EMBEDDING PAINT CROSS-SECTION SAMPLES IN POLYESTER RESINS: PROBLEMS AND SOLUTIONS
MICHELE DERRICK, LUIZ SOUZA, TANYA KIESLICH, HENRY FLORSHEIM, & DUSAN STULIK
ABSTRACT—Polyester resins have been commonly used in art conservation for embedding paint cross sections prior to microscopic and analytical studies. These resins set rapidly without heat, are clear, polish readily, and microtome easily. While they work well for embedding most paint samples, they pose problems for some specific samples. The polyester embedding media have been found to dissolve wax, some organic colorants, and fresh natural resin layers in cross sections. The embedding resin also wicks into porous samples of low binder content. At times, infiltration can be desirable for its consolidating effects, but it can also interfere with the determination of the type of binder in the sample by producing blotchy, uneven staining results and by obstructing infrared analysis. Alternate embedding materials and procedures are discussed in this paper. One method to prevent the infiltration of embedding media is presented in which the samples are precoated with a thixotropic acrylic gel before embedding. Optimal microtoming techniques are also presented.
1 INTRODUCTION
Polyester resins were introduced in the 1940s. Because of their transparency and ease of preparation, they were soon used for the encapsulation of natural history objects (Purves and Martin 1950). Although it was later discovered that the long-term life of the polyester was not appropriate for museum objects (Meurgues 1982), polyester resins are still commonly used by schools, scientists, and hobbyists for embedding and casting many objects. Polyester resin is also routinely used as a medium for embedding multilayer paint cross sections from art objects (Plesters 1956; Wolbers and Landrey 1987; Tsang and Cunningham 1991). In a recent detailed comparison of 14 types of embedding media, polyester resins were found to be the most satisfactory for embedding cross sections (Waentig 1993).
The preparation of cross-section samples has become standard practice for the examination of painted surfaces in art conservation and forensic analysis. Large, sturdy cross sections can often be polished and microtomed without embedding, that is, surrounding the sample with another material. However, tiny or fragile cross-section samples need to be embedded in a supporting medium to hold them together and in the correct orientation for examination. The mounted cross section is a valuable source of information for a painting's layered structure, pigments, and technique. Relating the structure to its materials can also provide indications of relining, overpainting, or other restoration procedures.
In the analysis of mounted paint cross sections for their pigment components using light microscopy and scanning electron microscopy with energy dispersive x-ray spectrometry (SEM/EDS), no apparent problems have been cited with polyester embedding resins. However, the determination of the types of binder in a sample can require other techniques, such as staining (fluorescent and nonfluorescent) and infrared microspectroscopy. With these techniques, it has become apparent that polyester resin and other polymeric embedding media can interact with some specific types of samples during the embedding process. This interaction may produce interferences in the analysis of the organic portion (binder and coatings) of the sample, and caution must be used when any results are interpreted.
Some samples may contain components that are soluble in the liquid prepolymer. Godla (1990) pointed out that polyester resin can dissolve some waxes in furniture finish samples, often making it difficult to examine wax finishes after embedding. (Bischoff 1994) has observed that some organic dyes on inorganic carriers in modern (post-1850) pigments are solubilized by polyester resin. In addition, polyester resin was found to dissolve fresh (less than one-year-old) natural resin samples of dammar, mastic, and copal (Derrick et al. 1992). Aged or oxidized resin layers on furniture finish cross sections did not seem to exhibit the same solubility problem.
Polyester resin has also been found to soak into or infiltrate porous, low-binder samples, as observed by (Baker et al. 1989) for embedded paper cross sections. In many cases, this impregnation is beneficial because it consolidates the sample, making it less fragile and easier to polish or microtome. However, embedding media infiltration also has unwanted characteristics. It may change the appearance of the sample and hinder infrared spectral analysis of the sample components. Penetration of the resin into the sample also interferes with the use of stains (fluorescent and nonfluorescent) for binder identification in cross sections by coating the particles in the sample and thereby inhibiting interaction of the stain with the binder. Thus, it is important to recognize that infiltration can occur with some embedded samples and to be able to prevent infiltration whenever it is undesirable. This paper will illustrate these problems and discuss alternate embedding materials and procedures.
2 EMBEDDING MATERIALS
An ideal embedding resin should meet the requirements listed below when used for the analysis of organic components in paint cross sections obtained from works of art. Different criteria are used when samples are embedded for other techniques such as petrography (Reedy 1994) or SEM analysis.
- The mounting medium and its solvent should not react with, soak into, or otherwise interfere with the analysis of the binder in the sample.
- The medium should cure at room temperature. The curing process should not be exothermic, since heat may adversely affect the organic materials or binders in the sample.
- For samples that are to be analyzed by infrared microspectroscopy, the resulting embedment and sample should be easy to section using a microtome. Transmitted infrared analysis requires a section of 1–10 μm in thickness for an optimum spectrum.
- The medium should be transparent to ensure proper orientation of the sample for microscopic examinations as well as for positioning the embedded specimen in the microtome jaws.
- The medium should not shrink upon curing, because shrinkage will apply stress to the sample and may cause problems during microtoming.
The advantages and disadvantages for several types of polymeric materials tested for embedding paint cross sections are summarized in table 1. Figure 1 shows example blocks of each of the polymeric media listed in table 1. Many other types and brands of embedding media are available; some are discussed in the text. Of the four major types of embedding resins tested, polyester was found to be the best for embedding and microtoming most types of art materials.
TABLE 1 TYPES AND BRAND NAMES OF POLYMERIC MEDIA TESTED FOR EMBEDDING AND MICROTOMING OF PAINT CROSS SECTIONS
Fig. 1.
Example blocks of polyester, epoxy, acrylic, and wax embedding media for paint cross sections corresponding to table 1. Break-away molds and small, flexible silicone rubber molds are shown.
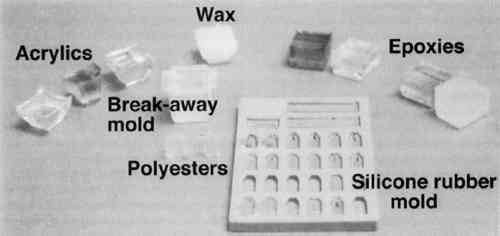 |
One polyester embedding resin commonly used in art conservation is Bio-Plastic. Similar polyester resins are sold under the brand names of Caroplastic, Castolite, Castoglas, and Vestopal W (see Suppliers). Polyester embedding resins contain a polyester prepolymer dissolved in styrene monomer to form a solution of appropriate viscosity (Horie 1987). When combined with a methyl ethyl ketone peroxide catalyst, a crystal clear, uniform, solid block is produced that is readily polished and easily microtomed. The resin cures overnight at room temperature or in a few hours at slightly elevated temperatures. It shrinks minimally during curing at room temperature and slightly more when cured with increased temperatures. It is cheap, readily available, easily prepared, and only moderately toxic. These many positive features give polyester resin several advantages over the other embedding resins on the market (e.g., acrylics, and epoxies) and account for its popularity as an embedding medium for paint cross sections.
While the polyester resins typically are used for paint cross sections in art conservation, forensic scientists generally use epoxies, acrylics, and cyanoacrylates. We found that the epoxies tend to be yellow in color as well as too hard and brittle to microtome sections at the thicknesses of 1–10 μm required for infrared analyses. The acrylics were clear and colorless, but they were very exothermic and shrank significantly upon curing. One acrylic embedding material became so hot during curing that it melted the 1 in. plastic mold. Both the epoxies and acrylics had dissolution and infiltration problems similar to those of the polyesters and thus are not recommended over the polyesters.
One method found in forensic science is the use of a drop of cyanoacrylate glue (e.g., Super Glue or Krazy Glue) to mount a paint chip on the end of a small wooden dowel (Cartwright et al. 1977). While it was difficult to get the sample oriented correctly, this method worked well for microtoming the sample. However, we still experienced infiltration problems with some samples, and dissolution of components is still a potential problem when the sample contains acrylic paints, waxes, or fresh resin layers. Krazy Glue gel, a thickened cyanoacrylate specifically designed to remain on the surface of porous substrates, also soaked into the outer layer of all plaster samples we tested.
Gelatin is another material that has been used successfully in the forensic field for embedding paint cross sections (Wilkinson et al. 1988). The procedure used by Wilkinson involved freezing the sample in a gelatin block for cryogenic microtoming. Afterward, the thin section was warmed and the gelatin was washed away, leaving just the thin section of sample. This method should be effective for samples that are not susceptible to water, such as wax cross sections. However, we did not test this method in our lab because gelatin could potentially interfere with the positive identification of glue binder, albumin varnish, or other protein-aceous media. In addition, glue or gum binders in the samples may be susceptible to damage or alteration by the water solvent in the gelatin.
The biomedical field uses several types of embedding media (e.g., glycol methacrylate, methyl cellulose, acrylamide, and epoxy) for cytology studies by electron microscopy. These studies often require ultrathin microtomy for producing thin sections of less than 1 μm thick. A low-viscosity embedding solution, usually water-miscible, is used to allow easy penetration of the resin into the sample. For the biomedical field and for many other purposes, penetration is desirable since it will stabilize the sample and help maintain the specimen's shape. However, since we were trying to eliminate infiltration of the resin, we did not test any of these low-viscosity media.
One embedding option is the use of low melting-point waxes such as Paraplast. This wax-polymer mixture melts at approximately 60�C and solidifies rapidly. The wax does not pose any infiltration or dissolution problem to the samples, but it is unlikely that surface wax layers would be detectable. While wax embedments are too soft to be polished, they can be microtomed easily. Wax is opaque, so it is critical to know the positioning of the sample before microtoming. It is also important to work quickly when pouring a mold with the hot wax because delays can result in a block that contains many bubbles. One method that shows potential has been developed by (Wolbers 1993). A wax is mixed with an inorganic salt, such as potassium bromide, thus increasing the hardness of the wax and making the mixture more transparent.
Similar to waxes are hot-melt adhesives, which are primarily polyethylene-based polymers. These adhesives range in transparency from semiclear to opaque. They are hard and rubbery at room temperature. Hot-melt adhesives are not recommended for general embedding purposes because they solidify rapidly (less than 60 seconds), making it difficult to orient and manipulate the sample. These adhesives did not infiltrate the samples, but they were slightly too soft for easy microtoming and had to be cooled in order to obtain thin sections. Optimally, this process requires a cryogenic microtome, since cooling samples prior to microtoming in a freezer or with a cryogenic spray can result in water condensation on the sample.
Several silicone rubbers have been tried for embedding samples. To date, we have not found any that are hard enough to be microtomed or polished. However, we are still looking for other silicone materials that may work.
Since the use of nonpolymeric mounting media would eliminate the dissolution and infiltration problems associated with the poly-meric media, we tested several inert materials, such as salts (BaF2, AgCl, KBr), cork, indium, and gallium. The inorganic salts barium fluoride, silver chloride, and potassium bromide were powdered and then placed in a pellet die with the sample. The salt was pressed into a transparent pellet with no apparent distortion to the sample. At this point, it was difficult to proceed further. We were not able to micro-tome any of the pressed pellets without the salt crumbling into a powder. And while the salts themselves could be easily polished, they did not polish at the same rate as the sample and tended to disintegrate.
Problems also occurred with cork embedments. A small slit was made in a piece of cork with a razor blade, the sample was inserted, then the sample area was sealed with cyanoacrylate adhesive (such as Krazy Glue). The cork itself was difficult to microtome because it was so soft. It tended to move and compress with each microtome slice. However, the area of cork and sample coated with cyanoacrylate exhibited some additional stiffness that allowed it to be microtomed into sections approximately 10 μm thick. We were not able to polish the sample, and it was necessary to use a fairly large sample (1 � 2 mm) in order to position it correctly. The same dissolution problems mentioned above for cyanoacrylate would still apply.
Malleable metals, gallium and indium were tried for embedding. In this test, two small pieces of metal were placed above and below the sample. Then some pressure was applied to compress the metal around the sample. The metals held the samples well for microtoming, but it was difficult to orient the samples properly because of the opacity of the metals. Since the metals are also expensive and toxic, this method was discarded.
Because an alternate embedding material was not found that had as many advantages as the polyesters, we focused our efforts on understanding the interaction of the polyester resin with the sample in hopes of finding conditions, samples, and methods for which the polyester works well.
3 EMBEDDING PAINT CROSS SECTIONS WITH POLYESTER RESIN
3.1 PROCEDURE
To embed a typical paint sample in polyester media (brands noted in table 1), six drops of catalyst (methyl ethyl ketone ether) are mixed thoroughly with 10 ml of liquid polyester/ styrene resin. The transparent resin is initially light blue in color. It turns yellow when the catalyst is well mixed and quickly becomes colorless as the reaction proceeds (Ward's Natural Science 1990). Excess catalyst will speed up the curing process but will also make the final mount more brittle and difficult to microtome. A mold is initially half-filled with the well-mixed embedding medium and cured at room temperature for 3–6 hours. A representative portion of the sample containing all the layers is transferred to the hardened base layer in the mold with forceps or a probe and positioned in the desired orientation. A label is placed on the opposite end of the mold; then both the label and the sample are covered slowly with freshly prepared polyester embedding medium. The embedment is cured and nontacky within 24 hours. When microtoming the sample for infrared microanalysis, the best results are obtained by allowing the embedment to set 36–48 hours before slicing. The medium continues to cure slowly over time (Demmler 1980). After one month, the microtoming becomes noticeably more difficult, and the samples tend to crumble. The bottom and top halves of the block should be prepared within a few days of each other to prevent a hardness differential between halves that interferes with microtoming.
Initially, plastic peel-away molds (1 in. cube, fig. 1) were used for embedding. However, because the presence of excess medium stresses the sample during microtoming, most of the plastic around the sample has to be trimmed away to reduce the contact area with the microtome blade. A razor blade or diamond saw is used to trim away all but a supporting cone of plastic with at most 1 mm of embedding media surrounding the sample at the cutting surface. Since trimming can be very time consuming, a switch was made to Pelco silicone rubber embedding molds (7�15�3 mm, (fig. 1). These molds produce small embedments with trapezoidal tips that do not require much trimming. These small embedments, while good for microtoming, are difficult to hold in a level position for polishing samples.
3.2 INFILTRATION
The penetration of the liquid embedding resin into the interior of a paint sample can occur when there are voids or open spaces in the sample. Typical porous samples are stones, pigments, papers, and textiles. Paints are porous when the amount of binder is low enough that it does not fill the void spaces around the pigment particles (Hansen et al. 1993). The embedding resin can then seep into the sample, fill these spaces, and, in this process, coat the particles. Infiltration can occur with matte or porous paints often found in wall paintings, polychrome sculptures, ethnographic objects, and glue gessos. Sample impregnation consolidates the sample, producing a smooth block that is readily polished and easily microtomed into intact thin sections. However, for analysis of the binder or organic components in the sample, infiltration may be undesirable, and, in some cases, steps should be taken to prevent the infiltration from occurring.
If several samples of a porous material are to be embedded for analytical studies of the media, it is prudent to test only one sample initially to see if infiltration occurs and, more important, whether it obstructs analysis of the sample. Depending on the type of analysis is to be done, it is possible that infiltration of the media will not cause a problem, but the analyst must recognize that the resin may be in the sample and take that into account in any interpretation. Infrared spectroscopy is more sensitive to the presence of the infiltrated resin than visual or microscopic examination and thus, when IR is used, it may be important to prevent the resin from infiltrating.
Visual examination of a paint cross section can often detect infiltration of the embedding resin due to the discoloration or darkening of the sample. This infiltration is particularly noticeable for white paints and grounds. Samples that visually appear very white and opaque before embedding can take on a darker, transparent appearance after resin penetration. Due to the presence of embedding resin inside and outside the sample, there is less contrast at the sample edges, and the edges may seem poorly defined. For example, two small portions of a sample from a polychrome sculpture were embedded separately, one in a thickened acrylic medium and one in polyester (figs. 2, 3). The photomicrographs show that the sample in the polyester medium experienced penetration of the resin, while the sample embedded in the acrylic did not. The acrylic embedded sample has very well-defined edges, and the opaque white ground remained white after embedding. The polyester embedded sample exhibits a more transparent, darker ground layer.
Fig. 2.
Photomicrograph of embedded paint cross section that is not infiltrated with embedding medium. The edges are well defined, the sample appears opaque, and except for pigment variation, the colors are uniform. 75�.
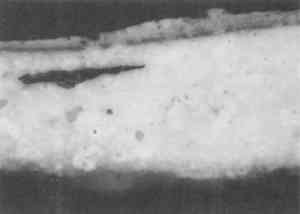 |
Fig. 3.
Photomicrograph of embedded paint cross section from the same sample shown in figure 2. However, in this example the paint is infiltrated with embedding medium. 75�
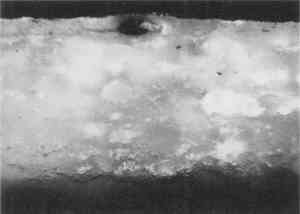 |
Infiltration of the embedding resin into the sample is readily and conclusively recognizable when infrared microspectroscopy is used to analyze the sample. An infrared spectrum of a cross section will contain absorption bands for each component in the analysis area. Thus, if a paint sample is infiltrated, the spectrum will show absorption bands that correspond to the polyester, the binder, and the pigment. Since the polyester resin absorbs strongly in the infrared region, and since the binder is likely to be in a low concentration, it may be difficult or impossible to identify the binder due to the large absorption bands overlapping and hiding the smaller ones. Spectral subtraction may or may not be successful in removing the absorption bands due to the resin. Figure 4 shows an infrared spectrum of an area of an infiltrated paint cross section along with a reference spectrum of a polyester resin (Caroplastic). Infrared spectroscopy confirms infiltration of the resin by the presence of the polyester absorption bands in the spectrum for the sample.
Fig. 4.
Infrared spectrum of paint cross section sample (top) and Caroplastic brand polyester resin (bottom). The spectrum for the paint sample contains absorption bands corresponding to the embedding resin (marked with ∗). This spectrum shows that the embedding resin has soaked into the paint sample.
 |
Resin-infiltrated samples do not uniformly retain fluorescent or nonfluorescent stains even though the binder is uniformly distributed throughout a layer. This characteristic is due to the coating of the paint particles by embedding resin, which inhibits the stain from reaching the sample and results in a blotchy appearance. Figures 5 and 6 illustrate that the infiltration problem occurs with low binder content samples. In a test sample prepared with a 40 wt% glue/gypsum concentration, no indications for penetration of the polyester embedding resin were found. The paint cross section stained uniformly and brightly with fluorescein isothiocyanate (FITC) under fluorescent light (fig. 6). A corresponding sample prepared with 5 wt% glue/gypsum was clearly infiltrated with the polyester resin. Figure 5 shows its uneven staining pattern with FITC.
Fig. 5.
Photomicrograph of embedded paint cross section containing 5 wt% glue in gypsum after staining with FITC. This low binder content results in a porous sample that rapidly wicks in embedding resin. The resin coats the sample particles and causes uneven fluorescent staining of the protein binder with FITC. 37�
 |
Fig. 6.
Photomicrograph of embedded paint cross section containing 40 wt% glue in gypsum after staining with FITC. The high binder content results in a nonporous sample that is not infiltrated by the embedding resin. The FITC stains the protein in the sample uniformly and fluoresces a bright yellow color. 37�
 |
Based on infrared analysis of a sequence of mixtures, it was found that samples with concentrations of 20 wt% or less of glue in gypsum experience infiltration to varying degrees. This finding verified that penetration occurs in low binder content samples. The specific binder-to-pigment ratio at which infiltration occurs depends on the type of binder and pigment present in the sample.
3.3 CURE TIME
After the polyester prepolymer is mixed with the catalyst, it has a fluidity similar to corn syrup. The resin flows readily around the sample, and any bubbles migrate to the surface. However, since this fluidity may also enhance the penetration of the resin into the sample, a test was done to permit the mixed resin to cure partially before pouring the second half of the mold. After 41 minutes cure time, the polyester was so thick that it did not form a uniform layer over the sample. However, infrared analysis of a paint sample coated with the polyester after 39 minutes of curing showed that the paint sample was infiltrated with the embedding material (fig. 7). Thus, it is not practical to use cure time to prevent the infiltration of the resin.
Fig. 7.
Infrared spectra of Caroplastic polyester resin (bottom) and paint cross section (top) for which the top embedding layer of Caroplastic was poured over the sample 39 minutes after the catalyst was added to the resin solution. Even though the embedding resin was extremely thick when it was placed on the top of the sample, the resin still infiltrated the paint sample. This infiltration is shown by its infrared spectrum, which contains absorption bands corresponding to the embedding resin (marked with ∗)
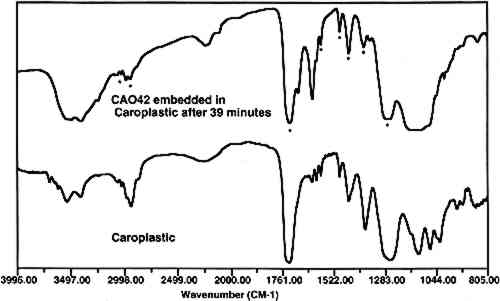 |
3.4 BARRIER METHODS
Since polyester resins are the embedding media of choice, several barrier methods were investigated to encapsulate the samples and prevent the polyester from infiltrating. These methods use a noninterfering material to surround the sample with a thin, impenetrable layer prior to embedding the sample in the polyester, thus keeping the good qualities of the polyester without allowing it to touch the sample.
The barrier methods tried for treatment of the samples before embedding in the polyester resin are shown in table 2. Only the last two of these methods were effective at preventing the infiltration of the polyester resin. However, the successful result was hard to achieve with the toluene thickener, because the film would sometimes remain rubbery rather than harden. Using fumed silica as a thickening agent is recommended as the best option tested.
TABLE 2 BARRIER METHODS TESTED TO PREVENT THE INFILTRATION OF POLYESTER RESIN INTO THE SAMPLES
Figures 8 and 9 show photomicrographs of an unstained and FITC-stained paint cross section that had been coated in wax prior to embedding. The sample microtomed easily but the wax did not prevent the infiltration of the resin. In practice, the resin dissolved the wax and created a halo image around the sample.
Fig. 8.
Photomicrograph of embedded paint cross section that was coated with wax prior to embedding in polyester resin. The wax did not prevent the infiltration of the resin. In fact, the resin dissolved the wax, creating the halo effect seen around the sample. 37�
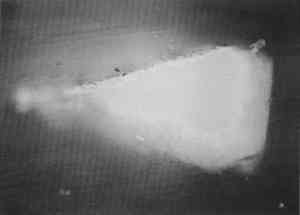 |
Fig. 9.
Photomicrograph of fluorescent image of FITC-stained sample shown in figure 8. The nonuniform appearance of the fluorescent stain is due to the infiltration of the polyester embedding resin into the sample. 37�
 |
Shrink-wrap was tested for encapsulating samples. However, the high temperatures required to shrink the material are not recommended for sample preparation; in a few attempts, the samples could not be prepared without bubbles being trapped inside the plastic. The presence of bubbles keeps the sample from being held uniformly and makes microtoming difficult.
The next test used a sputter coater to coat samples with greater than a 5-nm-thick layer of gold. Several samples of two types (plaster and paint facsimile with glue ground) were prepared by this method. The results were inconsistent within sample type; some were infiltrated by the polyester, and some were not. It appeared that the smoothness and lack of crevices in the sample were important for success, since complete coating of all surfaces is necessary to keep out the polyester. Additionally, careful handling of the sample after coating was required, because, in some cases, a small portion of the fragile coating would attach to the forceps and come off the sample. Figures 10 and 11 show photomicrographs of an unstained and FITC-stained paint cross section of a sputter-coated sample that experienced resin infiltration.
Fig. 10.
Photomicrograph of embedded paint cross section that was sputter-coated with gold before embedding in polyester resin. The gold layer did not prevent the infiltration of the resin, as can be seen by the transparent darker edges in the ground layer of the sample. 37�
 |
Fig. 11.
Photomicrograph of fluorescent image of FITC-stained sample shown in figure 10. The nonuniform appearance of the fluorescent stain is due to the infiltration of the polyester embedding resin into the sample. 37�
 |
Completely sealing samples in an aluminum foil sandwich with crimped edges was also tried. This method was extremely time-consuming and resulted in an opaque, difficult-to-position sample. Resin infiltration with this method was inconsistent, and thus it is not recommended.
Several conservation treatments for papers and painting relinings use nonpenetrating gel-like solutions such as methyl cellulose (Baker 1984), acrylic dispersions thickened with toluene (Keyser 1981; Mehra 1984), and acrylic dispersions thickened with fumed silica (Byrne 1984). Some of these methods were tested for encapsulating the samples and found successful. Many other thixotropic, quick-drying solutions may also work in the same manner. Of the gels tested, the cellulose ether (Klucel G) film dissolved in the polyester resin. The toluene-thickened acrylic (Rhoplex AC-33) was found to retain toluene, resulting in a pliable film that tended to stretch during microtoming. However, when the toluene-thickened gel was allowed to dry thoroughly (a day or more), a tough, clear film was formed over the sample. The acrylic (Rhoplex AC-33) thickened with fumed silica produced a tough film around the sample that dried quickly. Both of these last two gels, when dry, kept the polyester resin from infiltrating.
Mixtures of 1, 3, and 10 wt% of Cab-O-Sil (coated fumed silica) in Rhoplex AC-33 produced semiclear films that dried in less than 2 hours. Approximately 3 wt% of Cab-O-Sil in the acrylic emulsion was optimum; however, since the mixture thickens with time, it is best to judge it by its consistency. The consistency should be very viscous, that is, greater than that of honey but less than that of butter. It should be gel-like but should flow enough to seal at the points where the solution meets itself. It is better to have the consistency slightly too thin than too thick. If the mixture is too viscous, it will be hard to get a uniform, thin layer around the sample, and it will not adhere to itself, resulting in coverage gaps.
Tests were done using both pure fumed silica and Cab-O-Sil. Both materials work well for thickening the acrylic dispersion, but they behave differently because pure fumed silica is hygroscopic while the Cab-O-Sil has been coated to make it nonhygroscopic. Thus, Cab-O-Sil is not quite as effective at thickening the solution, is harder to mix with the acrylic and takes longer to dry. The optimum mix for pure fumed silica with Rhoplex AC-33 acrylic is approximately 1 wt%.
A five-step procedure was used to encapsulate the samples (fig. 12). The first step was to prepare polymerized polyester resin in the bottom half of the mold. A small, thin, contiguous area of the acrylic/silica gel solution was painted at the tip of the mold, and the sample was positioned on it in the desired orientation (typically with the top paint layer down and horizontal to the bottom of the embedment). The area of the gel should be slightly larger than the size of the sample. Once the sample was in place, a small amount of the gel was painted thinly over the top and sides of the sample using a fine-point paintbrush or a toothpick. We prefer a toothpick since it is conveniently disposable. The sample should be completely encapsulated with the gel, since any open areas or cracks in the film would defeat the purpose of encapsulation. To ensure sufficient drying time, the encapsulation films were allowed to dry overnight. Attempts to hastened the drying process using a vacuum desiccator resulted in bubble formation in the films. Once the gel has dried to a film, the top layer of the polyester resin can be prepared as usual and poured over the sample. Upon curing, the embedded cross section can be polished or microtomed for analysis.
Fig. 12.
Steps used for encapsulating a cross section sample with a barrier material inside a polyester embedding resin
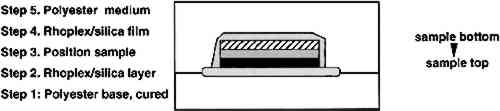 |
Two points should be kept in mind for success in using this method. First, the acrylic/silica encapsulation layer should not have any bubbles. To minimize bubbles during mixing, it is best to prepare only a small amount of the gel at a time. Second, the amount of acrylic/silica used to encapsulate should be kept at the very minimum needed to surround the sample completely, since thick (1mm) areas of the mixture can result in microtoming problems.
Figure 13 shows a photomicrograph of a paint cross section from a polychrome sculpture that was encapsulated in the acrylic/silica mixture and then embedded in polyester resin. The sample exhibits no visual signs of infiltration, and the glue-containing ground layer of the sample stained uniformly with FITC. Figure 14 shows a photomicrograph of the FITC-stained sample. The staining, though faint due to the low concentration of binder in the sample, does produce a uniform color for the glue ground layer portion of the sample. The variations in the intensity of the fluorescence on the bottom and middle layers of this sample are actually due to different concentrations of protein in the gesso sottile (bottom) layer and the gesso grosso (middle) layer of the sample (Souza and Derrick 1994). Figure 15 shows the infrared spectrum obtained from a thin section of the embedded sample. Infrared analysis confirmed that the ground layer of the sample did not experience infiltration of the polyester or of the acrylic media. Thus, the encapsulation of the sample was successful.
Fig. 13.
Photomicrograph of embedded paint cross section (Catas Altas sample CA039) that was barrier coated with Rhoplex thickened with Cab-O-Sil prior to embedding in polyester resin. the acrylic layer prevented the infiltration of the resin, as indicated by the opaque white appearance of the ground layer. 37�
 |
Fig. 14.
Photomicrograph of fluorescent image of FITC-stained sample shown in figure 13. The variations in the intensity of the fluorescence on the bottom and middle layers of this sample are due to different concentrations of protein in the gesso sottile (bottom) and gesso grossso (middle) layers of the sample. 37�
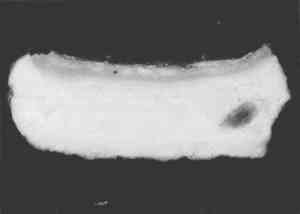 |
Fig. 15.
Infrared spectrum of paint cross section shown in figure 13. The spectrum shows that the embedding resin did not infiltrate the sample.
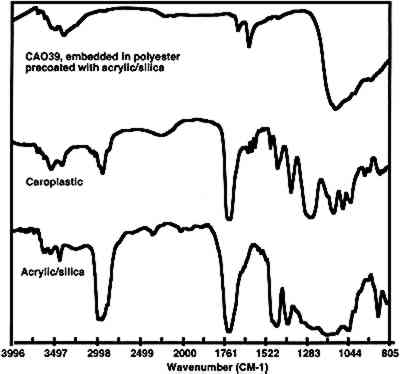 |
4 DISCUSSION
Preventing infiltration of the embedding resin into a sample will allow it to maintain its original physical and optical characteristics. This may or may not be desirable depending on the type of sample and the type of analyses to be done.
In the case of a porous, powdery sample the advantages of resin impregnation during embedding are that it will produce a solid, consolidated sample that may be highly polished to a flat, smooth surface. These qualities are useful for photomicrography, pigment identification, and SEM/EDS analysis. If needed, the sample should microtome well into intact thin sections. However, interpretation of any staining or infrared analysis would be difficult due to the presence of the resin within the sample. One viable option would be to perform infrared analysis on particles from a corresponding nonembedded portion of the sample.
Conversely, if this sample were not infiltrated with resin, the resultant embedment would contain a fragile and poorly cohesive sample. Some particles may fall out of the sample during polishing, and microtoming the sample will be difficult and time consuming. The advantage of an unadulterated sample is that any analysis method used for examining the sample is doing just that, examining the sample and not any added component. Resin infiltration may particularly affect the results of photomicrography, fluorescence, staining, colorimetry, or infrared microspectroscopy. The examination and detection of glazes and other thin, organic layers may be very susceptible to resin infiltration.
In our experience, the porous, crumbling sample is a rare, worst-case situation. Many cohesive samples that were not expected to be infiltrated were found to contain polyester resin after embedding. Thus, it is important to recognize that infiltration can occur and make a determination whether it is necessary to prevent the infiltration.
5 CONCLUSIONS
In a comparison of several types of polymers, polyester resins met the requirements for an ideal embedding media for most paint cross sections much better than did epoxies or acrylics. Polyester resins set rapidly without heat, are clear, polish readily, and microtome easily.
However, embedding resins, such as polyester, can dissolve some waxes, colorants, and resins. In addition, during the embedding process, porous, low-binder paints are penetrated by most types of polymer solutions, including polyester. Infiltration can occur with matte or porous paints that are often found in wall paintings, polychrome sculptures, ethnographic objects, and with glue gessos. This infiltration is visually detected by examining the cross section for poorly defined edges, darkening or discoloration, and blotchy stain results. Infiltration may be confirmed by the analysis of the sample with infrared microspectroscopy, where the presence of the embedding resin in the sample will produce a spectrum containing absorption bands characteristic of the resin.
It is important to recognize that infiltration can and has occurred in embedded paint cross sections. Infiltration has advantages and disadvantages, because it consolidates and supports the sample but can also hinder the analysis of the sample's components. Thus, potential infiltration should be considered when samples are examined, and a decision should be made whether it is necessary to prevent the infiltration based on the type of sample and the type of analyses.
In the cases where embedding without infiltration is chosen as the best option, several barrier methods have been tested for coating the sample with materials before embedding the sample in polyester resin. A method using Rhoplex AC-33 thickened with fumed silica worked best, forming a uniform coating around the sample that, when dry, did not allow the polyester resin to infiltrate into the sample. Many other types of high viscosity or thixotropic materials would probably work as well for precoating the sample.
6 EXPERIMENTAL
6.1 SAMPLES
Five types of samples were embedded in this study. The first type was a concentration series consisting of known mixtures of glue and gypsum to test the effects of binder concentration (figs. 5, 6). The second set of samples was obtained from a facsimile painting that had a glue-calcium carbonate ground layer with a glue content of 15 wt%. This set was used to evaluate the various kinds of embedding media shown in table 1. This set of samples along with samples of plaster (no binder) also were used to test all of the barrier methods shown in table 2. The fourth set of samples consisted of small portions obtained from a larger sample (CA042) that had fallen off a polychrome sculpture from the church of Catas Altas, Minas Gerais, Brazil (figs. 2, 3). Quantitative analysis of the protein content of the sculpture sample by gas chromatography showed that it contained 12% by weight of glue in gypsum (Schilling 1994). After the acrylic-silica barrier layer was found to work on the test samples, it was then tried on portions of another polychromy sample (CA039) from the church. This last set of samples, shown in figures 13 and 14, contain a gesso sottile layer (analyzed glue content of 5%) on the bottom and a gesso grosso layer in the middle (analyzed glue content of 10%).
6.2 MICROTOMING
An RMC Model 7000 microtome configured with a glass knife was used to produce 5 μm thin sections of the embedded samples. Tungsten knives may also be used to prepare thin sections in this range; stainless knives usually are used to produce thicker sections (20 μm) and diamond knives for thinner sections (1 μm). Glass knives are made by scoring and breaking a 1 in. square of glass to give 2 triangular pieces, each with a sharp edge. This procedure produces a 45� angle on the blade. This angle may be increased for cutting harder materials and decreased for softer materials (Malis and Steele 1990).
Small samples are easier to microtome. Larger samples may be cut into a pie shape and positioned in a mold so that the point is cut first. An embedded sample should have all excess medium trimmed from the embedment, leaving only a facet with a few mm of medium outside of the sample at the cutting surface. Too large a cutting surface can cause the section to curl and the sample to debond from the medium.
Orientation of the sample to the knife edge can affect the quality of the section. In this description, a multilayer sample is considered as a series of parallel lines that can be placed either vertically or horizontally in a rotatable vise of a microtome with a horizontal knife edge. For most samples, the initial cut is best made with the sample rotated 10� from vertical with the most important layer closest to the knife. Exact vertical orientation of the layers can increase section curling and particle loss, while direct horizontal orientation can result in the compression of the sample. Depending on the sample and how it behaves during cutting, the orientation may need to be changed. In all cases, the sample and the knife should be fastened securely and checked often for tightness.
The optimum thickness of a sample for infrared transmission analysis is 1–10 μm (Derrick et al. 1991). Sections over 15 μm can absorb IR radiation too strongly. Cutting thick sections can also result in particle loss, chattering of the knife, and damaged knife edges. Whenever a problem occurs, it can often be solved by slicing thinner sections (1 μm) and then returning to slice a thicker section (5–10 μm). All cuts should be smooth and slow, using a motorized microtome, if available, set at speeds of 0.1–3.0 mm/sec.
Vibrations, drafts, temperature, and humidity can all adversely affect microtoming results, as can variations due to lighting, hand temperature, and human breath. When problems occur, all factors should be considered and modified when possible.
As the section is being cut, it should be encouraged to cling to the knife. With a small, stiff artist's brush (size 2 or 3) the initial cut edge of the section can be held to the knife surface without touching the sample region. Static charges should keep the section on the knife. After the section is cut, it can also easily and delicately be picked up from the glass surface using the brush. For infrared analysis, our sections were taken directly from the microtome, placed on a BaF2 window, and transferred to the sample stage of the infrared microspectrophotometer.
6.3 INFRARED MICROSPECTROSCOPY
A Spectra-Tech IRμS organic microprobe was used for the infrared analysis of the thin sections of each sample. It is equipped with a narrow-band, cryogenically cooled mercury cadmium telluride (MCT) detector. The spectra are the sum of 200 scans collected from 4000–800 cm-1 at a resolution of 4 cm-1. The IRμS is continually purged with dry, CO2-free air.
Once microtomed, the thin section slices were placed on a BaF2 window and transferred to the sample stage of the infrared microspectrophotometer for analysis. An incandescent light is used to locate and focus on the sample. A small rectangular region of the sample, typically 30 � 60 μm, is isolated using dual adjustable knife-edge apertures located above and below the sample stage. The radiation source is then changed to an infrared beam for analysis of the imaged area.
6.4 FLUORESCENT STAINING
Fluorescein isothiocyanate (FITC) was used as the reagent for staining the proteinaceous media. Autofluorescence and other stains, such as amido black and rhodamine, may also be affected by infiltration of the resin. Our method used an aqueous solution; FITC in acetone also exhibited nonuniform intensities related to the resin infiltration.
FITC reacts with free amino end groups in the proteins to give a characteristic bright yellow fluorescence that is most visible in light color pigment areas. FITC and all "amine reagents" react best above pH 8 where the fraction of the free-base form of amines is higher (Haugland 1992). Following the procedure of Vera and Rivas (1988), an FITC solution was prepared by first dissolving FITC in acetone (5mg/ml) and then diluting it to 0.25% with a 0.1M phosphate buffer solution at pH 8.0 that additionally contains 0.1% nonionic detergent (Triton X-100) for better surface wetting. In comparison tests using glue, gelatin, whole egg, and casein, the aqueous FITC in phosphate buffer gave a much brighter yellow fluorescence color than a corresponding amount of FITC (0.25%) in acetone solvent. FITC is quite stable in water but will experience some degradation at increasing pH's. Therefore, the supplier, Molecular Probes, recommends pH 8.5–9.5 as optimum for reactions (Haugland 1992). We found that a pH 8.0 solution had good sensitivity (detection limit of 0.2% glue in a white pigment) and good stability.
Two photos were taken for each sample: (1) incandescent, incident light and (2) FITC-stained, fluorescent incident light using a mercury light source and a Leitz D filter cube. The Leitz D filter cube has a band pass excitation filter of 355–425 nm and a long pass barrier filter at 460 nm. For FITC staining, Leitz recommends a Leitz I2/3 filter cube (band pass filter: 450–490 nm, long pass filter: 515 nm) (Becker 1989). However, our studies showed that some proteins autofluoresced when we used a similar Leitz H filter cube, and that made it less effective for determining positive protein tests than the Leitz D filter cube. Also, the broader range of the Leitz D filter cube provides a brighter though less wavelength-specific fluorescent image.
For staining, a drop of the FITC solution was placed on the sample and allowed to set for 30–60 seconds. The excess solution was wicked off with a nonfluorescent, absorbent towel, since leaving the solution on the sample for longer periods, 5–15 minutes, may cause some of the proteins to swell.
REFERENCES
Baker, C. A.1984. Methylcellulose and sodium carboxymethylcellulose: An evaluation for use in paper conservation through accelerated aging. In Adhesives and consolidants. ed.N. S.Brommelle, et al. London: International Institute for the Conservation of Historic and Artistic Works. 55–59.
Baker, M., D.van derReyden, and N.Ravenel. 1989. FTIR analysis of coated papers. Book and Paper Group Annual8:1–12.
Becker, E.1989. Fluorescent microscopy. Germany: Wild Leitz GmbH.
Bischoff, J.1994. Personal communication. Detroit Institute of Arts, Detroit, Mich.
Byrne, G.S.1984. Adhesive formulations manipulated by the addition of fumed colloidal silica. In Adhesives and Consolidants. ed.N. S.Brommelle, et al. London: International Institute for the Conservation of Historic and Artistic Works. 78–80.
Cartwright, L. J., N. S.Cartwright, and P. G.Rodgers. 1977. A microtome technique for sectioning multilayer paint samples for microanalysis. Canadian Society of Forensic Science Journal10:7–12.
Demmler, K.1980. The determination of residual active oxygen in parts of unsaturated polyester resins and its influence on post curing and yellowing by light.Kunstoffe70:786–92.
Derrick, M. R., D. C.Stulik, and J. M.Landry. 1991. Scientific examination of works of art: Infrared microspectroscopy. Marina del Rey, Calif.: Getty Conservation Institute.
Derrick, M. R., D. C.Stulik, J. M.Landry, and S. P.Bouffard. 1992. Furniture finish layer identification by infrared linear mapping microspectroscopy. Journal of the American Institute for Conservation31(2):225–36.
Godla, J.1990. The use of wax finishes on pre-industrial American furniture. M.A. thesis, Antioch University, West Townsend, Mass.
Hansen, E. F., R. Lowinger, and E.Sadoff. 1993. Consolidation of porous paint in a vapor-saturated atmosphere: A technique for minimizing changes in the appearance of powdering, matte paint. Journal of the American Institute for Conservation32:1–14.
Haugland, R. P.1992. Handbook of fluorescent probes and research chemicals. 5th ed.Eugene, Oreg.: Molecular Probes.
Horie, C. V.1987. Material for conservation. London: Butterworths. 161–65.
KeyserB.1981. Use of acrylic emulsions as non-penetrating lining materials for paintings at the National Gallery of Canada. International Institute for Conservation-Canadian Group6(3):9–10.
Malis, T. F., and D.Steele. 1990. Ultramicrotomy for materials science. Workshop on specimen preparation for TEM materials 2, ed.R.Anderson. Materials Research Society Symposium Proceedings 199. Pittsburgh: Materials Research Society. 3–43.
Mehra, V. R.1984. Dispersion as lining adhesive and its scope. In Adhesives and consolidants. ed.N. S.Brommelle, et al. London: International Institute for the Conservation of Historic and Artistic Works. 44–45.
Meurgues, G.1982. Synthetic resins can be dangerous. Museum34:60–61.
Plesters, J.1956. Cross-sections and chemical analysis of paint samples. Studies in Conservation2:110–57.
Purves, P. E., and R.S.J.Martin. 1950. Some developments in the use of plastics in museum technology. Museums Journal49:184–95.
Reedy, C.1994. Thin section petrography in studies of cultural materials. Journal of the American Institute for Conservation33:115–29.
Schilling, M.1994. A fast, sensitive, reproducible method for the analysis of amino acids using ethyl formate derivitization. Getty Conservation Institute internal report.
Souza, L.A.C., and M. R.Derrick. 1994. The use of FT-IR spectrometry for the identification and characterization of gesso-glue grounds in wooden polychromed sculptures and panel paintings. In Materials issues in art and archaeology 4, Materials Research Society Symposium Proceedings. Pittsburgh: Materials Research Society.
Tsang, J., and R.Cunningham. 1991. Some improvements in the study of cross sections. Journal of the American Institute for Conservation30:163–77.
Vera, J. C., and C.Rivas. 1988. Fluorescent labeling of nitrocellulose-bound proteins at the nanogram level without changes in immunoreactivity. Analytical Biochemistry173:399–404.
Waentig, F.1993. Casting resin systems for embedding specimens. Restauro3:195–99.
Ward's Natural Science. 1990. Ward's curriculum aid: Embedding in bio-plastic, 6th ed. No. 246-0005. Rochester: Ward's Natural Science.
Wilkinson, J. M., J.Locke and D. K.Laing. 1988. The examination of paints as thin sections using visible microspectrophotometry and Fourier transform infrared microscopy. Forensic Science International38:43–52.
Wolbers, R. and G.Landrey. 1987. The use of direct reactive fluorescent dyes for the characterization of binding media in cross sectional examinations. AIC preprints, American Institute for Conservation. 15th Annual Meeting, Vancouver. Washington D.C.: AIC. 168–202.
Wolbers, R.1993. Personal communication. Winterthur, Del.
SUPPLIERSMaterials tested in this study were obtained from the suppliers listed below. These products are also available from other suppliers that specialize in general laboratory and microscopy products.Butylmethyl methacrylate (acrylic)Ladd Research Industries, Inc., Burlington, Vt., 05402; (802) 658–4961 Bio-Plastic (polyester)Ward's Natural Science, P.O. Box 92912, Rochester, N.Y.; (800) 962–2660 Cab-O-Sil (coated fumed silica)Conservation Materials Ltd., 1165 Marietta Way, P.O. Box 2884, Sparks, Nev. 89431;, (702) 331–0582 Caroplastic (polyester)Carolina Biological Supply Company, 2700 York Road, Burlington, N.C. 27215; (800) 334–5551, Buehler, 41 Waukegan Road, Lake Bluff, Ill., 60044; (800) 283–4537 Castolite (polyester)The Castolite Company, Woodstock, Ill. 60098;, (815) 338–4670 Epon 812 (epoxy)Ted Pella, Inc., P.O. Box 2318, Redding, Calif., 96099; (916) 243–2200 Fluorescein isothiocyanate (FITC)Molecular Probes, Inc., 4849 Pitchford Ave., Eugene, Oreg. 97402; (503) 465–8300. Krazy Glue and Krazy Glue Gel (cyanoacrylate)Distributed by Borden, Inc., HPPG, Columbus, Ohio 43215 LR White (acrylic)Ladd Research Industries, Inc. LX-112 (epoxy)Ladd Research Industries, Inc. Maraglas 655 (acrylic)Ladd Research Industries, Inc. Paraplast (wax)Ladd Research Industries, Inc. Pelco, embedding moldsTed Pella, Inc. Rhoplex AC-33 (acrylic emulsion)Rohm and Haas Co., Philadelphia, Pa., Distributed by Conservation Materials, Ltd. Silica, fumedAldrich, 1001 W. Saint Paul Ave., Milwaukee, Wisc. 53233; (800) 558–9160 Quetol 523M (acrylic)Ted Pella, Inc. SPURR (epoxy)Ted Pella, Inc. Vestopal W (polyester)Ladd Research Industries, Inc.
AUTHOR INFORMATION
MICHELE R. DERRICK graduated from Oklahoma State University in 1979 with an M.S. in analytical chemistry. In 1983 she joined the scientific program of the Getty Conservation Institute, where she is currently an associate scientist. Her research involves the development of new methods for the characterization and identification of organic materials in cultural objects primarily using infrared spectroscopy and pyrolysis gas chromatography. Address: Getty Conservation Institute, 4503 Glencoe Ave., Marina del Rey, Calif. 90292.
LUIZ A. C. SOUZA received his B.S. (1986) and M.Sc. (1991) in chemistry from the Federal University of Minas Gerais. The experimental work for his M.Sc. was done at the Institut Royal du Patrimoine Artistique, Brussels (1987–88). Since 1989 he has been teaching and researching at the CECOR Center for Conservation and Restoriation of Movable Cultural Properties of the Federal University of Minas Gerais, Brazil. He is currently a research fellow at the Getty Conservation Institute. Address: CECOR, Escola de Belas Artes, Universidade Federal de Minas Gerais, Av. Antonio Carlos, 6627, Belo Horizonte 31270, MG, Brazil.
TANYA L. KIESLICH received her B.S. from Loyola Marymount University in 1992 with a major in chemistry and a minor in art history. As part of her undergraduate research, she used infrared microspectroscopy for the analysis of paint samples. She is currently attending the Courtauld Institute of Art for a postgraduate diploma in the conservation of easel paintings. Address: Courtauld Institute of Art, Dept. of Conservation and Technology, Somerset House, Strand, London WC2R ORN, England.
DUSAN C. STULIK graduated from Charles University in Prague, Czechoslovakia, with B.S. and M.S. degrees in chemistry. He subsequently obtained a Ph.D. in physics from the Czechoslovakia Academy of Sciences. He is currently acting head of the scientific program at the Getty Conservation Institute. His current research is in the application of modern scientific methods in conservation science. Address: same as for Derrick.
Section Index |