical and physical systems of a historical leaf made with the characteristics we currently consider essential in a long-lasting paper (i.e., a neutral or alkaline pH, alkaline reserve, high alpha cellulose content, and low levels of iron and copper) could have been altered by the addition of high-quality gelatin in a manner that gave the paper significantly improved permanence. And if so, why? The answer to these and other questions related to the role of gelatin in paper permanence must be left for future work.
5 CONCLUSIONS
- Correlations between pH, calcium content, and gelatin content in the 35 historical specimens tested suggest all three variables make a contribution to increasing L∗ value (lighter color). This finding, in turn, suggests the three may interact in a chemical and physical system that has an overall positive effect on the long-term stability of paper.
- It is not possible to state from these data that any one variable makes a more significant contribution to higher L∗ values.
- Gelatin is an important component in the chemical and physical systems of papers made in Europe between 1400 and 1800 that deserves additional research attention. Such work can be significant to conservators of rare books and works of art on paper with regard to the advisability of washing, aqueous deacidification, and resizing treatments.
6 FUTURE WORK
In combination with additional work to demonstrate that gelatin does, in fact, occur at higher levels in best present-day condition historical samples, accelerated aging studies will be necessary to understand fully the interrelationship between gelatin and the other components in paper and their respective contributions to paper permanence. Future accelerated aging projects should incorporate exposure to pollutant gasses as well as temperature and humidity cycling to understand more fully the role of gelatin in paper stability. Research of particular interest to conservators will include studies on gelatin's buffering capacity, the relationship between gelatin and alum, and the differences between modern gelatins, historical gelatins, and parchment size prepared in the conservation laboratory. Such work will have a direct bearing on treatment decisions. Common treatment-related questions are: When is resizing important? What type of gelatin should be used? Will a starch, synthetic, or cellulose-derivative size yield the same results as gelatin? And how do the benefits associated with alkalinization/deacidification compare or relate to the importance of resizing? We hope these and additional related questions will be investigated by interested researchers in the not-too-distant future.
ACKNOWLEDGEMENTS
Our thanks go to the Grinnell College Chemistry Department and Jim Swartz for access to laboratory facilities. Special thanks go to Irene Br�ckle for making the required Minolta Chroma Meter L∗ determinations. The Samuel H. Kress Foundation provided the major portion of funds required for this project. We thank Jodie Plumert for her recomendations during preparation of the manuscript and JAIC reviewers and editors whose careful readings and recommendations made a significant improvement in the quality of this report.
NOTES
1. The TAPPI sample size is 1 g. TAPPI oven-dry weight is calculated based on the moisture content of a separate 2 g specimen as determined in accordance with TAPPI 412 (TAPPI 1991c). Our results showed no significant differnce in the % hydroxyproline recovered from paper reference standards that had been dried at 105�C for 12 hours and those that had not been dried.
2. The TAPPI extract is filtered through hardened filter paper.
3. TAPPI hydrolysis conditions are overnight at 110�C.
4. Decolorization with activated charcoal is not used in the TAPPI procedure. Our results showed no significant loss of hydroxyproline from paper reference standards treated with charcoal when run against calibration curve standards made from the same gelatin used to size the paper reference standards.
5. TAPPI evaporation is done at 110�C under nitrogen. We found no significant difference in % hydroxyproline measured in hydroxyproline solutions evaporated under house air at 100-105�C and reconstituted with water and tubes containing the same solutions and not evaporated at all.
6. TAPPI calibration curve hydroxyproline standards are not frozen. Our results showed no significant difference in the absorbances of hydroxyproline standards that had been stored frozen and those that had not. Percent recovery of hydroxyproline from the paper reference standards continued to be similar throughout the batches run, averaging 96 � 5% over the course of the experiment. Had the concentration of the calibration curve standards been changing, this recovery calculation would have so indicated.
7. The TAPPI procedure requires that three reference standards be tested in triplicate each time a batch is run. The reference standard is a solution of the same glue used to size the paper being analyzed if possible. Otherwise, good-grade hide glue or purified calfskin gelatin are recommended. There is no procedure for preparing paper reference standards. Because we modified early steps, we felt that paper reference standards were necessary in order to monitor the entire procedure.
REFERENCES
Armstrong, W. G., and L.B.H.Tarlo. 1966. Amino acid components in fossil calcified tissues. Nature210:481–82.
Barrett, T.1989. Early European papers/contemporary conservation papers. The Paper Conservator13:1–108.
Barrett, T.1992. Evaluating the effect of gelatin sizing with regard to the permanence of paper. In Conference papers Manchester 1992, ed.S.Fairbrass. Manchester: Institute of Paper Conservation. 228–33.
Barrow, W.J.1972. Manuscripts and documents: Their deterioration and restoration. Charlottesville, Va.: University Press of Virginia. 35–37.
Barrow, W. J.1974. Physical and chemical properties of book papers 1507-1949. Richmond, Va.: W.J. Barrow Research Laboratory.
Courts, A.1980. Properties and uses of gelatin. InApplied protein chemistry, ed.R.A.Grant. London: Applied Science Publishers. 1–29.
Erickson, H.1994. Personal communication. Graduate School of Library and Information Studies, University of Texas at Austin, Austin, Tex. 78712
Finch, C.A., and A.Jobling. 1977. The physical properties of gelatin. In The science and technology of gelatin, ed.A. G.Ward and A.Courts. London: Academic Press. 249–94.
Hare, P.E.1969. Geochemistry of proteins, peptides and amino acids. In Organic geochemistry, ed.G.Eglinton and M.T.J.Murphy. Berlin: Springer-Verlag. 438–63.
Ho, T.1965. The amino acid composition of bone and tooth proteins in the late Pleistocene mammals. Proceedings of the National Academy of Sciences54: 26–31.
Inaba, M., and R.Sugisita. 1988. Permanence of washi (Japanese paper). In Preprints of the contributions to the Kyoto Congress, 19–23 September 1988, ed.J.Mills et al. London: International Institute for Conservation of Historic and Artistic Works. 1–4.
Leach, A. A., and J. E.Eastoe. 1977. The chemical examination of gelatins. In The science and technology of gelatin, ed.A.G.Ward and A.Courts. London: Academic Press. 475–506.
Rasch, R. H.1929. A study of purified wood fibers as a papermaking material. Bureau of Standards Journal of Research3(February):469–506.
Rasch, R. H.1931. Accelerated aging test for paper. Bureau of Standards Journal of Research7:465–75.
Rasch, R. H., M. B.Shaw, and G.W.Bicking. 1931. Highly purified wood fibers as papermaking material. Bureau of Standards Journal of Research7(August):765–82.
Rasch, R.H., M. B.Shaw, and G. W.Bicking. 1933. A study of some factors influencing the strength and stability of experimental papers made from two different sulphite pulps. Bureau of Standards Journal of Research11(July): 7–23.
Swartz, J.1994. Personal communication. Grinnell College, Department of Chemistry, Grinnell, Iowa 50112.
TAPPI. 1991a. Glue in paper (qualitative and quantitative determination). T504 om-89. In TAPPI Test Methods 1991. Atlanta, Ga.: Technical Association of the Pulp and Paper Industry.
TAPPI. 1991b. Organic nitrogen in paper and paperboard. T418 om-90. In TAPPI Test Methods 1991. Atlanta, Ga.: Technical Association of the Pulp and Paper Industry.
TAPPI. 1991c. Moisture in paper and paperboard. T412 om-90. In TAPPI Test Methods 1991. Atlanta, Ga.: Technical Association of the Pulp and Paper Industry.
Wilson, W. K., and E.J.Parks. 1980. Comparison of accelerated aging of book papers in 1937 with 36 years natural aging. Restaurator4(1):1–55.
Wilson, W. K., and E.J.Parks. 1983. Historical survey of research at the National Bureau of Standards on materials for archival records. Restaurator5(3–4):191–241.
AUTHOR INFORMATION
TIMOTHY BARRETT is an associate research scientist at the University of Iowa Center for the Book (UICB). As program director for UICB Paper Facilities, his duties include teaching papermaking history and technique at the School of Art and Art History and overseeing research and conservation paper production at a separate center facility. Address: UICB Paper, M109 Oakdale Hall, University of Iowa, Iowa City, Iowa 52242.
CYNTHEA MOSIER received a B.A. in biology from the University of California at Santa Cruz in 1971. Between 1972 and 1988 she served as staff research associate, Chemistry Board of Studies, at the same university. She studied under Timothy Barrett in the classroom for one year and worked as his research associate for an additional year. She is currently an apprentice in book and paper conservation under Pamela Spitzmueller in the Book Conservation Laboratory, University Libraries, University of Iowa.
This paper was presented at the 1994 AIC annual meeting, Book and Paper Specialty Group session, as “The role of gelatin in paper permanence 2” and published in the 1994 AIC Book and Paper Group Annual under the same title. The present version includes additions and revisions. The first work by Barrett on essentially the same topic is listed in the References above (Barrett 1992).
Section Index |