EFFECTS OF AQUEOUS LIGHT BLEACHING ON THE SUBSEQUENT AGING OF PAPER
TERRY TROSPER SCHAEFFER, MARY T. BAKER, VICTORIA BLYTH-HILL, & DIANNE VAN DER REYDEN
ABSTRACT—The effects of aqueous light bleaching of a naturally aged, 100% cotton rag, gelatin-sized paper on the subsequent aging of that paper have been investigated. Effects of exposure to various control conditions were compared with those due to aqueous light bleaching. Physical properties of all papers were monitored by measurement of surface pH, optical properties, stress-strain tensile behavior, and presence of sizing. Measurements were made before and after treatments and after artificial aging at 90�C and 50% RH for 20 days. An unsized filter paper, made from 100% cotton fibers, was exposed to identical conditions, and the same properties were measured. For the gelatin-sized paper, aqueous light bleaching resulted in less color reversion after artificial aging than did exposure to any of the control conditions. However, the sized paper specimens exhibited significant change in tensile properties. Similar responses were also observed in controls that were immersed in the dark. The changes noted are likely to be attributable to immersion temperature. All paper specimens were significantly embrittled by artificial aging. The changes in tensile properties of both types of papers upon aging did not appear to be influenced by exposure to light during the bleaching procedure.
1 INTRODUCTION
The technique of aqueous light bleaching to diminish stains in paper was first brought to the attention of paper conservators in 1980 by Keyes at the International Conference on Conservation of Library and Archive Materials and the Graphic Arts in Cambridge, England. Simply stated, the technique utilizes exposure to light from the sun (e.g., van der Reyden 1981; Eldridge 1982 or an artificial light source such as fluorescent lamps (e.g., Branchick et al. 1982; Baker 1986), preferably with far ultraviolet radiation removed by filtration, to reduce discoloration in paper while the paper is submerged in a bath of purified and buffered water. Many variations in the procedure have been used, such as the “moisturizing sandwich” (Keyes 1980). However, specific requirements for successful application of aqueous light bleaching have been found to be: prebathing the object, using a buffered or slightly alkaline immersion solution, and restricting treatment to low lignin content paper. Because the procedure is straightforward and an effective tool, many paper conservators have incorporated it into their stain removal repertoire. Their reasons for using this bleaching method are many: (1) It is relatively easy to control; (2) the color of the paper is quite natural after treatment, as opposed to the sometimes overly bright white of chemical bleaching; (3) the paper feels stronger afterward; and (4) the procedure avoids the introduction of another extraneous chemical into an already degraded paper.
The chromophores in discolored paper may be degradation products of cellulose or sizing, or they may have been formed by interactions among these chemicals or between them and other inclusions or impurities in the paper, such as metal ions or lignins (Feller et al. 1982). When an immersed paper object is bleached by light, the chromophores are altered to colorless products that may or may not be removed from the paper, depending on details of the treatment procedures and on whether the products are still covalently bound to polymeric material (Burgess 1988). The bleaching reactions proceed via absorption of light energy by a chromophore, which is then converted to a reactive peroxide or free radical (Phillips 1985). These substances in turn cause a chemical change, usually an oxidation process. In this respect aqueous light bleaching is not fundamentally different from chemical bleaching. However, the former has the advantages that no extraneous chemical reagent is added to the paper and that excess reagent need not be removed to stop the bleaching reaction. It is thus more easily controlled.
The possible disadvantages of aqueous light bleaching, which are similar to some of those incurred with other bleaching treatments, are that the absorbed light energy may: (1) cause formation of colored products in the components of the paper; (2) catalyze formation of colorless degradation products in the exposed paper, which may degrade further to colored compounds upon subsequent aging (Burgess 1988); and (3) induce degradation of the paper compounds, which could result in changes in mechanical properties.
Several investigators have addressed the above-mentioned possibilities, studying the immediate effects of aqueous light bleaching on the properties of various new and old papers. The surface strength of some papers appears to be increased (van der Reyden 1981), while the tensile strength appears to remain unchanged (van der Reyden et al. 1988); colored substances will appear in some types of papers (Savard 1986); increased efficiency of the treatment may or may not be related to an increase of the pH of the solution(Eldridge 1982); and changes in degree of polymerization (DP) of cellulose after treatment appear to be indetectable (LePage and Perron 1986; Lee et al. n.d.).
The effects of the aqueous light bleaching treatment on the subsequent aging of paper have received less attention (Savard 1986; Lienardy and van Damme 1989; Hofmann et al. 1991; Flieder et al. 1991; Lee et al. n.d.). Color reversion will occur, but the extent of this reversion compared to that of aged, unbleached control papers has not always been quantified. The mechanical properties of treated, aged papers have received little unequivocal documentation. Few studies have included aged papers likely to have been used by artists, such as the 1956 protein-sized cotton paper used in this study.
An earlier study performed at the Conservation Analytical Laboratory, Smithsonian Institution (CAL) was undertaken to determine to what extent various aspects of an aqueous light-bleaching treatment would affect the color and tensile properties of certain papers (van der Reyden et al. 1988). In these experiments a naturally aged, mixed pulp, alum rosin-sized paper and unsized mixed pulp and cotton linter papers were aqueously light bleached or subjected to various control conditions (e.g., dark immersed or exposed to light while dry) for up to 96 hours in a Weather-ometer. Measurements of paper color before and after treatments showed that the aqueous light bleaching conditions were by far the most effective in removing discoloration from the mixed pulp papers and that the greatest decrease in discoloration occurred in the first 2 hours under these experimental conditions. Interestingly, neither aqueous light bleaching nor dark immersion, even at the elevated temperatures reached in the Weather-ometer, had a significant effect on either the ultimate strengths or the strain to break of the mixed pulp test papers when compared to washed controls that were not exposed to any of the Weather-ometer conditions. A recent investigation of the properties of these papers upon subsequent aging has been reported separately (Schaeffer et al. 1991).
The aqueous light bleaching research project at CAL has now been extended to include a study of the effects of accelerated aging on color and tensile properties of two papers that represent a modern control paper and a naturally aged artists' paper. As in the previous study, the papers were aqueously light bleached, or subjected to various control conditions, in the Weather-ometer (fig. 1). Physical and chemical properties were monitored before and after the exposures and after artificial, humid oven, aging.
Fig. 1.
Flow chart showing different experimental conditions to which papers were exposed during the course of the experiment
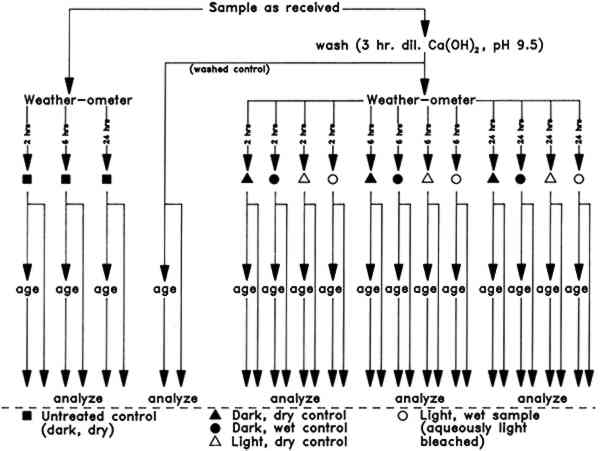 |
The results of this study, indicate that aqueous light bleaching is an effective method for decreasing discoloration in the papers used and also that less color reversion occurs upon artificial aging of samples which received the aqueous light-bleaching treatment as compared to papers subjected to various control conditions. However, potentially disadvantageous alterations in other properties of the papers did occur. These changes did not appear to be directly attributable to light exposure, although they suggest that further detailed study of the conditions for treatment should be undertaken before aqueous light bleaching can be employed without reservation as a conservation technique.
2 MATERIALS AND METHODS
2.1 PAPERS
The papers used in this study were:
- Whatman 1 (hereafter W1) filter paper, manufactured from 100% cotton seed hairs without sizing, used as an appropriate unsized control. An analysis of this paper was reported previously (van der Reyden et al. 1988).
- English artists' rag paper bearing the watermark “J. Whatman 1956” (hereafter W56), made of 100% cotton rag sized with alum and gelatin.1
Analytical procedures, including spot tests, optical and scanning electron microscopy (SEM), energy-dispersive x-ray analysis (EDX), and Fourier transform infrared spectrometry (FTIR), were used to assess the overall chemical composition and condition of the paper specimens and to monitor changes in these properties. Not all the tests were applied to all paper specimens at each stage of the experiment, especially in the case of the W1 unsized control paper. Details of these procedures are given in the appendix.
The information obtained from analyses of W1 and W56 papers “as received” (untreated) and after washing confirmed the manufacturer's specifications.
Elemental analysis of the untreated W56 paper by EDX documented the presence of aluminum and sulfur. After washing, the presence of these elements was barely detectable. The observations are consistent with removal of a major portion of the alum and other aluminum salts during the washing step.
Scanning electron micrographs of washed papers showed a significant increase in the cross-sectional area of most fiber lumens. Greater variation in interfiber spaces was also evident. Both these observations may be related to the ca. 15% increase observed in nominal thickness of the washed W56 paper, from 0.0165 � 0.0007 cm before to 0.0196 � 0.0005 cm after washing (n = 5).
2.2 EXPERIMENTAL DESIGN
All the W1 and W56 papers were handled as works of art on paper would be, with the additional precaution that plastic gloves were always worn when the papers had to be manipulated by hand. The overall experimental protocol is diagrammed in figure. 1. Details of the procedures are given in the appendix.
2.2.1 Washing and paper preparation
A piece of each paper was reserved as an untreated control. Other sheets of the W56 and W1 papers were washed by soaking them individually in a Ca(OH)2 solution, initial pH 9.5, and then drying them between blotters, felts, and Plexiglas under weight. This preliminary step was undertaken to simulate the usual conservation procedure of washing a discolored paper object in an alkaline bath before bleaching as well as to provide washed controls to isolate the effects of alkaline water alone. The untreated papers and the washed papers were cut into specimens of appropriate size for exposure to aqueous light bleaching or the other, control, conditions described below.
2.2.2 Aqueous light bleaching and control exposure conditions
Aqueous light bleaching was carried out in an Atlas Ci35 Weather-ometer, with each individual paper specimen suspended in a 600 ml flat-sided polystyrene culture bottle as described previously (van der Reyden et al. 1988). Thirty such bottles could be placed in the Weather-ometer chamber at one time, permitting 15 specimens of W1 paper and 15 specimens of W56 paper to be exposed simultaneously. In practice, 3 specimens of each paper were exposed to the same conditions, but for three different time periods: 2, 6, or 24 hours. Thus, five different experimental conditions were used for each of the two papers (see fig. 1). These were: (1) unwashed control paper, kept dry in the dark; (2) washed control paper kept dry in the dark; (3) washed control paper immersed in Ca(OH)2, initial pH 9.5, in the dark; (4) washed control paper kept dry and exposed to light; and (5) washed sample paper immersed in Ca(OH)2, initial pH 9.5, and exposed to light, which is equivalent to aqueous light bleaching. All dark controls were in bottles that had been wrapped securely in heavy-duty aluminum foil.
One specimen of each paper subjected to each experimental condition was removed from the Weather-ometer in its bottle after each of the specified exposure times. The immersed papers were blotted and dried in a felt and Plexiglas press under weight. After the incubations the pH of all—and the temperatures and protein content of some—immersion solutions was measured. Details of these procedures are included in the appendix.
The entire experiment was performed twice.
2.2.3 Artificial aging
All papers that had been in the Weather-ometer were cut in half. One set of these halves was reserved for analysis. The other set was sewn into Plexiglas frames for humid oven aging. They were artificially aged for 20 days in the dark at 90�C and 50% RH in an Associated Environmental Systems HK-4116 temperature-humidity chamber. These conditions have been used previously for artificial aging studies (Lee et al. 1989b; Erhardt et al. 1987). Washed papers that had not been in the Weather-ometer were aged at the same time. Aged papers were subsequently analyzed using the same procedures as those applied to the un-aged portions of each specimen.
2.3 EVALUATION OF EFFECTS OF TREATMENTS AND AGING ON PAPER PROPERTIES
In addition to the qualitative chemical analyses described in section 2.1, each paper specimen was characterized physically by surface pH, colorimetry, and relaxation tensometry. Experimental details of these analytical procedures are provided in the appendix.
In this study, changes in surface pH have been regarded as a qualitative indicator of changes in the acidity of the paper. Because of the recognized inaccuracy of the measurement, only changes of at least one-half pH unit are considered significant.
Colorimetry was used as a quantitative, objective measure of paper appearance. Reflectances throughout the visible range were recorded, and CIE L∗a∗b∗ values were generated from these. Comparisons of these values for different specimens will indicate not only changes in lightness-darkness, but also shifts in red-green and yellow-blue color coordinates.2 The CIE L∗la∗b∗ data for all papers are given in table 1 and table 3.
TABLE 1 COLORIMETRIC DATA FOR WHATMAN 1 PAPER
TABLE 3 COLORIMETERIC DATA FOR WHATMAN 1956 PAPER
Tensile data obtained with sensitive relaxation tensometers were used to monitor mechanical properties of the papers before and after treatments and artificial aging. To use these data, nominal stress (force on the paper strip divided by cross-sectional area of the strip) was plotted as a function of strain (percent change in length). In these graphs, the relative strength of the paper is indicated by the stress withstood at a given strain; the slope of the curve is an indication of the stiffness; and decreased strain at failure implies increased brittleness of the paper. A summary of tensile data for all papers (ultimate stresses and strains to break) is presented in table 2 and table 4.
TABLE 2 ULTIMATE STRESS AND MAXIMUM STRAIN SUSTAINED BY WHATMAN 1 SAMPLES
TABLE 4 ULTIMATE STRESS AND MAXIMUM STRAIN SUSTAINED BY WHATMAN 1956 SAMPLES
3 RESULTS
3.1 WHATMAN 1 (UNSIZED CONTROL PAPER)
3.1.1 Morphological and chemical characterization
The thickness of W1 paper was not significantly changed by washing, by aqueous light bleaching, or by incubation under any of the other conditions to which it was subjected.
Some treated papers that had been used for measurement of tensile properties were also examined by SEM. Micrographs of the fracture regions of papers which had been in the Weather-ometer for 24 hours indicated that individual fibers did not become brittle and shatter, even after humid oven aging at 90�C and 50% RH for 20 days. Very few short, stubby, broken fiber ends were observed along the entire break in these papers, with no more appearing in the aged than in the unaged papers.
3.1.2 Surface pH
The pH of the untreated W1 control paper was 6.9 � 0.1, and that of the washed control paper was pH 6.5 � 0.1. The surface pH of the W1 papers was significantly decreased by Weather-ometer treatment, typically by 0.3 to 0.7 units. The specimens most severely affected were those exposed to light while dry. The pH of the immersion solutions fell, as expected. All those that had remained in the dark fell to 6.7 � 0.1; the immersion solutions that had been exposed to light had a pH of 6.3 � 0.2.
Humid oven aging lowered the surface pH of all specimens significantly. Least affected were the papers that had not been washed before Weather-ometer treatments. Their surface pH following aging was in the range 5.5–5.7. In contrast, the surface pH's of the washed W1 papers were all in the range 4.7–5.1 after exposures in the Weather-ometer and artificial aging, regardless of the particular conditions to which they were exposed. The papers exposed to light appeared systematically to have pH's at the low end of this range.
3.1.3 Colorimetry
The colorimetry data for all W1 papers are presented in table 1. The untreated W1 filter paper did not appear discolored to the eye. Washing this paper did not have a significant effect on its appearance. Brightness and green-red color values were unchanged, and only a slight decrease in b∗ occurred (decrease in yellow chromophores) (fig. 2). Weather-ometer exposures of washed specimens caused further slight decrease in b∗, with the largest changes occurring in the aqueously light-bleached samples. The extent of this change appears to have been correlated to time of aqueous light bleaching.
Fig. 2.
CIE b∗ values for W1 papers treated in the Weather-ometer for 2, 6, and 24 hours under the conditions indicaated, before and after humid oven aging at 90�C and 50% RH for 20 days
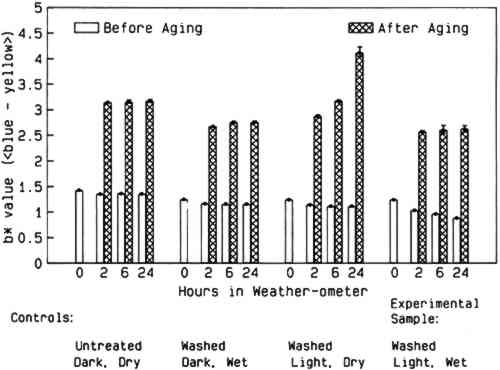 |
Humid oven aging caused a slight darkening of all the W1 papers (lower L∗). Changes in the aqueously light bleached samples are not distinguishable from those in washed controls that were immersed in the dark in the Weather-ometer (table 1). However, exposure of the W1 paper to light while dry resulted in significantly more discoloration upon subsequent humid oven aging (fig. 2). Increases in both red and yellow absorption (increases in a∗ and b∗) were directly related to time of dry light exposure in the Weather-ometer (table 1).
3.1.4 Tensile Measurements
Washing the unsized W1 paper caused a significant increase in maximum strain (1.9 � 0.2% vs. 2.5 � 0.2%) but no statistically significant change in stiffness or ultimate stress to break. Immersion and/or exposure of W1 paper to light in the Weather-ometer caused no statistically significant changes in stress to break (table 2). There were no obvious systematic changes in brittleness due to Weather-ometer exposures. The averaged data (n = 3) for each of the papers exposed for 2 hours to each of the different incubation conditions are shown in figure 3. Aside from the obvious increase in maximum strain of all the washed papers, the tensile characteristics of the specimens can be seen to be very similar despite the disparate exposure conditions they endured.
Fig. 3.
Nominal stress as a function of strain for W1 papers (in the machine direction - MD) before artificial aging. The papers were in the Weather-ometer 2 hours under the conditions indicated. The curves shown are averages of data from triplicate measurements.
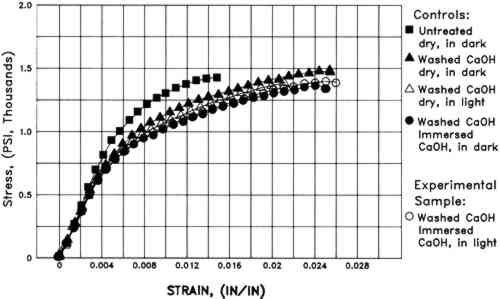 |
Artificial aging caused marked embrittlement but no significant changes in stress to break of the washed paper specimens (fig 4). In addition, papers exposed to light did not display significant differences from the corresponding controls kept in the dark in the Weather-ometer (table 2, fig. 4). Thus none of the changes in tensile properties of W1 paper can be attributed unequivocally to light exposure.
Fig. 4.
Nominal stress as a function of strain for W1 papers (MD), treated as ain figure 3, after artficial aging at 90�C and 50% relative humidity for 20 days. The papers were in the Weather-ometer for 2 hours. the curves averages of triplicate measurements.
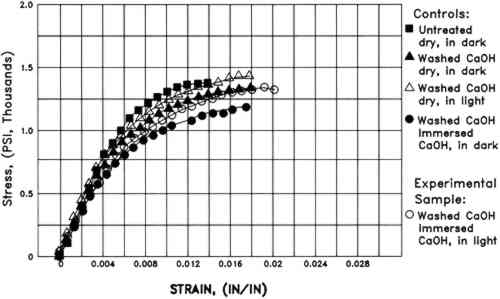 |
3.2 WHATMAN 1956 (ALUM- AND GELATIN-SIZED ARTISTS' PAPER)
3.2.1 Morphological and chemical characterization
All papers on which spot tests were performed gave positive results for both the alum and the ninhydrin protein test. However, W56 paper that had been immersed in the Weather-ometer for 24 hours responded to the ninhydrin test with blurred spots and slower color development, which might be indicative of lesser amounts of protein present. The characteristic protein bands were still present in an FTIR reflectance spectrum of a paper that had been immersed while in the Weather-ometer. Loss of some protein from the W56 paper into the immersion solution was confirmed by Lowry assays of two W56 immersion solutions for protein, using a gelatin protein standard. Both a light and a dark immersion solution had ca 0.25–0.3 mg protein per ml of solution. This result corresponds to a loss of 15–18 mg of gelatin from the paper specimen, or about 6–8% of the weight of the paper before incubation in the Weather-ometer.
Very low levels of aluminum were also detectable by EDX in the papers after they had been in the Weather-ometer, whether or not they had been immersed. This result is consistent with the positive alum spot tests obtained on all the differently treated papers that were tested.
The thickness of the W56 paper increased by approximately 15% after washing, a result that may be due to swelling of the paper as a consequence of removal of size. Immersion during incubation in the Weather-ometer did not cause any additional change in the thickness of W56 papers beyond that observed after the washing step. Artificial aging also had a negligible effect on paper thickness.
3.2.2 Surface pH
Surface pH of the untreated W56 paper was 4.7 � 0.1. The washing procedure raised the surface pH to 5.3 � 0.1. The surface pH of the papers after Weather-ometer treatments did not significantly change. The pH of the immersion solutions fell about 1 unit further than the corresponding W1 immersion solutions did. The extent of decrease was somewhat greater for the aqueous light-bleached samples than for those kept in the dark. It appeared to depend slightly on the length of time in the Weather-ometer.
The surface pH of all washed, aged W56 papers was in the range 4.5–4.7. The value appeared to be independent of type or length of Weather-ometer exposure. The surface pH of all the W56 controls aged without washing or without Weather-ometer exposure was decreased to 4.5 by humid oven aging.
3.2.3 Colorimetry
The CIE L∗a∗b∗ values for all the W56 papers are summarized in table 3. The W56 paper appeared cream colored when received. It was lightened slightly by washing in dilute Ca(OH)2, losing some red- and to a lesser extent, yellow-absorbing material. Immersion and light exposure in the Weather-ometer both caused additional color loss and lightening of the papers. Aqueous light bleaching resulted in a synergistic effect, seen most markedly in the time-dependent decrease in b∗. For example, immersion in the dark or dry light exposure for 2 hours each caused about a 15% decrease in b∗, but aqueous light bleaching resulted in a lowering of b∗ by more than 40% (fig. 5). Thus aqueous light bleaching caused a reduction of yellow chromophores that was greater than the sum of those due to immersion alone or light exposure alone.
Fig. 5.
CIE b∗ values for W56 papers after 2 hours in the Weather-ometer under various conditions, before and after aging
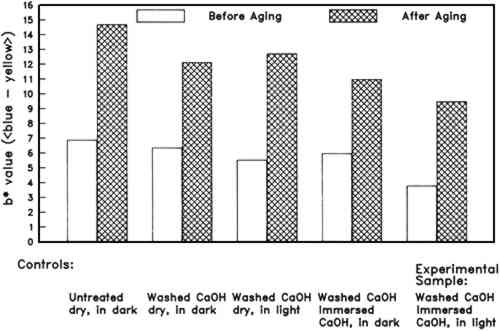 |
Marked color reversion occurred in all W56 papers upon humid oven aging (table 3). The extent of darkening and discoloration depended strongly on the conditions of Weather-ometer incubation. After artificial aging, only the papers that had been immersed remained significantly lighter than the washed control. The samples that were aqueously light bleached for 24 hours were the least discolored by aging. Again, the changes in the amount of yellow-absorbing material were the most dramatic (fig. 5). Of all the washed, Weather-ometer–incubated W56 papers, those that were exposed to the light while dry showed the greatest color reversion. In addition, these were the only papers in which a statistically significant difference between recto and verso color was observed for b∗, and they also had markedly more red-absorbing material. They did not, however, reach the extent of discoloration that the unwashed, untreated W56 paper did upon humid oven aging (table 3).
3.2.4 Tensile measurements
The tensile behavior of the W56 paper as received (untreated) and after washing is shown in figure 6. The washed paper appears to display a slight decrease in stress to break, compared to the unwashed controls. However, the ca. 15% increase in thickness of the W56 paper after the washing step (see above) would contribute substantially to the apparent decrease in nominal stress, which is inversely proportional to the cross-sectional area (= width � thickness) of the paper strip.
Fig. 6.
Nominal stress as a function of strain for W56 paper untreated (MD), and after preliminary washing in aqueous Ca(OH)2 solution as described in the text.middle curves of each set represent averages of measurements on five and three specimens, respectively. The data ranges are represented by the outer curves.
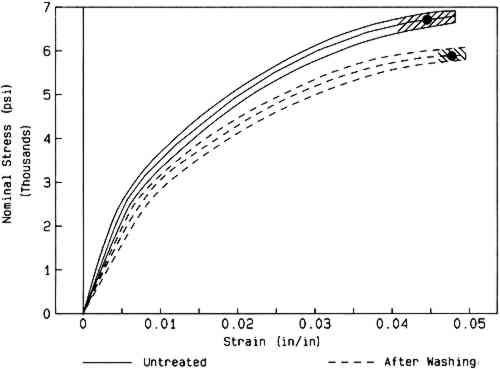 |
The W56 controls that were exposed to dry Weather-ometer conditions did not display significant changes in their tensile properties, whether or not they were exposed to light (table 4; see also fig. 7 for 2 hour Weather-ometer exposures). However, those W56 papers that were immersed while in the Weather-ometer registered a large decrease in stress to break and, in almost all cases, a concomitant decrease in strain to break. The decrease in stress to break was independent of any effect due to light exposure (fig. 7). A difference in strain to break between aqueous light-bleached and dark-immersed papers was detectable in specimens exposed for only 2 hours; longer incubations in the Weather-ometer eliminated this light/dark difference. The role of sizing and temperature in these changes in tensile properties of the paper are considered in section 4. Humid oven aging had only a slight effect on the stress to break of the W56 papers, as indicated by there being only very minor changes in the shapes or initial slopes of the stress-strain curves. However, artificial aging did embrittle the paper (fig. 8; see also fig. 7). Maximum strain at breakage was reduced to 70–85% of that before aging. Again, aqueous light bleaching did not appear to have any worse effect on the embrittlement due to artificial aging than any of the control Weather-ometer conditions (e.g., fig. 8).
Fig. 7.
Nominal stress as a function of strain for W56 papers (MD) after 2 hours in the Weather-ometer under the conditions indicated. Curves are averages of two sets of triplicate measurements.
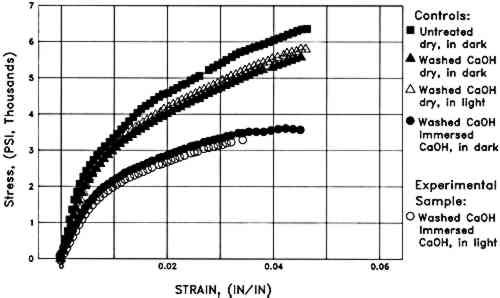 |
Fig. 8.
Nominal stress as a function of strain for W56 papers (MD) after the 2 hour Weather-ometer treatments indicated and humid oven aging as described in the text. Curves are averages of two sets of triplicate measurements.
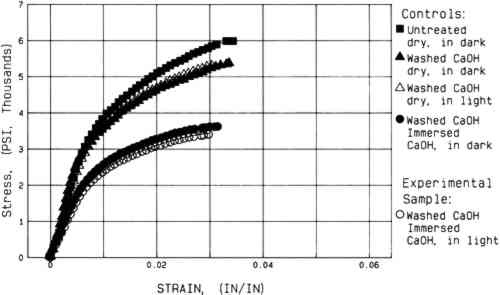 |
4 DISCUSSION
The results of this investigation demonstrate that aqueous light bleaching decreases discoloration immediately after treatment of both the sized and unsized papers. In addition, the extent of color reversion caused by humid oven aging of these papers is significantly less than that caused by aging controls that had been exposed to light while dry. After aging, the aqueously light-bleached W56 paper remained less discolored than the controls that were immersed and kept in the dark during Weather-ometer incubation.
The W56 cotton rag paper used in this study is a very strong paper that is also very deformable. Its strength is undoubtedly due in part to the presence of gelatin sizing.3 The disadvantageous effect of the aqueous light-bleaching treatment for the W56 paper was the marked decrease in its stress to break; however, similar decreases occurred in controls that were immersed in the dark during Weather-ometer incubation. This result suggests that the reduction in stress to break was caused by immersion of the paper for a second time and it was not directly owing to a photochemical reaction. The removal of some of the gelatin sizing could have contributed to this result; the paper lost some of its reinforcement with the size and also may have swelled as a result, causing an increase in cross-sectional area and a subsequent apparent decrease in stress to break. Two factors might have enhanced protein solubilization during Weather-ometer incubation. Concomitant loss of alum during the washing step may have softened the originally hardened gelatin, so that it was more easily dissolved out of the paper during the next immersion. Second, the unavoidably elevated temperatures in the Weather-ometer chamber (see appendix) would have increased protein solubility. Additional experiments to determine the effect of temperature on this process are under consideration. It should be noted that in an early study of aqueous light bleaching (Branchick et al. 1982), the samples that suffered the greatest loss of strength were 18th-century rag papers incubated at the highest temperature used in the investigation (40.5�C).
The reduced strain to break of the W56 papers that were immersed during Weather-ometer incubation may also be due in part to loss of gelatin size. The difference between the strain to break of the 2 hour light- and dark-immersed papers could be attributable to a temperature discrepancy, since the solution exposed to light may have heated up more rapidly than the solution kept dark. This difference becomes statistically insignificant with increase in immersion time (and increased solution temperature) and upon aging. It appears, however, that the initial effect of exposure to light during immersion and the effects of prolonged immersion may be comparable to the effect of artificial aging, exhibited by reduced strain to break. Because this similarity is not exhibited in the unsized paper, the possibility of interaction between light and size components (such as alum) must also be considered. Additional investigation of this effect is required before the phenomenon can be adequately explained.
The surface pH of the W56 paper was increased slightly by washing, but it fell back approximately to its original value upon artificial aging. The surface pH of the W1 paper was lowered slightly by the initial wash, and lowered much further by artificial aging. These observations suggest that the dilute Ca(OH)2 bath did not adequately neutralize acidic moieties in the paper and did not provide a buffer reserve that could successfully counteract acid groups formed during aging.4
The conditions to which the papers were exposed in the Weather-ometer were rather extreme. The W56 papers survived exposure to these conditions in surprisingly good shape. That the strong light itself—as distinct from immersion—was not responsible for significant deleterious changes in W56 papers that had been immersed is encouraging. Furthermore, no significant detrimental effect of light was discovered upon humid oven aging of the W56 papers immersed during Weather-ometer incubation. All papers suffered color reversion and embrittlement, but in no case was an aqueously light-bleached sample significantly more damaged by the artificial aging process than the corresponding control paper that was kept in the dark under otherwise identical conditions.
The results of this study indicate that aqueous light bleaching decreases the extent of discoloration on subsequent aging of the W56 paper, even for the shortest time of exposure under the present experimental conditions. The reduced color reversion in aqueously light bleached W56 samples might be partly attributed to removal of gelatin size, which could discolor upon artificial aging. However, this loss is unlikely to account for all of the decreased color reversion since the controls immersed in the dark lost about the same amount of gelatin as those that were aqueously light bleached, yet the former developed significantly more yellow chromophores during humid oven aging than did the latter samples.
It is interesting to note that the aqueously light-bleached W1 samples discolored the least after aging, discoloring less even than untreated papers. These results, for a paper composed solely of cellulose fibers, combined with the above results for sized W56, suggest not only that aqueous light bleaching removes chromophores from the paper directly but also that immersion during light exposure prevents colorless but potentially deleterious moieties from being present in the paper following that exposure. Whether this result is achieved by prevention of formation of the precursors that discolor upon aging or by removal of them into the immersion solution must be determined by more detailed chemical studies.
That sizing, and the chemical composition of the paper fibers themselves, will have a major effect on the response of any paper to every step of the aqueous light-bleaching process cannot be overemphasized. For example, the above results demonstrate that the effects of immersion on naturally aged, protein-sized papers must be carefully considered before the paper is washed, as well as reimmersed in preparation for the aqueous light-bleaching step (e.g. Burgess 1985). In general, a more complete basis for making the decision to use aqueous light-bleaching is required. More research must be performed with careful control of as many variables as possible. Not only must the effects of solution temperature, pH, and buffering agent be studied, but the composition of the paper and the nature of the chromophores responsible for the discoloration should also be considered (Lee et al. 1989a). These factors can be expected to have a major influence on the effectiveness of the treatment.
If loss of sizing is experimentally controlled, more subtle effects of light upon the mechanical properties of the paper may become evident. For documentation of these types of changes, chemical analyses and measurement of degree of polymerization are expected to be appropriate (Burgess 1985). It will also be desirable to use as a starting material a paper that is well defined chemically—that is, a new paper—and to discolor it by preaging it artificially under controlled conditions before subjecting it to further experimentation (Hofmann et al. 1991). Once the effects of the aqueous light-bleaching procedure in the long as well as the short term have been thoroughly documented, those papers for which it would be an efficacious conservation treatment can be identified, and appropriate treatment conditions can be determined.
ACKNOWLEDGEMENTS
We thank Lambertus van Zelst and Alan Postlethwaite for providing the opportunity to continue this research project at the Conservation Analytical Laboratory, Marion F. Mecklenburg for advice and discussion, and Melanie Feather for expert technical guidance in SEM/EDX data acquisition. This project could not have been completed in the limited time available without the assistance of paper conservation interns at the Conservation Analytical Laboratory, most notably Nancy McRaney and Danielle Nguyen. Emily Klayman, Crista Hofmann, Olga Souza, and Andrew Robb also participated. Terry Trosper Schaeffer thanks the Chemistry Department, California State University—Northridge, for the use of its Beckman DU-64 spectrophotometer, and the staff of the Conservation Analytical Laboratory for their cheerful hospitality.
APPENDIX
1 APPENDIX
1.1 PAPER PREPARATION
A 14� � 21 in sheet of paper was misted with deionized water and submerged in a bath of 21 liters of Ca(OH)2 solution, initial pH 9.5, prepared by dropwise addition of a filtered, saturated Ca(OH)2 solution to deionized water until the desired pH was reached. The reverse osmosis water purification system at CAL consistently provides water of resistivity ≥ 18 Mohm-cm. After 4 hours, the paper was removed from the bath on polyester webbing, blotted between blotters, and then placed between blotters in a felt and Plexiglas press under ca. 1 psi weight. The blotters were changed after 5, 20, 40, and 60 minutes. A final blotter change was made the next day. Two sheets of each paper were so washed. The pH of the bath at the end of the washing procedure was 6.5 for the W56 paper and 7.3 for the W1. Each sheet of paper remained in the press 2 weeks, when it was removed, covered with polyester webbing, and allowed to equilibrate in the laboratory atmosphere for 1 day before being cut into 4 � 4 � in specimens with the machine direction in the short dimension. Cotton threads were sewn into two corners about � in from the edges, so that each paper could be suspended vertically, with the machine direction horizontal.
Papers were suspended vertically in 600 ml polystyrene flat-sided culture bottles as described previously (van der Reyden et al. 1988), oriented so that the felt side of the paper would face the light source in the Weather-ometer. To each flask that contained a paper to be immersed during Weather-ometer incubation was added 580ml of a stock pH 9.5 Ca(OH)2 solution prepared as above. Enough stock solution was prepared immediately prior to loading the papers to be adequate for all immersed papers in an experiment.
2 AQUEOUS LIGHT-BLEACHING AND CONTROL TREATMENTS
Irradiance in the Weather-ometer chamber was maintained constant at 0.35 w/m2. Quartz tubes were used for the water jacket, which serves as a cooling filter for the xenon arc lamp. The walls of the polystyrene culture bottles filtered out most of the ultraviolet radiation (van der Reyden et al. 1988). The lamp power and the temperature in the Weather-ometer chamber were monitored throughout the experiment. The lamp power fluctuated between 7.1 and 7.4 kW during operation.
To prevent fogging by water condensation on the bottle surfaces, the Weather-ometer was operated without humidity in the chamber. Because the instrument has no refrigeration unit, it was not possible to keep the chamber temperature at room temperature while the xenon arc lamp was on. Dry bulb temperature in the chamber rose to 39 � 1�C within a few minutes of starting the lamp and stayed in that range throughout the experiment. Immediately upon removal from the chamber, the temperature of the solutions in the bottles wrapped in foil were about 1�C above the temperature of the chamber. The temperature of the solutions in bottles exposed to light was 47–48�C. The temperature inside the “dry” control bottles could not be measured accurately. It is assumed to have been approximately the same as the chamber temperature.
The pH of the immersion solutions was measured when the solutions had cooled to approximately room temperature. As expected, the pH of all immersion solutions fell during incubations in the Weather-ometer. One W1 immersion solution and two W56 immersion solutions were assayed by a modified Lowry procedure to determine if protein had been solubilized from the W56 paper.
The dry condition controls were placed in archival polyester sleeves immediately upon removal from the Weather-ometer and stored in a binder. As each immersed paper was removed from its bottle, it was placed on a thick blotter and turned over after about 1 minute. These papers were then placed individually between 7 � 9 in blotters in a Plexiglas press and weighted under less than 1 psi. The blotters were changed twice at 10 minute intervals. The wet papers were then placed between blotters and felts in a larger Plexiglas sandwich and more heavily weighted overnight (less than 1 psi). The blotters were changed once the next day, and these papers remained in the press for 2 weeks, after which the dry papers were transferred to polyester webbing, covered with a fresh blotter, and allowed to equilibrate with the laboratory atmosphere for 1 day.
3 ARTIFICIAL AGING
All papers that had been in the Weather-ometer were cut in half along the machine direction, with a fresh scalpel blade, to give two pieces 4 � 2 � in. The half of each paper that was to be artificially aged was sewn with cotton thread into a Plexiglas frame. The halves were positioned with the machine direction vertical and all four corners anchored, so that no papers were touching. The papers could not rotate or swing in the frames to come into contact with each other; however, they did vibrate in the oven draft.
At the end of the aging period, the papers were equilibrated overnight, still in the Plexiglas frame, in the dark at room temperature. After being cut out of the frames, they were stored in polyester film enclosures.
4 ANALYTICAL PROCEDURES.
The following tests were applied:
- Spot tests. The spot test for aluminum and the ninhydrin spot test for protein size were performed according to Browning (1977) on small pieces of selected specimens of W56 paper. Papers known to contain these sizes and W1 filter paper were used as positive and negative controls, respectively.
- SEM/EDX. Thin strips of papers to be examined by SEM/EDX were mounted on aluminum stubs and coated with carbon. The JEOL JXA-840A scanning electron microscope with a Tracor Northern TN5502 energy-dispersive x-ray analysis accessory was used to obtain representative micrographs of fibers and for elemental analysis. Dot map representations of Al distributions were recorded where appropriate.
- FTIR. Both reflectance and transmittance infrared spectra of some papers were recorded using a Mattson Cygnus 100 Fourier Transform Infrared Spectrometer with a Spectratech IR-Plan microscope accessory. These spectra were compared with those of hide glue and of cellulose standards.
- Protein measurement. Protein content of some 24 hour immersion solutions was measured using the procedure of Lowry et al. (1951), slightly modified. Gelatin (Knox), rather than the usual bovine serum albumin, was used as a standard. To 0.1 ml of immersion solution, or solution of gelatin standard, in a test tube, was added 0.25 ml of 1N NaOH. After incubation in hot tap water for 30 minutes, 2.5 ml of a freshly prepared mixture of: [100 ml 2% (w/v) NaCO3, 2 ml 1% Na(or K)tartrate, and 2 ml 0.5% anhydrous CuSO4] was added. The mixture was stirred vigorously. Ten minutes later, 0.1 ml of Folin reagent (Sigma Chemical Co.) was added, and the reaction mixture was immediately stirred vigorously. After 45 minutes, the extent of blue color development was determined by measuring the absorbance at 740 nm on a Beckman DU-64 spectrophotometer. A series of gelatin standard solutions of different concentrations were assayed with each set of samples.
- Surface pH was measured with an Orion #81-35 flat surface Ross-style combination electrode and Corning model 12 pH meter. The well-rinsed electrode, with a pendent drop of laboratory deionized water, was lowered onto a � inch square paper specimen resting on a polyethylene bag padded with blotters. The pH was recorded after 5 minutes. The electrode was calibrated with pH 7 and pH 4 buffers before each measurement session, and calibration was checked at the end of the session. As expected for this very stable electrode, calibration did not change detectably during any of the 2–3 hour periods over which measurements were made.
- Colorimetry. Paper color was quantitated with a HunterLab Ultrascan Spectro-colorimeter. Reflectance spectra were recorded at 10 nm intervals from 375 to 750 nm, using the visible light source, at three different locations on each side of each paper specimen. The instrument was set for a 10 degree observer, the small (� in diameter) area of view was used, and specular reflectance was included. The triplicate readings were averaged. CIE La∗a∗b∗ values and delta E∗ were computed automatically from these data.5 In most cases, values for recto and verso sides of each specimen, and of the papers treated identically in the two experiments, did not differ significantly from each other. Thus, the values were averaged, and standard deviations are reported above.
- Tensile measurements. Tensile properties of all papers were investigated using Mecklenburg relaxation tensometers6(Mecklenburg 1984), with a horizontal load applied in the machine direction to the paper strips. Narrow strips of uniform width were cut with a Dahle mat cutter. After measurement of paper thickness in five places with a micrometer, the paper strips were mounted horizontally in the apparatus exposed to laboratory atmosphere. Care was taken not to subject the papers to excess moisture as they were being mounted in the apparatus. Over the several months that tensile measurements were performed, the laboratory temperature fluctuated no more than 1.7�C and the relative humidity no more than 2%. After an initial equilibration period of 5–15 minutes at a gauge length of 2.5 in, the strip was stretched 2.5 � 10−3 in, and 1 minute later the stress sustained by the paper strip was recorded. This process was repeated once per minute until the paper strip broke. Measurements were made on three strips of each paper. From these data, nominal stress (force applied per nominal cross-sectional area of the strip) and strain (change in length divided by gauge length) were computed and corrected for bending of the strain gauge. Nominal stress was plotted as a function of strain for each paper strip. In the figures presented above, averages of the curves obtained from the measurements on the triplicate papers are shown. Where error bars do not appear, they would be no larger than the symbols used on the graphs. In almost all cases reproducibility was very good both among the triplicates for each paper in an experiment and between the two different experiments performed (tables 2 and 4).
NOTES
1. Dr. David Martin of Whatman Paper, Ltd., London, advised us that Whatman records showed that the paper was made of 100% cotton rag with alum, gelatin, and soap flakes added. Further details of the manufacture of this paper are no longer available.
2. A major advantage of collecting spectral data is that any of the color measurement scales can be used to describe the results. CIE L∗a∗b∗ is considered to be one of the more useful and sensitive scales; however, many researchers use % reflectance or K/S values at certain wavelengths. These data can be taken from the tabulated spectral data recorded on the HunterLab instrument. For example, for measurements of some color standards, the following results were obtained:
The wavelength used for % reflectance and K/S was 457 (TAPPI Standard 452 os-77). In addition to its sensitivity in making color changes more evident, the spectral data give a complete picture of what is happening to the specimen. For example, the curves for the standard tiles are very similar at the 457 nm area, but the orange tile spectrum shows peaks at 510 and 620 nm, while the yellow-green tile spectrum shows peaks at 525, 600, and 675 nm.
3. Application of animal glue has also been shown to increase the strength of Japanese papers; for some of these papers this strength advantage is maintained upon artificial aging (Inaba and Sugisita 1986).
4. Although it provides a solution with greater neutralizing capacity, the common procedure of diluting a saturated Ca(OH)2 solution 1:1 with water was avoided because it can yield a solution of pH 12 initially and because it does not provide a reproducibly reliable buffer reserve. It is also likely to result in precipitation of CaCO3 during the 24 hour incubation, as far more CO2 is absorbed from the air.
5. Readers interested in more complete reflectance data or in brightness values can obtain these by contacting the authors at the Conservation Analytical Laboratory.
6. Details of the construction and use of these instruments may be obtained from the Conservation Analytical Laboratory.
REFERENCES
Baker, C.1986. The doublesided light bleaching bank. American Institute for Conservation Book and Paper Group Annual4:88–91.
Branchick, T. J., K. M.Keyes, and F. C.Tahk. 1982. A study of the bleaching of naturally aged paper by artificial and natural light. AIC preprints, 10th Annual Meeting, American Institute for Conservation, Washington, D.C.29–39.
Browning, B. L.1977. Analysis of paper, 2d ed.New York: Marcel Dekker. Chaps. 8–10.
Burgess, H.D.1980. The colour reversion of paper after bleaching. In: Conservation of Library and Archive Materials and the Graphic Arts, ed.G.Petherbridge. London: Butterworths. 57–70.
Burgess, H. D.1985. Gel permeation chromatography: Use in estimating the effect of water washing on the long-term stability of cellulosic fibers. In Historic textile and paper materials: Conservation and characterization, ed.H. L.Needles and S. H.Zeronian. Advances in Chemistry series 212, Washington, D.C.: American Chemical Society. 363–76.
Burgess, H. D.1988. Practical considerations for conservation bleaching. Journal of the International Institute for Conservation–Canadian Group13:11–26.
Eldridge, B. P.1982. A sun bleaching project. American Institute for Conservation Book and Paper Group postprints. 1:52–55.
Erhardt, D., D.vonEndt, and W.Hopwood. 1987. The comparison of accelerated aging conditions through the analysis of extracts of artificially aged paper. AIC preprints, 15th Annual Meeting, American Institute for Conservation, Washington, D.C.43–55.
Feller, R.L., S. B.Lee, and J.Bogaard. 1982. The darkening and bleaching of paper by various wavelengths in the visible and ultraviolet. American Institute for Conservation Book and Paper Group postprints. 1:65–83.
Flieder, F., M., Leroy, J. C.Andreoli, and F.Leclerc. 1991. Comparative study of four paper bleaching methods. Presented at the International Federation of Library Associations International Preservation and Conservation Research Seminar, New York.
Hofmann, C., D.van derReyden, and M.Baker. 1991. Comparison and evaluation of bleaching procedures: The effect of five bleaching methods on the optical and mechanical properties of new and aged cotton linter paper before and after accelerated aging. American Institute for Conservation Book and Paper Group Annual10: 109–27.
Inaba, M., and R.Sugisita. 1986. Effect of Doza on the deterioration of Washi (Japanese paper). Scientific Papers on Japanese Antiquities and Art Crafts31:32–40.
Keyes, K. M.1980. Alternatives to conventional methods of reducing discoloration in works of art on paper. In Conservation of Library and Archive Materials and the Graphic Arts, ed.G.Petherbridge. London: Butterworths. 49–55.
Lee, S. B., J.Bogaard, and R. L.Feller. 1989a. Darkening of paper following exposure to visible and near-ultraviolet radiation. Journal of the American Institute for Conservation28:1–18.
Lee, S. B., J.Bogaard, and R. L.Feller. 1989b. Damaging effects of visible and near-ultraviolet radiation on paper. In Historic textile and paper materials II: Conservation and characterization, ed.S. H.Zeronian and H. L.Needles. Advances in Chemistry series 410.Washington, D.C.: American Chemical Society. 54–62.
Lee, S. B., J.Bogaard, and R. L.Feller. n.d. Bleaching by light, I: Effect of pH on the bleaching or darkening of papers in the dry and in the immersed condition under visible and near-ultraviolet radiation. In Symposium 88: The conservation of historic and artistic works on paper. Ottawa: Canadian Conservation Institute. Forthcoming.
LePage, M., and J.Perron. 1986. Investigation of some aspects of the light bleaching of paper. Conservation Training Programs, 11th Annual Conference, University of Delaware, Winterthur, Del., 54–68.
Lienardy, A., and P.vanDamme. 1989. R�sultats de recherches exp�rimentales sur le blanchiment du papier. Studies in Conservation34:123–36.
Lowry, O. H., N. J.Rosebrough, A. L.Farr, and R. J.Randall. 1951. Protein measurement with the Folin phenol reagent. Journal of Biological Chemistry193:265–75.
Mecklenburg, M. F.1984. The role of water on the strength of polymers and adhesives. Ph.D. diss., University of Maryland, College Park, Md.
Phillips, G. O.1985. The effects of light on cellulosic systems. In Cellulose and its derivatives, ed.J. F.Kennedy et al. Chichester, England: Ellis Horwood. 119–34.
Savard, G.1986. An investigation of whitening and colour reversion effects on light bleached, artificially aged paper. Conservation Training Programs, 12th Annual Conference, New York University and Columbia University, New York. 57–83.
Schaeffer, T. T., M. T.Baker, and D.van derReyden. 1991. Effect of aging on an aqueously light-bleached, mixed pulp paper. American Institute for Conservation Book and Paper Group Annual10:205–15.
van derReyden, D.1981. Wax pick testing: A preliminary study. Art Conservation Training Programs, 8th Annual Conference, New York University, New York. 62–73.
van derReyden, D., M.Mecklenburg, M.Baker, and M.Hamill. 1988. Update on current research into aqueous light bleaching at the Conservation Analytical Laboratory. American Institute for Conservation Book and Paper Group annual7:73–106.
AUTHOR INFORMATION
TERRY TROSPER SCHAEFFER received a B.A. in physics and biophysics (1961) and a Ph.D. in biophysics (1967) at University of California, Berkeley. Most of her research has been in the areas of interaction of photosynthetic pigments with other plant and bacterial membrane components, and ion transport across mammalian cell membranes. In 1989–90, she entered the field of conservation research when she was a Fellow-by-Courtesy, G.W.C. Whiting School of Engineering, Johns Hopkins University, and a Visiting Research Collaborator, Conservation Analytical Laboratory. She is a fellow in Paper Conservation Reseach at the Conservation Center of the Los Angeles County Museum of Art. Address: Conservation Center, Los Angeles County Museum of Art, 5905 Wilshire Blvd., Los Angeles, Calif. 90036.
MARY T. BAKER received her B.S. in chemistry in 1980 and her Ph.D. in 1986 in materials science with a specialty in polymer science from the Institute of Materials Science at the University of Connecticut. She has worked at the Conservation Analytical Laboratory, Smithsonian Institution, as a research chemist since 1987, collaborating with conservators on projects such as the effects of fumigation on materials; treatment and characterization of coated papers; light bleaching of paper; and methods development for analysis of microsamples of paints, varnishes, and other materials. Her current research is on the modern polymeric materials in air and space artifacts, their aging mechanisms, storage, treatment, and display. Address: Conservation Analytical Laboratory, Museum Support Center, Smithsonian Institution, Washington, D.C. 20560.
VICTORIA BLYTH-HILL is the senior paper conservator in the Conservation Center of the Los Angeles County Museum of Art. She is past chair of the Book and Paper Group of the AIC and has been a Fellow of that organization since 1988. She has presented lectures at AIC and other professional organizations and at universities on subjects ranging from general conservation awareness to the treatment of the Leonardo da Vinci “Codex Hammer.” Blyth-Hill has supervised many interns over the years at LACMA and has sponsored such research projects as accelerated aging of adhesives and pigment analyses on Persian miniatures. Address: Conservation Center, Los Angeles County Museum of Art, 5905 Wilshire Blvd., Los Angeles, Calif. 90036.
DIANNE VAN DER REYDEN received certificates in conservation from Harvard University Art Museums (1981) and the Conservation Center, Institute of Fine Arts, New York University (1980), along with an M.A. in art history (1979), serving internships at the Fogg Art Museum, the Library of Congress, and the Museum of Modern Art. She is senior paper conservator and co-head of the Paper Conservation Laboratory at the Conservation Analytical Laboratory, Smithsonian Institution, engaged in research in aqueous light bleaching and the effects of solvents on specialty papers and in the training of interns and professionals. She recently served as secretary of AIC and has been a Fellow for several years. Address: Conservation Analytical Laboratory, Museum Support Center, Smithsonian Institution, Washington, D.C. 20560.
Section Index |