THIN-SECTION PETROGRAPHY IN STUDIES OF CULTURAL MATERIALS
CHANDRA L. REEDY
ABSTRACT—Thin-section petrography can serve a number of functions in studies of cultural objects. It is used to characterize and identify inorganic materials, locate the specific source of materials, group objects that may have a common source, and, less commonly, provide information on object deterioration and the effects of conservation treatment. This paper presents an introduction to the types of cultural materials and research questions that have been examined through thin-section petrography. Examples involve studies of stone (sculptures and architectural materials), ceramics (vessels and sherds, terracotta sculptures, and architectural ceramics), glass and glazes, and miscellaneous materials such as clay core materials from bronzes, plaster and cements, frescoes, and slags.
1 INTRODUCTION
Thin-section petrography is polarized light microscopy of rocks and other mineral-containing materials, using samples ground to a standard thickness of 30 μm. The standard thickness gives known colors between crossed polarizers, facilitating comparison of different samples and the use of reference tables of mineral optics for identifying unknowns. Use of a specific, known thickness for all standard petrographic samples differs from the cross sections often used in conservation.
Geologists use thin-section petrography to describe and classify rocks, soils, and sand. Archaeologists and conservation scientists use it to study many inorganic materials used in the production of cultural objects. Purposes of such analyses in cultural object studies include making correct material identifications, grouping similar objects, comparing materials-based groupings to art historical groupings, identifying the geological origin of the object or some of its components, and studying manufacturing technology. For some art materials, structural and mineralogical changes on weathered surfaces in comparison to unaltered interior sections of a sample may provide information concerning the authenticity of a piece. Thin sections have also been used to study the deterioration of inorganic art and architectural materials and to check the effects of conservation treatments on those materials.
One advantage of thin-section petrography is that the necessary equipment (a polarizing microscope) is relatively inexpensive, making the technique potentially available to almost any laboratory as a routine method of analysis. Although a significant investment in training and experience is required to use this technique to full advantage, thin-section analysis is still a very efficient way to obtain crucial information about many inorganic materials. The cost of purchasing and maintaining a polarizing microscope is much less than for other types of equipment used to study inorganic objects (such as a scanning electron microscope, electron beam microprobe, x-ray diffractometer, or elemental analysis instrumentation). All these alternatives, including thin-section petrography, require extensive training and experience in the materials being analyzed and in the theory underlying the instrument.
Although thin-section petrography was developed many decades ago, conservation science still does not utilize it to its full potential. Thin sections are often the most useful starting point for a study of inorganic materials, even if they may sometimes need to be supplemented by other approaches. They provide information not available by other analytical techniques.
To make a thin section, the sample material is mounted on a glass slide using an epoxy resin with a refractive index (1.54–1.55) essentially the same as that of quartz, a ubiquitous mineral found in almost all geological materils. Thin sections are then ground to a uniform thickness of 30 μm. Very porous or friable materials may be impregnated, and techniques for mounting loose grains have also been developed. A thin section may be protected by a coverslip, particularly important for friable specimens and other materials that might easily become damaged. However, if thin sections are left uncovered they can also be used for a variety of additional analytical techniques such as microhardness and microchemical tests, scanning electron microscopy with elemental analysis capabilities, or microprobe analysis (Hutchison 1974; Loretto 1984; Goodhew 1988; Reed 1993). Staining of uncovered sections can aid the identification of minerals that are otherwise difficult to differentiate in thin section. Examples of the use of stains include distinguishing between alkali and plagioclase feldspars and between calcite and dolomite (Allman and Lawrence 1972; Hutchison 1974).
When examined under a polarizing microscope, minerals in mounted thin sections of 30 μm can be identified through a variety of optical properties, usually at magnifications ranging from 16� to 400�. For transmitted plane polarized light, the properties include transparency versus opaqueness, color, pleochroism, refractive index, relief, morphology, and cleavage. Between crossed polarizers, important properties include isotropism versus anisotropism, birefringence, extinction angle, and the presence or absence of other features such as zoning, twinning, undulous extinction, and anomalous polarization colors (Phillips 1971; Kerr 1977; MacKenzie and Guilford 1981; MacKenzie et al. 1982; Williams et al. 1982; Adams et al. 1984; Yardley 1990).
In addition to the identification of specific minerals, thin-section petrography also involves the study of mineral and rock textures, coarseness, and the relative or quantitative percentage of various constitutents. The data collected from thin-section studies can easily be used for statistical analysis by using quantitative measurements, converting binary and categorical information to arithmetic data, or using statistical methods designed for binary and categorical data (Chayes 1956; Keith and Cooper 1974; Stoltman 1989; Agresti 1990; Matthew et al. 1991; Reedy 1991; Reedy and Reedy 1994). One can also decrease the subjective aspects of thin-section analysis by coding sections for “blind” analysis, randomizing the order of analysis, or using semiautomatic image analyzer systems (Middleton et al. 1985; Garrett 1986; Stoltman 1989; Middleton et al. 1991).
To introduce the potential of thin-section petrography for studies of cultural materials, this paper includes brief discussions of a wide variety of relevant materials and research problems. These examples are divided into sections on stone, ceramics, glass and glazes, and other miscellaneous materials.
2 STONE
2.1 STONE SCULPTURES
Thin-section studies of stone sculptures frequently aim to identify the stone material or find its source. Some of the sculptural materials that have been characterized are sandstone, limestone, marble, phyllite, schist, quartzite, granite, basalt, andesite, tuff, steatite, soapstone, gypsum, and others (Kempe and Harvey 1983; Newman 1984).
A notable example is work on ancient Egyptian limestone sculptures (Harrell 1992). Many ancient quarries have been located in the Nile Valley, and samples from them have been characterized by thin-section analysis. Because of this work it is possible to identify the provenance of many sculptures and architectural stones. Limestones from different sources can differ greatly in grain size and texture and in the types of microfossils present, as illustrated in figures 1 and 2. They may also differ in type and ratio of carbonates present (generally calcite and/or dolomite). Other techniques such as elemental analysis have also proven quite useful in limestone provenance work (for example, Meyers and van Zelst 1977; Holmes et al. 1986). Still, thin-section analysis can often answer the question of provenance as long as the potential sources differ in one or more of the characteristics mentioned above.
Fig. 1.
A large (2 mm) bioclast, or skeletal particle from a carbonate-secreting organism, in microcrystalline limestone with some scattered accessory minerals. Plane polarized light, 32x
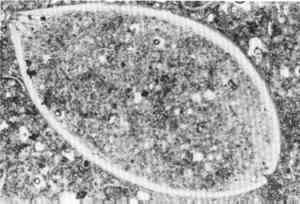 |
Fig. 2.
Limestone with spherical particles called ooids. The coarse-grained matrix has a number of rhombohedral calcite grains. Plane polarized light, 32x
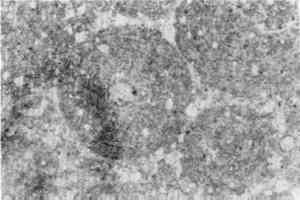 |
Marble sculptures, particularly from the Aegean region, have received much attention from thin-section petrographers. A systematic description (now outdated) of the petrography of classical marble quarries was published more than a century ago (Lepsius 1890). That work described features such as grain size, extent and orientation of metamorphic foliation, color, and presence of trace minerals. Later work expanded knowledge of the petrographic characteristics of specific quarries of the classical world (for example, Washington 1898; Marinos 1948; Weiss 1954; Herz 1955). These studies added the description of features such as preferred orientation of calcite grains, form of grain boundaries, and the degree and manner of recrystallization. Weiss (1954) and Herz (1955) also used identification of the preferred orientation of calcite to match fragments that had once been part of a single sculpture or inscribed slab. Renfrew and Peacey (1968) reviewed inaccuracies and inadequacies in Lepsius's work (1890). Some of these were due to incomplete petrographic descriptions, the use of subjective methods of analysis that did not include blinding or randomization, and sample sizes too small to characterize adequately the variation found in marble quarries.
As the number of sampled quarries has increased, it is now recognized that no one method of analysis alone is sufficient for provenance studies of classical marbles, except when the possible source locations and comparisons are very limited. Inherent overlaps found when many potential sources are compared inhibit the effectiveness of petrographic, elemental, or isotopic data (Kempe and Harvey 1983). Therefore thin-section analysis is most useful for this material when used in combination with other methods, particularly if statistical analysis includes two or more types of data (for example, Lazzarini et al. 1980; Germann et al. 1988; Moens et al. 1988; Roos et al. 1988; Moens et al. 1989; Lazzarini 1990;Moens et al. 1990). Petrographic features found most useful in those studies include the shape of calcite crystals, average and maximum calcite grain size, and to a limited extent the presence of accessory minerals. The thin sections can also be stained with Alizarin Red S in dilute hydrochloric acid to distinguish between calcite and dolomite. Since calcite is readily soluble in the acid and dolomite is not, only the calcite stains (Hutchison 1974).
Thin-section analysis has proven very useful in provenance studies of Gandharan schist sculptures from ancient Afghanistan and Pakistan (Courtois 1962–63; Lahanier 1976; Kempe 1982; Newman 1984; Kempe 1986; Newman 1992; Reedy 1992b). These Buddhist sculptures from northwest Pakistan show a clear mix of influences from both Greece and India. They are most often made of a shiny gray schistose material that has been identified in thin section as a carbonaceous phyllite or schist primarily consisting of quartz and muscovite, with chlorite sometimes present. An unusual feature is the prominent inclusions of tiny rods of chloritoid (fig. 3). Comparison with samples from possible quarry sites indicates that the sources were located within the Swat and Peshawar Valleys. Newman's (1992) microprobe analysis of the chloritoid inclusions has enabled further grouping of sculptures that probably derive from the same source.
Fig. 3.
Rod-shaped chloritoid inclusions are characteristic of carbonaceous phyllites and schists from northwest Pakistan used to produce many Gandharan Buddhist sculptures. Plane polarized light, 80x
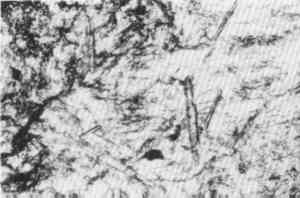 |
Thin sections of stone sculptures have also been used in authenticity studies, as one way of examining the natural patina or weathering layers that may have formed since the surface was worked by the sculptor (fig. 4). The mineralogical and textural changes of the stone depend upon the type of stone (such as marble, tuff, schist, etc.) and its environmental conditions (such as burial in the soil or long-term outdoor exposure). For any combination of stone type and environmental condition we need a set of reference specimens that includes a variety of objects of known authenticity, and preferably some known to be forgeries. Newman (1990), building on earlier work on weathering layers (Hipkiss et al. 1937; Young and Ashmole 1968), discussed characteristics of marble weathering layers. he prepares thin sections without cover slips so they can also be used in electron beam instruments. Such background studies are not yet available for most classes of stone sculpture; the effects of common conservation treatments on the overall surface and on weathering layers have also received little research.
Fig. 4.
A natural weathering layer found on the outer surface of volcanic tuff. Plane polarized light, 32x
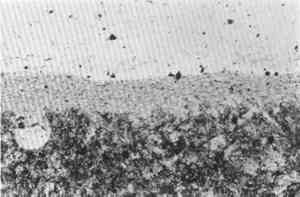 |
One of the earliest and most successful petrographic thin-section studies of artifacts was of ceramic materials excavated at the site of ancient Troy (Felts 1942). This study remains a model of the potential utility of thin-section analysis of ceramics and sherds. Three research questions were addressed in this study: (1) Among the ceramics excavated at the site, is it possible to distinguish local from imported wares? (2) Do ceramics produced in different time periods differ in thin section? (3) Can ceramic manufacturing methods be deduced from a study of ceramic structures and how they have been altered by firing conditions?
Felts compared thin sections made from the ceramics and from soil samples taken in and around Troy. Most ceramic samples were found to have rock and mineral inclusions native to the local region and seen in the local soils (such as quartzite, a green hornblende trachyte porphyry, siltstone, and a strongly foliated mica schist). In a small percentage of the objects the inclusions consisted of minerals and rock fragments not seen locally (such as basaltic lava). Therefore, these objects were hypothesized to be imported. The imported wares also seemed to differ from local ones in shaping and firing techniques. Shaping techniques identified through thin sections include the use of a potter's wheel, which is indicated by internal alignment of pores and platy and rodlike mineral fragments (fig. 5). The degree of firing was estimated qualitatively by observing whether the clay matrix had been partially vitrified, whether calcite had been thermally dissociated, and whether there were thermal changes apparent in minerals such as quartz.
Fig. 5.
Internal structure alignment in a ceramic thin section indicating that the vessel was shaped on a potter's wheel. Plane polarized light 32x
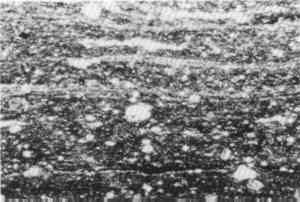 |
Pottery and soils from various archaeological strata were examined for temporal changes. The earliest sherds were very porous (up to 20% of a typical thin section consisted of spherical and elliptical vesicles). Through time there was a clear change in technology toward smaller grain size for tempering materials, less porosity, and an increasing percentage of objects produced on a wheel. Only a few mineralogical differences were found among the different strata.
The thin-section study successfully identified a variety of manufacturing approaches. The earliest clays showed fine, stream-rounded natural inclusions of quartz, feldspars, micas, and calcium carbonate minerals, indicating that detrital silts were collected with little or no purification. The purity of the clay increased over time and was accompanied by an increase in the use of added tempers. A lack of internal preferred orientation of grains and the haphazardly distributed porosity showed that a minimum of working was done during the shaping process. Firing occurred in a reducing atmosphere, producing gray pottery with reduced iron oxides and small amounts of carbon from organic matter. However, frequent patches of oxidized materials showed that the firing conditions were not well controlled. Very few sherds reached temperatures high enough to cause calcite to disintegrate.
Over the years a large number of successful provenance studies of ceramic vessels have used thin-section petrography (Peacock 1970; Williams 1983). These studies usually involve analyzing the larger-grained tempering material and comparing it to potential geological sources (for exact pinpointing of manufacturing sources) or to temper material from other ceramic samples (for relating objects that might have a common source). These studies have involved ceramic traditions from a wide range of regions such as the American Southwest (Shepard 1942), Greece (Farnsworth 1964) and the West Indies (Donahue et al. 1990).
One particularly interesting example is work carried out on sand-tempered Pacific Island ceramics. This work helped to reconstruct the history of the settling of the islands by providing evidence for the movement of people from one island or island group to another (Dickinson and Shutler 1974; Dickinson and Shutler 1979; Dickinson et al. 1990). Island potters were relatively restricted in their choice of where to obtain sand tempers, generally to those available within their island or local island cluster. After petrographically characterizing the sands of 50 islands and 15 major island groups, the researchers were easily able to identify local versus imported ceramics. When objects found on one island were clearly made elsewhere, the patterns of past human movement could be retraced. Since the differently tempered ceramics did not differ significantly in visual appearance or quality and materials for manufacturing ceramics are readily available on all the islands, the authors felt that the addition of foreign-tempered examples was more likely due to movement of people than to a ceramics trade. In addition to the presence, size, and shape of diagnostic minerals, inclusions within mineral grains were very important. For example, glass inclusions within plagioclase and pyroxene grains indicated that they derived from volcanic source rocks.
Some ceramic petrographers are more interested in understanding the wider context of ceramic technology than in provenance (Hodges 1963; Freestone et al. 1982; Middleton and Freestone 1991). Although they draw on methods of sedimentary petrography and soil micromorphology, they note that since ceramics are made by humans, the methods of geologists are not sufficient for understanding ceramic fabric variations caused by human interactions with raw materials. Thus ceramic petrographers are working to develop their own methods to describe ceramic fabrics in such a way as to highlight both the nature of the raw materials and the effects of ceramic technology (Whitbread 1989).
2.3 TERRACOTTA SCULPTURES
Since it is a coarse ceramic material, terracotta should theoretically be suitable for provenance studies by thin-section petrography. It tends to have high amounts of heavy minerals (such as olivine, zircon, hornblende, hypersthene, garnet, tourmaline, rutile, epidote, and chloritoid) known to be extremely sensitive indicators of provenance (Morton 1985). However, no major studies focusing on petrography of terracotta sculptures have as yet been published.
In a pilot study of three Gandharan terracotta sculptures, major differences were found in the coarse-grained fractions (Reedy 1992a). A hooded female figure contained a fine-grained quartz in a hematite-rich clay, with accessory amounts of muscovite, gypsum, anhydrite, carbonates, clinopyroxene, and iron oxides (fig. 6). A female bust also contained primarily fine-grained quartz along with the clay and accessories of plagioclase feldspar, muscovite, chloritoid, and hypersthene. However, a bearded head was formed with a material that looked quite different from the other two in thin section. The quartz fraction was coarser, but the most striking difference was that the amphibole mineral hornblende was present as a major constituent (fig. 7). Thirteen different accessory minerals and lithic fragments were also present. The variation seen here indicates that thin-section petrography of terracotta sculptures might very well be useful in distinguishing between periods and places of production as well as in studying the materials and technology employed by craftspeople.
Fig. 6.
Material from a Gandharan terracotta sculpture. The dark chunks are a hematite-rich clay and the light ones are the fine-grained sand component (mainly quartz). Plane polarized light, 32x
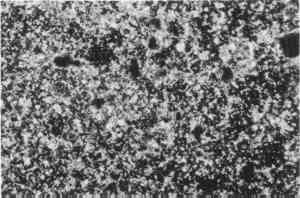 |
Fig. 7.
Material from a different Gandharan terracotta sculpture that is much more coarse-grained than that in figure 6. Most of the chunky dark-colored grains are hornblende, an amphibole mineral that is a prominent constituent of many detrital sediments. Plane polarized light, 32x
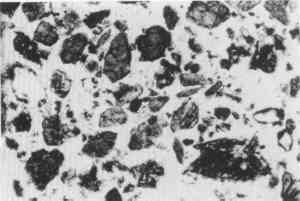 |
2.4 ARCHITECTURAL CERAMICS
Architectural ceramics such as bricks and terracotta tiles are amenable to thin-section studies for identification of components, provenance studies, assessment of deterioration, or monitoring of conservation treatments. In one example, thin sections of bricks, roof tiles, and floor tiles from York, England, were studied from a variety of medieval and postmedieval structures. The results showed that although the majority of York's ceramic building materials were of local manufacture, some were imported to the area during various periods (Betts 1991). Among the materials manufactured locally, some functional and chronological differences became apparent through analysis of grain size distribution. (Grain size distribution for a thin section is an estimate of the fraction of grains in each of several size classes.)
3 GLASS AND GLAZES
3.1 GLASS
Glass, as a noncrystalline material, does not normally reveal any useful information in thin section. However, optical microscopy has at times been a useful adjunct to x-ray diffraction and electron microscopy for studying devitrification processes in glass, the development of cracks and crack profiles, and inclusions that reflect the production history, homogeneity, and stability of glass (Porai-Koshits 1973; Simmons et al. 1982). For example, some of the mineral inclusions found in 19th-century glass include wollastonite, diopside, cordierite, and quartz (Barger et al. 1989).
3.2 GLAZES
The study in thin section of glazes on ceramics, as in the case of glass, can be used to identify crystalline inclusions. In addition, a thin section can help to determine if any interactions have occurred between the ceramic body and the glaze. As one example, thin sections, in conjunction with other methods of analysis, have been used to study Korean celadons (Newman 1991; Koh Choo 1992). These objects consist of stoneware with a pale green or greenish blue glaze that resembles jade in color and texture. They were produced in Korea from about the late 9th century into the 15th century. The earliest works were modeled on Chinese celadons, but distinctively Korean forms and techniques evolved. A variety of factors contribute to the visual appearance of the glaze. These include the presence in the glaze of bubbles, undissolved raw materials such as quartz grains, and minerals such as anorthite and wollastonite that form during firing of the glaze. Interactions between the glaze and the ceramic body can also contribute to the visual effect, when the body absorbs some of the light transmitted by the glaze and decreases its brightness.
4 MISCELLANEOUS MATERIALS
4.1 CLAY CORE MATERIALS FROM BRONZES
Hollow-cast metal sculptures frequently have an inner clay core material. This clay is often only sun-dried rather than baked or fired, so it is not strictly classified as a ceramic material. The strength of a fired ceramic is not required for this application. Furthermore, fine clays are not necessary since plasticity is not important. A major sand component is advantageous since it helps to prevent gas bubbles during casting by facilitating gas release. Material collected at local stream beds is usually sufficient, so this type of material can often be useful for grouping objects according to place of manufacture as long as the variation within a single region is less than the variation between regions. Results have been successful for studies of South Asian sculptures and Renaissance bronzes (Milam et al. 1988; Reedy 1991)(fig. 8).
Fig. 8.
A sandy clay core from a Renaissance bronze. The light-colored grains are primarily quartz; the dark matrix is primarily clay. Crossed polarized light, 32x
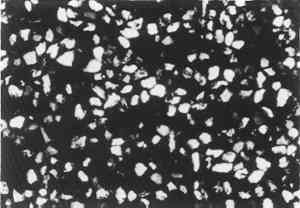 |
Because of the sandy nature of most clay core materials, some of the most useful petrographic work has come from in-depth studies of variation in the quartz grains. Since quartz is by far the most abundant mineral in sediments, it has received much attention in geology as a source indicator, and many features such as texture, size, shape, and inclusions have been identified as important for provenance work (Scholle 1979; Folk 1980)(figs. 9 and 10). For example, lattice dislocation in quartz can produce grains made up of a number of subcrystals with different crystallographic orientations, called polycrystalline quartz. Such lattice dislocation may be more common in metamorphic rocks, so this quartz feature may indicate sediments with metamorphic source rocks, especially if the boundaries between subcrystals are sutured rather than straight (Adams et al. 1984; Basu 1985). In addition to quartz studies, noting the presence or absence of numerous accessory minerals is an important part of thin-section studies of clay core materials.
Fig. 9.
Polycrystalline quartz, in which more than three subcrystals are present within a single grain. Different subcrystals go extinct at different degrees of rotation of the microscope state, producing a patchy effect. Crossed polarized light, 32x
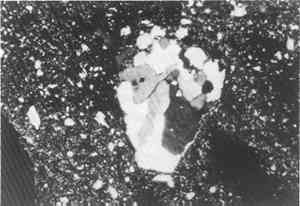 |
Fig. 10.
Polygonized quartz is differentiated from polycrystalline quartz by being limited to two or three subcrystals present within a single grain. Crossed polarized light, 80x
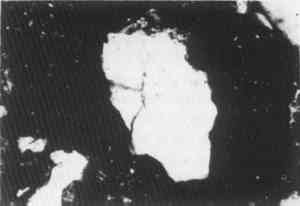 |
4.2 PLASTERS AND CEMENTS
Plaster may contain burnt lime, gypsum, clay, sand, water, and organic materials. It is used as a coating for architectural surfaces such as walls and floors and may also be used to produce objects such as beads, figurines, or vessels. Thin-section petrography is used to identify the various components of a plaster and their respective proportions. It can also give information such as grain size, mineral impurities present in the sand or lime, textural characteristics of minerals, and degree to which components such as shell were crushed during processing. Barnett (1991) has pointed out that optical mineralogy is generally the easiest and least-expensive method of distinguishing among gypsum, lime, and clay in plasters. These three components vary greatly in birefringence, indices of refraction, and morphology.
Goren and Goldberg (1991) found that since thin sections provide information on grain textures and interrelationships, they were more useful than other techniques (such as x-ray diffraction, scanning electron microscopy, and elemental analysis) for reconstructing Neolithic lime plaster production technology in northern Israel. Thin-section analysis was also the primary method of a major study that characterized the calcareous cements used in pre-Hispanic Mesoamerican building construction (Hyman 1970). Besides just identifying the major components, Hyman was able to discern the types of microfossils present in the lime and the grain size and degree of rounding. With this information he could deduce the environment in which the limestone must have formed and thus narrowed the possible locales where it could have been collected. Klemm and Klemm (1991) used thin sections to characterize mortar samples from the pyramid districts of the Old Kingdom in Egypt. Contrary to earlier assertions, they determined that both gypsum and lime mortars were used.
Although plaster of paris is a very fine-grained, highly processed material, it, too, is amenable to thin-section studies. Variation in this gypsum-based material is found in features such as particle size; the presence of mineral contaminants or additions such as quartz, carbonates, and clay; and the percentage of various anhydrite and gypsum phases (natural or high-temperature anhydrite; dihydrate, alpha hemihydrate, or beta hemihydrate phases of gypsum) (Kuntze 1984). Since gypsum dissolves relatively easily in water, thin-section preparation of gypsum-containing materials must use an oil-based preparation method.
4.3 FRESCOES
Petrographic analysis has potential usefulness in the study of conservation treatments for frescoes. One common problem is the consolidation of surfaces that are crumbling and friable due to excess humidity. Vagts (1993) included thin-section analysis as part of her research into the potential of colloidal silica in a sol-gel form (Ludox-SM colloidal silica dispersed in water) for consolidation of friable surfaces of frescoes. Thin sections of consolidated and unconsolidated samples were compared to examine the effect of the consolidation as well as the depth of penetration. The unconsolidated sample had a much more fractured appearance than the consolidated one. In addition, it was possible to see that the silica dispersion had penetrated approximately 3 mm into the surface, although the penetration was very uneven across the surface. One advantage of a standard thin section is that the mineralogy and microtexture of the fresco can easily be characterized as part of the study—important considerations for any study of deterioration and treatment of a material. Another is that the structure and grain relationships can be monitored before and after treatment and any other potentially adverse changes noted. With the relatively large area of a standard thin section, one can also easily observe the degree of consistency in depth of penetration of the consolidant.
4.4 SLAGS
Slags are waste products from the reduction of metals from ores. They are frequently present in archaeological collections and at historic sites. Most common in such collections are slags from copper smelting and iron smelting and smithing. Tin and lead slags are also sometimes encountered. The value of slag is not always recognized by curators, and sometimes irreplaceable collections have been destroyed to make space for objects more readily recognizable as cultural artifacts (MacMahon 1988). However, slags are an extremely important material for reconstructing past metallurgical processes. Thin-section petrography is generally done along with other techniques such as x-ray diffraction and elemental analysis to identify the composition of slag and its various components (Bachmann 1982).
A wide variety of minerals might be visible in slag thin sections, including many silicates, oxides, and sulfides. These minerals provide information about the composition of ores and fluxes that were used. The amount of metallic inclusions in slag indicates the efficiency of smelting operations, since the goal was to remove as much of the metal as possible. Furthermore, because the temperature of formation of minerals is well understood, the identification of mineralogical content helps in the reconstruction of temperature ranges achieved during smelting.
5 SUMMARY
The primary applications of thin-section petrography to studies of cultural objects have been for simple characterization of materials and for provenance studies or technological reconstructions. Despite pioneering studies in the early 1940s that showed the enormous potential of thin-section petrography for studies of cultural materials, there has been rather limited application of this approach, leading petrography to be called “one of the most under-utilized analytical techniques of proven value” (Stoltman 1989, 147). The areas where this is most true have been studies of deterioration and the effects of conservation treatments. There is certainly room for greater development of this technique as a tool for the analysis of cultural materials.
REFERENCES
Adams, A. E., W. S.MacKenzie, and C.Guilford. 1984. Atlas of sedimentary rocks under the microscope. New York: John Wiley.
Agresti, A.1990. Categorical data analysis. New York: John Wiley.
Allman, M., and D. F.Lawrence. 1972. Geological laboratory techniques. New York: Arco.
Bachmann, H-G.1982. The identification of slags from archaeological sites. London: Institute of Archaeology.
Barger, M. S., D. K.Smith, and W. B.White. 1989. Characterization of corrosion products on old protective glass, especially daguerreotype cover glasses. Journal of Materials Science24:1343–56.
Barnett, W. K.1991. Optical petrography as a tool for examining gypsum and lime plaster pyrotechnology. Journal of Field Archaeology18:253–55.
Basu, A.1985. Reading provenance from detrital quartz. In Provenance of arenites, ed. G. G.Zuffa. Boston: D. Reidel Publishing. 231–47.
Betts, I.1991. Thin-section and neutron activation analysis of brick and tile from York and surrounding sites. In Recent developments in ceramic petrology, ed. A.Middleton and I.Freestone. London: British Museum Research Laboratory. 39–55.
Chayes, F.1956. Petrographic modal analysis. New York: John Wiley.
Courtois, L.1962–63. Examen min�ralogique de quelques roches de monuments Gr�co-Bouddhiques. Arts Asiatiques9:107–13.
Dickinson, W. R., and R.ShutlerJr.1974. Probable Fijian origin of quartzose temper sands in prehistoric pottery from Tonga and the Marquesas. Science185:454–57.
Dickinson, W. R., and R.ShutlerJr.1979. Petrography of sand tempers in Pacific Islands potsherds: Summary. Geological Society of America Bulletin90(1):993–95.
Dickinson, W. R., J.Takayama, E. A.Snow, and R.ShutlerJr.1990. Sand temper of probable Fijian origin in prehistoric potsherds from Tuvalu. Antiquity64:307–12.
Donahue, J., D. R.Watters, and S.Millspaugh. 1990. Thin section petrography of Northern Lesser Antilles ceramics. Geoarchaeology5(3):229–54.
Farnsworth, M.1964. Greek pottery: A mineralogical study. American Journal of Archaeology68:221–28.
Felts, W. M.1942. A petrographic examination of potsherds from ancient Troy. American Journal of Archaeology46:237–44.
Folk, R. L.1980. Petrology of sedimentary rocks. Austin: Texas Hemphill's Book Store.
Freestone, I., C.Johns, and T.Potter. 1982. Current research in ceramics: Thin-section studies. London: British Museum Research Laboratory.
Garrett, E. M.1986. A petrographic analysis of Black Mesa ceramics. In Spatial organization and exchange, ed. S.Plog. Carbondale: Southern Illinois University Press. 114–42.
Germann, K., et al. 1988. Provenance characteristics of Cycladic (Paros and Naxos) marbles: A multivariate geological approach. In Classical marble: Geochemistry, technology, trade, ed. N.Herz and M.Waelkens. Dordrecht: Kluwer. 251–62.
Goodhew, P. J.1988. Electron microscopy and analysis. New York: Taylor and Francis.
Goren, Y., and P.Goldberg. 1991. Petrographic thin sections and the development of Neolithic plaster production in northern Israel. Journal of Field Archaeology18(1):131–37.
Harrell, J. A.1992. Ancient Egyptian limestone quarries: A petrological study. Archaeometry34(2):195–211.
Herz, N.1955. Petrofabrics and classical archeology. American Journal of Science253:299–305.
Hipkiss, E. J., W. J.Young, and G. H.Edgell. 1937. A modified tomb monument of the Italian Renaissance. Bulletin of the Museum of Fine Arts35:83–90.
Hodges, H.W.M.1963. The examination of ceramic materials in thin section. In The scientist and the archaeologist, ed. E.Pyddoke. New York: Roy. 101–10.
Holmes, L. L., C. T.Little, and E. V.Sayre. 1986. Elemental characterization of medieval limestone sculpture from Parisian and Burgundian sources. Journal of Field Archaeology13(4):419–38.
Hutchison, C. S.1974. Laboratory handbook of petrographic techniques. New York: John Wiley.
Hyman, D. S.1970. Precolumbian cements. Ph.D. diss., Johns Hopkins University, Baltimore, Md.
Keith, V., and M.Cooper. 1974. Nonparametric design and analysis. Ottowa: University of Ottowa Press.
Kempe, D.R.C.1982. Nature and source of the Gandhara sculptural schist. Journal of Archaeological Science9:25–28.
Kempe, D.R.C.1986. Gandhara sculptural schist: Proposed source. Journal of Archaeological Science13:79–87.
Kempe, D.R.C., and A. P.Harvey, eds. 1983. The petrology of archaeological artefacts. Oxford: Clarendon Press.
Kerr, P. F.1977. Optical mineralogy. New York: McGraw-Hill.
Klemm, D. D., and R.Klemm. 1991. Mortar evolution in the Old Kingdom of Egypt. In Archaeometry '90, ed. E.Pernicka and G. A.Wagner. Basel: Birkh�user Verlag. 445–54.
Koh Choo, C. K.1992. A preliminary scientific study of traditional Korean celadons and their modern developments. In Materials issues in art and archaeology III, Materials Research Society Symposium Proceedings 267. ed. P. B.Vandiver, J. R.Druzik, G. S.Wheeler, and I. C.Freestone. Pittsburgh: Materials Research Society. 633–38.
Kozlowski, R., et al. 1990. Decay and conservation of Pincz�w porous limestone. Part 1. Lithology and weathering. Studies in Conservation35:205–21.
Kuntze, R. A.1984. The chemistry and technology of gypsum. Philadelphia: American Society for Testing and Materials.
Lahanier, C.1976. Laboratoire de recherche des mus�es de France examen en laboratoire d'une t�te de roi barbare. Arts Asiatiques32:80–81.
Lazzarini, L.1990. Rosso Antico and other red marbles used in antiquity: A characterization study. In Marble: Art historical and scientific perspectives in ancient sculpture, ed. M.True and J.Podany. Malibu: J. Paul Getty Museum. 237–52.
Lazzarini, L., G.Moschini, and B. M.Stievano. 1980. A contribution to the identification of Italian, Greek and Anatolian marbles through a petrological study and evaluation of ca/sr ratio. Archaeometry22(2):173–83.
Lepsius, G. R.1890. Griechische Marmorstudien. Berlin: Konige.
Loretto, M. H.1984. Electron beam analysis of materials. New York: Chapman and Hall.
MacKenzie, W. S., C. H.Donaldson, and C.Guilford. 1982. Atlas of igneous rocks and their textures. New York: John Wiley.
MacKenzie, W. S., and C.Guilford. 1981. Atlas of rock-forming minerals in thin section. Essex: Longman.
MacMahon, D. A.1988. Archeological collections management at the Saugus iron works national historic site. Boston: U.S. Department of the Interior.
Marinos, G. P.1948. Notes on the structure of Greek marbles. American Journal of Science246: 386–89.
Martinez, G. M., and E. N.Martinez. 1991. Characterization of stone from the Metropolitan Cathedral and from the facade of the National Museum at Tepotzotl�n, Mexico. Studies in Conservation36:99–110.
Matthew, A. J., A. J.Woods, and C.Oliver. 1991. Spots before the eyes: New comparison charts for visual percentage estimation in archaeological material. In Recent developments in ceramic petrology, ed. A.Middleton and I.Freestone. London: British Museum Research Laboratory. 211–64.
Meyers, P., and L.vanZelst. 1977. Neutron activation analysis of limestone objects: A pilot study. Radiochimica Acta24:197–204.
Middleton, A. P., I. C.Freestone, and M. N.Leese. 1985. Textural analysis of ceramic thin sections: Evaluation of grain sampling procedures. Archaeometry27(1):64–74.
Middleton, A. P., M. N.Leese, and M. R.Cowell. 1991. Computer-assisted approaches to the grouping of ceramic fabrics. In Recent developments in ceramic petrology, ed. A.Middleton and I.Freestone. London: British Museum Research Laboratory. 265–76.
Middleton, A., and I.Freestone, eds. 1991. Recent developments in ceramic petrology. London: British Museum Research Laboratory.
Milam, B., C. L.Reedy, and C.Sussman. 1988. Technical analysis of Renaissance bronzes for provenance studies: Pilot project. Technical report submitted to the Getty Conservation Institute, Marina del Rey, Calif.
Moens, L. et al. 1988. A multi-method approach to the identification of white marbles used in antique artefacts. In Classical marble: Geochemistry, technology, trade, ed. N.Herz and M.Waelkens. Dordrecht: Kluwer: 243–50.
Moens, L., et al. 1989. Chemical and petrographical identification of white marbles from the Mediterranean area. In Proceedings of the 25th International Archaeometry Symposium, ed. Y.Maniatis. Amsterdam: Elsevier. 613–25.
Moens, L., et al. 1990. Scientific provenance determination of ancient white marble sculptures using petrographic, chemical, and isotopic data. In Marble: Art historical and scientific perspectives in ancient sculpture, ed. M.True and J.Podany. Malibu: J. Paul Getty Museum. 111–24.
Morton, A. W.1985. Heavy minerals in provenance studies. In Provenance of arenites, ed. G. G.Zuffa. Boston: D. Reidel Publishing. 249–77.
Newman, R.1984. The stone sculptures of India. Cambridge, Mass.: Harvard University Art Museums.
Newman, R.1988. The materials of Hoysala temples and sculptures: A petrographic characterization. Archaeometry30:120–31.
Newman, R.1990. Weathering layers and the authentication of marble objects. In Marble: Art historical and scientific perspectives on ancient sculpture, ed. M.True and J.Podany. Malibu: J. Paul Getty Museum. 263–82.
Newman, R.1991. A compositional and microstructural study of Korean celadon glazes of the 11th to 15th centuries. In Materials issues in art and archaeology, II, Materials Research Society Symposium Proceedings 185, ed. P. B.Vandiver, J.Druzik, and G. S.Wheeler. Pittsburgh: Materials Research Society. 429–40.
Newman, R.1992. Applications of petrography and electron microprobe analysis to the study of Indian stone sculpture. Archaeometry34(2):163–74.
Peacock, D.P.S.1970. The scientific analysis of ancient ceramics: A review. World Archaeology1: 375–89.
Phillips, W. R.1971. Mineral optics: Principles and techniques. San Francisco: W. H. Freeman.
Porai-Koshits, E. A., ed. 1973. Phase-separation phenomena in glasses. New York: Consultants Bureau.
Reed, S.J.B.1993. Electron microprobe analysis. Cambridge, Eng.: Cambridge University Press.
Reedy, C. L.1991. Petrographic analysis of casting core materials for provenance studies of copper alloy sculptures. Archeomaterials5(2):121–63.
Reedy, C. L.1992a. Gandhara and related terracotta technologies. In The crossroads of Asia, ed. E.Errington and J.Cribb. Cambridge, Eng.: Ancient India and Iran Trust. 278–80.
Reedy, C. L.1992b. Petrographic analysis of Gandhara stone sculptures. In The crossroads of Asia, ed. E.Errington and J.Cribb. Cambridge, Eng.: Ancient India and Iran Trust. 264–77.
Reedy, T. J., and C. L.Reedy. 1994. Statistical analysis in conservation science. Archaeometry36(1): 1–23.
Renfrew, C., and J. S.Peacey. 1968. Aegean marble: A petrological study. Annual of the British School at Athens63:45–66.
Roos, P., et al. 1988. Chemical and petrographical characterization of Greek marbles from Pentelikon, Naxos, Paros, and Thasos. In Classical marble: Geochemistry, technology, trade, ed. N.Herz and M.Waelkens. Dordrecht: Kluwer. 263–72.
Rossi-Manaresi, R.1982. Scientific investigation in relation to the conservation of stone. In Science and technology in the service of conservation, ed. N. S.Brommelle and G.Thomson. London: International Institute for Conservation. 39–45.
Saleh, S. A., et al. 1991. Study and consolidation of sandstone temple of Karnak, Luxor, Egypt. Studies in Conservation37:93–104.
Scholle, P. A.1979. A color illustrated guide to constituents, textures, cements, and porosities of sandstones and associated rocks. Tulsa: American Association of Petroleum Geologists.
Shepard, A. C.1942. Rio Grande glazed paint ware: A study illustrating the place of ceramic technological analysis in archaeolgical research. Washington, D.C.: Carnegie Institution of Washington.
Simmons, J. H., D. R.Uhlmann, and G. H.Beall, eds. 1982. Nucleation and crystallization in glass. Columbus: American Ceramic Society.
Stoltman, J. B.1989. A quantitative approach to the petrographic analysis of ceramic thin sections. American Antiquity54(1):147–60.
T�rkmenoglu, A. G., E. H.G�t�rk, and E. N.Caner. 1991. The deterioration of tuffs from the Cappadocia region of Turkey. Archaeometry33(2):231–38.
Vagts, L.1993. The preliminary examination of modified colloidal silica as a consolidant for frescoes. Art conservation training programs student papers, 18th annual conference. Kingston, Ontario: Queens University Art Conservation Program.
Washington, H. S.1898. The identification of marbles used in Greek sculpture. American Journal of Archaeology. 2:1–18.
Weiss, L. E.1954. Fabric analysis of some Greek marbles and its applications to archeology. American Journal of Science252:641–62.
Whitbread, I. K.1989. A proposal for the systematic description of thin sections towards the study of ancient ceramic technology. In Archaeometry, ed. Y.Maniatis. Amsterdam: Elsevier. 127–38.
Williams, D. F.1983. Petrology of ceramics. In The petrography of archaeological artefacts, ed. D.R.C.Kempe and A. P.Harvey. Oxford: Clarendon Press. 301–29.
Williams, H., F. J.Turner, and C. M.Gilbert. 1982. Petrography: An introduction to the study of rocks in thin sections. San Francisco: W. H. Freeman.
Yardley, B.W.D.1990. Atlas of metamorphic rocks and their textures. New York: John Wiley.
Young, W. J., and B.Ashmole. 1968. The Boston relief and the Ludovisi throne. Bulletin of the Museum of Fine Arts66:124–66.
AUTHOR INFORMATION
CHANDRA L. REEDY received her Ph.D. from the interdisciplinary Archaeology Program at the University of California, Los Angeles. Her dissertation research focused on materials analysis of art and archaeological objects and on the South Asian region. After serving as associate conservation scientist at the Los Angeles County Museum of Art, she joined the faculty of the University of Delaware Art Conservation Department. She coordinates the Art Conservation Research Ph.D. Program and teaches methodology of scientific research, provenance and authenticity studies, archaeological theory, and materials and technology of inorganic objects. Address: Art Conservation Dept., University of Delaware, 303 Old College, Newark, DE 19716.
Section Index |