THE HISTORY AND SIGNIFICANCE OF THE SCHREGER PATTERN IN PROBOSCIDEAN IVORY CHARACTERIZATION
EDGARD O'NIEL ESPINOZA, & MARY-JACQUE MANN
ABSTRACT—Proboscidean ivories display a uniquely identifiable pattern of crossing lines in transverse section. Until recently this pattern, properly called the Schreger Pattern, was effectively indistinguishable in the three main commercial sources of proboscidean ivory: the woolly mammoth and two modern elephant genera. The Schreger Pattern contains apparent angles that are acute (<90�) in mammoth ivory and obtuse (>115�) in modern elephant ivories. The ability to differentiate extinct mammoth from modern elephant ivories in carved objects by Schreger angle measurement facilitates international enforcement of laws concerned with protection of elephants through ivory trade restrictions. It also helps the efforts of the art conservation and archaeological communities through improved curatorial identification potential.
1 INTRODUCTION
Ivory refers to commercially significant teeth or tusks large enough to be carved or scrim-shawed (superficially inscribed). There are numerous sources of ivory (Espinoza and Mann 1991), but elephant ivory, formerly considered the only “true” ivory (Owen 1856; Hanausek 1907; Penniman 1952) is by far the most recognized type. Each ivory type is morphologically distinct.
Proboscidean ivory is distinguishable from other ivory forms by the presence of a unique pattern of crossing lines visible in transverse tusk sections. This pattern is a reflection of underlying micromorphology and the focus of a nondestructive forensic method for morphologically differentiating between elephant and mammoth ivories.
2 HISTORY
Much of the current knowledge of proboscidean ivory morphology is based on observations published in the previous century. Two of the most respected names in 19th-century odontology, Bernhard Schreger and Richard Owen, are associated with the history and scientific analysis of ivory.
Schreger is credited with the description of Hunter-Schreger Bands in enamel. Schreger (1800) published observations on human and animal “Habitus der Knochensubstanz” (dentine) and “Schmelz” (enamel). Referring to dentine, he wrote “The differences of the direction of the stripes: namely it [the direction] depends on the form of the base of the inner cavity of the tooth … thus they [the stripes] tend to point in a simple arch with a concavity toward the hole” (p. 2). In contrast, with respect to enamel substructure, he referred to “distinguishable layers or bands” (p. 2).
Obermayer (1881) referred to Schreger's work. In the section on elephant ivory Obermayer stated, “First described by Schreger (1800) these lines characteristic of genuine ivory … carry the name ‘Schreger Lines’” (p. 106). Two subsequent German-language publications (H�hnel 1892;Hanausek 1907) use the term “Schreger Lines” as an accepted scientific term. After 1907, however, references to “Schreger Lines” seem to have disappeared from the literature.
Sir Richard Owen (1845, 1854, 1856) also published on the subject of proboscidean ivory pattern, and his work is cited in studies in English (Miller 1890; Penniman 1952; Miles and White 1960; Brown and Moule 1977) and German (Obermayer 1881; H�hnel 1892). Although Owen is clearly associated with the proboscidean ivory pattern, his name was given to a system of concentric dentine growth lines (Miles and White 1960) and not to the pattern itself. The term “Schreger Lines” was not used by English-language researchers, who chose Owen's descriptors for the proboscidean ivory pattern: “decussation,” “curvilinear,” “lozenge.”
Exclusive use of subjective and antiquted descriptors is inappropriate in a forensic or legal forum. Although utilization of the term “Schreger Lines” is limited to three authors, it appears to be the earliest known and accepted scientific appellation for the proboscidean ivory pattern. In deference to the historical precedent set by these early authors, and because of our need for consistency, we refer to the pattern of crossing lines in proboscidean ivory as the Schreger Pattern.
3 SIGNIFICANCE
Elephants are protected by the Convention on International Trade in Endangered Species (CITES) treaty. Ivory from the Asian elephant (Elephas maximus) has been banned from importation into the United States since 1976 (41 Federal Register 24064) and African elephant (Loxodonta africana) ivory was banned in 1989 (54 Federal Register 24758).
Mammoth ivory is excluded from import restrictions. Although many extinct elephant-like mammals, such as the Gomphotherium, the Stegodon, and the mastodon left tusk material in the fossil record (Fenton and Fenton 1958), only Alaskan and Siberian woolly mammoths (Mammuthus primigenius) produced ivory that has been sufficiently well preserved to be of value for trade.
Mammoth ivory has been a significant commercial entity for centuries. Between 1809 and 1910 the Siberian ivory mining industry extracted nearly 6,000 metric tons of mammoth tusks (Tolmachoff 1929); over the last 350 years, approximately 7,000 tons of mammoth ivory have been imported into China (Bruemmer 1989), and it has been estimated that 550,000 tons of mammoth tusks have yet to be recovered from a 1,000 km coastal strip between the Yana and Kolyma rivers in Siberia (Vereshchagin 1974).
Carved mammoth ivory is remarkably similar in appearance to elephant ivory. This resemblance, and the relative abundance of mammoth ivory, presents a significant impediment to the international enforcement of elephant ivory trade bans. In response, we have developed a method to objectively and nondestructively differentiate mammoth from elephant ivory in carved objects using the Schreger Pattern.
The Schreger Pattern is a system of crossing lines visible in transverse section of proboscidean ivory. The intersections of these lines form apparent concave and convex angles. The concave form has slightly concave side elements and opens medially. The convex form opens laterally and has slightly convex side elements. The Schreger Pattern can have two expression areas consisting of inside (medial) and outside (lateral) pattern areas. The inside pattern area usually contains faintly expressed, tightly packed angles. The outside pattern area consists of easily visible angles. A null or neutral pattern area may exist in the transition zone between inside and outside pattern areas (fig. 1).
Fig. 1.
A transverse section of Loxodonta africana ivory showing the outer and inner Schreger Pattern areas. Bar equals 1.25 cm
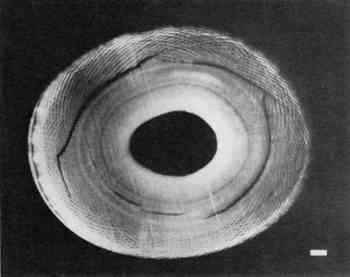 |
Penniman (1952, 21) observed that the “intersecting convex lines are finer, thinner, and closer together (in mammoth ivory), making narrower and longer angles with each other.” We verified this observation by analyzing Schreger Pattern angles and examining the dentine of known samples of elephant and mammoth ivories.
4 METHODS
Fifty-two mammoth and elephant ivory samples were examined visually and processed statistically. Our elephant sample consisted of 26 cut and polished transverse dentine sections of Loxodonta africana (n = 4), Elephas maximus (n = 1), and unspecified species of extant elephant (n = 21). The mammoth (Mammuthus primigenius) sample consisted of 26 identically prepared sections. The samples, each representing a randomly excised section from an individual tusk, were obtained from museum and zoo collections, whole or largely intact confiscated tusks, and commercial sources. Five concave and five convex angles from the outside expression area of each sample were collected and analyzed according to Espinoza et al. (1990).
Ivory from three Elephas maximus, three Loxodonta africana, five unspecified species of modern elephant, and five Mammuthus primigenius were examined using scanning electron microscopy. Five mm square blocks of ivory from tusk sections of equivalent diameter and central cavity configuration were prepared by chisel fracture. The blocks were glued to 2.5 cm SEM stubs, sputter coated with gold, and examined using a CamScan Series 4 scanning electron microscope at magnifications from 500x to 4,000x.
5 OBSERVATIONS AND DISCUSSION
We obtained intertusk Schreger angle data from the outside pattern area to document angle variance within and along a single tusk. Five convex and five concave outside angle measurements were obtained from each of 16 sequential sections taken from a Loxodonta africana tusk approximately 90 cm x 12.5 cm.
Pooled convex angle and concave angle measurements from tusk sections ranged between 134.5� and 143� (average = 139.1�, standard deviation = 2.37) with no significant variance observed from section to section. Sectional convex and concave angle data were then converted to radians for statistical purposes and compared to each other by t-test statistic with p<0.05 considered significant. The t-test statistic tests the hypothesis (HO) that there is no difference between the convex and concave angle data, where p<0.05 represents the smallest value that would lead to rejection of the hypothesis. No significant difference was observed between the convex and concave Schreger angles within the outside area of a tusk.
Five convex and five concave outside Schreger angle measurements were taken from each Loxodonta and Elephas (n = 26), and from each Mammuthus primigenius (n = 26) tusk sample. For elephant the combined average was 124.2� (standard deviation = 9.28) with a range from 95� to 163�; for mammoth the average was 73.7� (standard deviation = 9.76) with a range from 35� to 115�. Radian-transformed convex and concave angle data from each tusk sample were compared by t-test statistic, with p<0.05 considered significant. No significant statistical difference was observed between convex and concave Schreger angles within individual samples. Since no differences were observed, the data from each tusk sample were averaged to get a single value to represent each individual.
Radian-transformed mean data from each of the 26 elephants and 26 mammoth samples were compared by t-test statistic with p<0.05 considered significant. The t-test statistic tests the hypothesis (HO) that there is no difference between the angle data from extinct and modern samples, where p<0.05 represents the smallest value (t.95 = � 1.671) that would reject the hypothesis. The statistical analysis rejected the hypothesis and showed that the differences in Schreger angle means in modern versus extinct proboscidean samples are statistically significant (t = 19.42). Table 1 shows the distribution in degrees and radians of the mammoth and elephant sample data. Figure 2 presents a graphic summary of all the outer Schreger Pattern angles of extinct and modern proboscidean ivory samples measured for this study. Although not included in the data set, the Schreger angles of three Gomphotherium and three mastodon tusk samples fell within the range (35�–115�) observed in mammoth samples.
TABLE 1 DISTRIBUTION IN RADIANS AND DEGREES OF MAMMUTHUS PRIMIGENIUS AND LOXODONTA/ELEPHAS SCHREGER PATTERN ANGLE MEANS
Fig. 2.
Histogram of all outer Schreger Pattern angles of extinct and extant Proboscidean ivory samples (N = 260 each). This figure is reprinted from Identification guide for ivory and ivory substitutes with permission from the World Wildlife Fund.
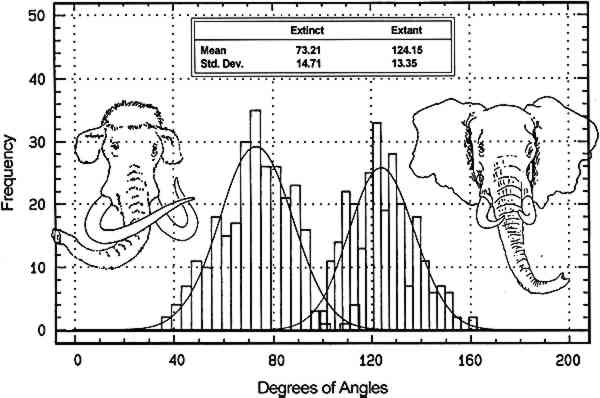 |
Schreger Pattern outer angles in proboscidean ivories clearly differentiate between extinct (legal) and modern (illegal) sources, and micromorphology is the basis for these angle expression differences.
The Schreger Pattern is a shadow phenomenon created primarily by the structure of microscopic dentinal tubules (Miller 1890; Miles and White 1960; Miles and Boyde 1961); the contribution of laminar dentine deposition to the Schreger Pattern is suggested but not fully understood. Dentinal tubules are fluid transport microcanals that radiate medially to laterally in rows from the center of the tusk, like the spokes of a wheel. Tubule diameter ranges from approximately 0.8 μm to 2.2 μm, and proboscidean dentinal tubules are sinusoidal. Both mammoth and modern elephant dentinal tubules have peak to peak sinusoidal wave distances of 0.8 to 2.5 mm. The visual perception of the Schreger Pattern is a function of intertubule distances and the wave alternation of adjacent dentinal tubule rows.
Scanning electron microscopic examinations of Loxodonta, Elephas, and Mammuthus ivories revealed differences in tubule density. Tusks with effectively equal macromorphology have more dentinal tubules per unit area in mammoths than in modern elephants (fig. 3).
Fig. 3.
Radial section scanning electron micrographs of (left) Mammuthus primigenius and (right) Loxodonta africana illustrating the tubule density per area difference between extinct and modern proboscidean ivories. Bar equals 10 μm, original magnification 1000 x
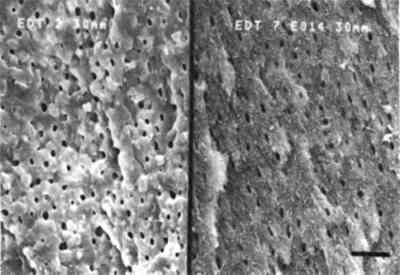 |
The effect of greater tubule density creates shadow lines that form small (acute) Schreger angle patterns such as those seen in mammoth ivory. Fewer tubules per unit area, as observed in Loxodonta and Elephas ivories, result in greater distance between shadows and therefore greater (obtuse) angles (fig. 4).
Fig. 4.
Transverse sections of (left) Mammuthus primigenius and (right) Loxodonta africana ivories showing the acute angle Schreger Pattern angles in Mammuthus and obtuse Schreger Pattern angles in Loxodonta. Bar equals 1 cm
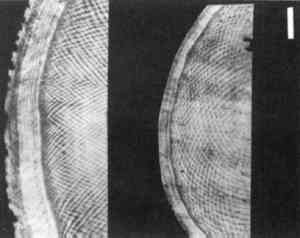 |
The Schreger Pattern is a unique and reliable identifier of proboscidean ivory. Statistical analysis and microscopic observations show that the Schreger Pattern can also serve to nondestructively and objectively differentiate between elephant and mammoth ivories.
ACKNOWLEDGEMENTS
The authors thank Larry Agenbroad, William Gould, Clayton Ray, and Richard Tedford for contributing mammoth ivory standards used in this study. We also thank Bernhard Binder, Evelyn Burchett, and Susan Taylor and for translations of the Schreger and Obermayer papers, and Stephen D. Busack for critical review of this manuscript.
REFERENCES
Bruemmer, F.1989. Arctic treasures. Natural History98:39–46.
Brown, G., and A. J.Moule. 1977. The structural characteristics of elephant ivory. Australian Gemmologist13:13–17.
Espinoza, E. O., and M. J.Mann. 1991. Identification guide for ivory and ivory substitutes. Baltimore, Md.: World Wildlife Fund and Conservation Foundation.
Espinoza, E. O., M. J.Mann, J. P.LeMay, and K. A.Oakes. 1990. A method for differentiating modern from ancient Proboscidean ivory in worked objects. Current Research in the Pleistocene7:81–83.
Fenton, C. L., and M. A.Fenton. 1958. The fossil book. Garden City, N. Y.: Doubleday.
Hanausek, T. F.1907. The microscopy of technical products, trans. A. L.Wilton. New York: John Wiley and Sons.
H�hnel, V.1892. Beitrag zur kenntniss der technisch ver wendeten elfenbeinarten. Zeitschrift f�r Nahrungsmittel-Untersuchung, Hygiene und Waarenkunde7:141–44, 9:183–88, 10:205–11. German.
Miles, A.E.W., and A.Boyde. 1961. Observations on the structure of elephant ivory [abstract]. Journal of Anatomy95: 450.
Miles, A. E. W., and J. W.White. 1960. Ivory. Proceedings of the Royal Society of Medicine53:775–80.
Miller, W. D.1890. Studies on the anatomy and pathology of the tusks of the elephant. Dental Cosmos32:337–48.
Obermayer, F.1881. Beitrag zur kenntnis des zahnbeines vom elefanten, nilpferd, walross und narwal. Verlag des Vereines der Wiener Handels-Akademie102–13. German.
Owen, R.1845. Odontography. London.
Owen, R.1854. The principal forms of the skeleton and of the teeth. Philadelphia, Pa.: Blanchard and Lea.
Owen, R.1856. Ivory and the teeth of commerce. Journal of the Royal Society of Arts5:65–71.
Penniman, T. K.1952. Pictures of ivory and other animal teeth, bone and antler. Occasional Paper on Technology5:4–40.
Schreger, B.N.G.1800. Beitrag zur geschichte der z�hne. Beitrage f�r die Zergliederungskunst1:1–7. German.
Tolmachoff, I. P.1929. The carcasses of the mammoth and rhinoceros found in the frozen ground of Siberia. Transactions of the American Philosophical Society23:12–14.
Vereshchagin, N. K.1974. The mammoth “cemeteries” of North-East Siberia. Polar Record17:3–12.
AUTHOR INFORMATION
EDGARD O'NIEL ESPINOZA is the chief chemist and Criminalistics Section chief at the National Fish and Wildlife Forensic Laboratory in Ashland, Oregon. His areas of expertise are organic chemistry, general criminalistics, wildlife toxicology, and ivory identification. Along with administrative duties and the examination of evidence submitted in connection with crimes against wildlife, Espinoza maintains a program of research and methods development in the chemical-instrumental identification of parts and products from endangered and protected species. He holds a B.S. in medical technology from Loma Linda University in California, and M.P.H and Dr. P.H. degrees in forensic science from the University of California, Berkeley. Espinoza was formerly an assistant professor of forensic sciences at Sacramento State University in California and a private practice forensic consultant with a specialization in homicide cases. Address: National Fish and Wildlife Forensic Laboratory, 1490 E. Main St., Ashland, Oreg. 97520.
MARY-JACQUE MANN is a senior forensic scientist in the Criminalistics Section of the National Fish and Wildlife Forensic Laboratory in Ashland, Oregon. Her areas of expertise are scanning electron microscopy, trace evidence analysis, firearms comparisons, and ivory identification. Mann has a bachelor's degree in biology from Radford University in Virginia and an M.F.S. in forensic science from George Washington University in Washington, D.C. She was a scanning electron microscopist with the Smithsonian Institution's National Museum of Natural History for 10 years before she became interested in the forensic sciences. Immediately prior to accepting her current position, she was a forensic analyst for six years with the New Mexico State Police Crime Laboratory in Santa Fe, N.M. Address: National Fish and Wildlife Forensic Laboratory, 1490 E. Main St., Ashland, Oreg. 97520.
Section Index |