A STUDY OF THE REMOVAL AND PREVENTION OF FUNGAL STAINS ON PAPER
HANNA SZCZEPANOWSKA, & CHARLES M. LOVETT
ABSTRACT—A method for the rapid production of specific fungal stains on paper is described and it is shown that the stained paper can be used for studying practicable approaches to the removal and prevention of fungal stains on paper artworks. The fungal species chosen for this investigation are frequently found on paper and produce characteristic stains: Alternaria solani (black stains), Penicillium notatum (yellow-green stains), Fusarium oxysporum (purple-pink stains), and Chaetomium globosum (yellow-grayish-brown stains). The effects of solvent treatment and laser irradiation on stains produced by the individual fungi are as follows: Exposure to 1,4-dioxane for 24 hours completely removes the stain produced by F. oxysporum and significantly reduces stains produced by P. notatum and C. globosum. Exposure to N,N-dimethyl-formamide for 24 hours significantly reduces the stains produced by C. globosum and P. notatum and slightly reduces the stain produced by F. oxysporum. Exposure to pyridine for 24 hours partially removes the stains produced by C. globosum and F. oxysporum.
1 INTRODUCTION
Fungi growing on paper art objects affect the surface characteristics of the artwork primarily by producing, and often secreting, a variety of pigments. Surface characteristics will also be affected to varying degrees due to the fungal digestion of the cellulose fibers. Fungal pigments are typically composed of many complex chemical substances that are formed during metabolic processes; these pigments may be encrusted in spores, present in mycelium (the mass of filaments constituting the body of the fungus), or secreted to a substrate such as paper. An example of a paper artwork, an early 19th-century map that was damaged by fungal growth, is shown in figure 1. The section of the map depicted exemplifies all types of damage that can be caused by fungi: staining, degradation of cellulose fibers (resulting in tears), and residue embedded in the paper. While fungal damage presents a very serious problem to paper conservators, there are presently no effective methods for local, nonaqueous removal of fungal stains from paper, and there are no definite storage guidelines for the protection of paper artworks against fungal damage.
Fig. 1.
Section of an early 19th-century map damaged by fungal growth
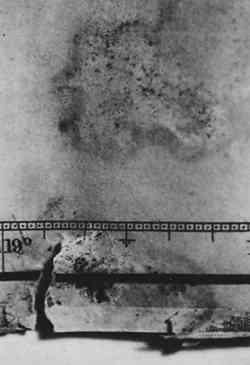 |
This study addresses a fundamental problem that faces paper conservators: the need for a repertoire of practicable methods for removing fungal stains from paper (Szczepanowska 1986; Sczepanowska and Lovett 1988). Because the stains produced by different fungi are chemically distinct and therefore respond differently to any given treatment, it is important to define removal methods for stains produced by individual fungi. With this in mind, we developed a simple methodology for studying the removal of stains produced by specific fungi. Briefly, this methodology involves growing a known fungal strain aseptically under conditions that result in intense paper staining in a few days. The paper thus stained can be subjected to removal techniques systematically in order to identify effective techniques for a particular fungal stain. Clearly, it would be desirable to have a catalog of stain removal techniques, classified according to individual fungi, so that a conservator could use the appropriate method for an identified fungal strain. We describe a means toward such a classification that obviates experimenting on artworks themselves.
Our investigation focused on four fungal species that are frequently found on artworks in this climate and are responsible for the most prominent stains: Fusarium oxysporum, Alternaria solani, Penicillium notatum, and Chaetomium globosum(Strzelczyk 1981). These fungi were selected on the basis of data collected by Szczepanowska during 14 years of restoring artworks infested by fungi. It should be noted that these are not the only fungi that infest paper artworks and the methods we describe could be applied to the growth and analysis of stains produced by any known fungal strain.
We explored the effect of various solvents in removal of fungal stains. This method provides a potentially quick and effective means for paper conservators to treat artworks discolored by fungal pigments. Although we have by no means exhausted potential solvents, our results suggest possible approaches to the conservation of paper artworks damaged by these particular fungi. Not surprisingly, we found that the individual fungal stains respond differently to various treatments thus demonstrating the need for stain-specific removal techniques. The search for such removal techniques should be facilitated by the rapid growth and staining method decribed in this article.
The ability to grow a particular fungi rapidly under conditions that result in stain production also affords a way to assess the effects of certain environmental conditions on fungal growth and stain production. Using our method of fungal growth under aseptic conditions we can easily alter parameters such as pH, temperature, and light exposure—factors that are known to affect fungal growth (Wolf and Wolf 1947). We report here the effects of these factors, under our growth conditions, on both fungal growth and stain production. Our results are consistent with previous studies showing that varying these parameters affects growth rate and pigment production for a variety of fungal strains (Hulme and Stranks 1976; Linfield 1986; Nol et al. 1983; Verona 1969; Wolf and Wolf 1947). This type of analysis should help to determine appropriate stronge conditions that would minimize fungal destruction of paper artworks. Unfortunately, it is not possible to examine the effects of humidity (probably the single most important factor affecting fungal growth) using our growth procedure; fungi are grown in covered Petri dishes, containing agar, where the humidity is expected to be very high (nearly 100% RH while the agar is wet).
2 MATERIALS AND METHODS
2.1 FUNGAL STRAINS AND MEDIA
Strains of the fungal species Fusarium oxysporum, Alternaria solani, Penicillium notatum, and Chaetomium globosum were obtained from Carolina Biological Supply. F. oxysporum, A. solani, and P. notatum were grown and maintained on either potato dextrose agar or Sabouraud dextrose agar (Difco Laboratories). C. globosum was grown and maintained on V-8 juice agar as recommended by Carolina Biological Supply. Artist's watercolor, laid, medium-weight paper (Saunders Company), readily available at art supply stores, was chosen because it represents features characteristic of paper supports encountered during restoration of paper artworks infested by fungi. It is medium-weight, pliable, rag paper that does not exhibit the application of heavy sizing.
2.2 FUNGAL STAINING OF PAPER
Fungi were cultured on agar plates containing sterilized artist's watercolor paper placed on the agar surface as follows: Sabouraud dextrose agar was rehydrated by suspending 65 g of agar in 1 liter of deionized water. The medium was sterilized by autoclaving for 15 minutes (15 lbs pressure and 121�C). The sterile medium was allowed to cool to 55�C and then poured into approximately 20 100 � 15 mm sterile Petri dishes. Covered agar plates were stored at 4�C until ready for use. Paper cut into 2 in squares and autoclaved for 15 minutes was placed on the agar surface, and either F. oxysporum, P. notatum, or A. solani was aseptically removed from a culture stock and streaked along the interface of the paper and the agar and grown under different conditions (as indicated in text) until staining of paper edges was pronounced. C. globosum was similarly streaked on V-8 juice agar plates containing sterile paper. V-8 juice agar was prepared by adding 200 ml V-8 juice, 3.0 g calcium carbonate, and 20 g Bacto-agar (Difco Laboaraties) to 1 liter deionized water. The medium was autoclaved and poured into plates as described above. It is essential that streaking of fungal strains be done quickly to minimize the length of time that plates are uncovered and exposed to potential contaminants.
2.3 EFFECTS OF LIGHT, TEMPERATURE, AND pH ON FUNGAL GROWTH AND PIGMENT PRODUCTION
Fungi were incubated in a constant temperature growth chamber (4�, 25�, or 37�C), equipped with a timer-controlled fluorescent light source, for several days as indicated. Temperature and light exposure were varied as descibed in the text. The pH of the agar media was varied from 5.0 to 9.0 by the addition of hydrochloric acid or sodium hydroxide before autoclaving. Unless otherwise indicated, strains were grown on media at pH 5.6. The extent of growth and pigment production was evaluated qualitatively by visual inspection, and photographic records were maintained.
2.4 TREATMENT OF STAINED PAPER WITH ORGANIC SOLVENTS
Artists' watercolor paper was stained by fungi during growth over several days (as described above), and fungi were removed by scraping with a scalpel. The stained paper was placed on a porcelain Hirsch funnel, and approximately 5 ml of solvent was applied with a Pasteur pipet. The filtrate was collected. Alternatively, the stained paper was soaked in each solvent for a period of 24 hours prior to filtration. The ability of each solvent to remove stains was assessed in three ways: (1) visual inspection of the paper after treatment; (2) comparison of photographic records from both before and after treatment; and (3) analysis of ultraviolet visible spectra of filtrates.
The solvents used here are hazardous substances that produce harmful vapors and are irritating to the skin. All solvent work was conducted in a ventilated hood.
2.5 SCANNING ELECTRON MICROSCOPY
Paper samples, cut in approximately 0.5 cm squares, were attached to mounting stubs with double-backed tape and sputter coated with gold. Samples were scanned at the indicated magnifications on a Cambridge Stereoscan 100 scanning electron microscope.
3 RESULTS AND DISCUSSION
3.1 FUNGAL GROWTH AND STAIN PRODUCTION
The four fungal strains were individually streaked onto sterile agar plates at pH 5.6, as described in section 2.2, and incubated at 25�C. (A pH of 5.6 was used because this is the pH of the medium when prepared as described.) After 3 days of incubation at constant temperature and exposure to fluorescent light for 8 hours a day, all fungi had grown significantly both on the agar and the paper, covering an area of several square cm. Removal of the fungus by scraping with a scalpel revealed significant staining of the paper by each fungus (fig. 2). From the homogeneous appearance of the fungi as well as their characteristic stains (see below), we ascertained that the individual fungi were not contaminated by other micro-organisms. Thus, growing the fungi in this manner provides a rapid means of obtaining paper stained by a specific fungus.
Fig. 2.
Stains produced by Chaetomium globosum, Fusarium oxysporum, Alternaria solani, and Penicillium notatum
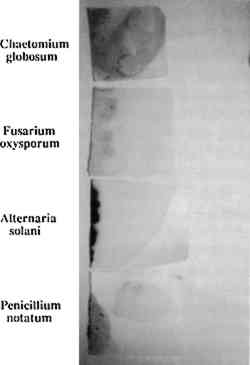 |
Growth on enriched agar medium provides both essential nutrients and water needed for rapid fungal growth. However, this medium also supports the growth of many other micro-organisms that grow faster than the fungi described here. It is therefore critical that the agar and paper have been sterilized and that the fungi are introduced to the agar aseptically.
All four fungal species produce characteristic pigments that stain paper or any medium on which they are grown. Many of these fungal pigments have been isolated, and in most cases they contain a complex mixture of chemicals that strongly absorb visible and ultraviolet light. The stains produced on paper by the four fungi (see fig. 2) are consistent with previous reports of pigments from these fungal species. Penicillium notatum produces a yellow-green stain on paper under our growth conditions consistent with the yellow compounds secreted by this species (Rothe 1950; Wolf and Wolf 1947). The stain apparently penetrates the paper, because its intensity is the same on both the back and front of the paper. We subsequently found that the isolated stain, extracted from the growth medium, is bright yellow (Szczepanowska and Lovett, unpublished). The greenish appearance of the fungi corresponds to the color of the spores, which are embedded on the paper surface and difficult to remove mechanically. Alternaria solani produces a pronounced black stain that adheres very strongly to the paper, consistent with reports of black pigments produced by this fungi (Stoessl 1969; Zaprometova et al. 1971). In fact, it is not possible to mechanically remove the fungus from the paper, and we cannot determine whether the stain is secreted on to the paper or simply a pigment contained within the fungus itself. There is evidence that the pigment produced by A. solani is not secreted by the fungus (Zaprometova et al. 1971). Fusarium oxysporum produces a purple-pink stain that remains on the paper after the fungus is mechanically removed. This stain could be due, in part, to the presence of fusarubin, a red pigment secreted by Fusaria(Ruelius and Gauhe 1950). The stain appears to penetrate the paper, although the intensity on the surface in direct contact with the fungus seems to be greater. Chaetomium globosum produces a yellow stain after about 3 days; the stain becomes grayish-brown in another 2 days. This stain could be due, in part, to the presence of tomichaedin, a yellow pigment extracted from the mycelium of Chaetomium species (Itabashi et al. 1955).
Scanning electron microscopy affords a relatively simple way to examine the effects of fungal growth and stain production on the paper at a microscopic level. Figures 3–7 show scanning electron micrographs of unstained paper and paper samples stained by the different fungi. Fungal debri, including mycelium and spores, are evident on paper stained by a fungal species. Although all four species produce enzymes that digest cellulose (Agrawal et al. 1963; Peterson 1963; Rautela and Cowling 1966), comparison of unstained paper with stained paper suggests that in most cases the destruction of paper fibers is not severe (see figs. 3–6). It is likely, however, that under our growth conditions, the components of the medium are preferred sources of nutrients; this would not be the case when fungi infest paper artworks.
Fig. 3.
Scanning electron micrographs of unstained paper. (Photo is 60% of original size.)
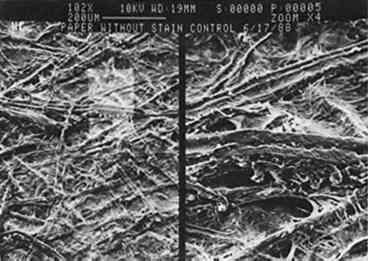 |
Fig. 4.
Scanning electron micrographs of paper stained by Alternaria solani. (Photo is 60% of original size.)
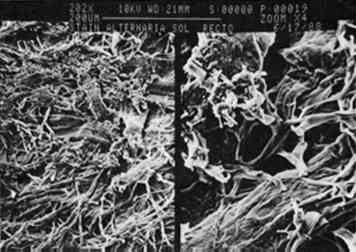 |
Fig. 5.
Scanning electron micrograph of paper stained by Fusarium oxysporum (Photo is 60% of original size.)
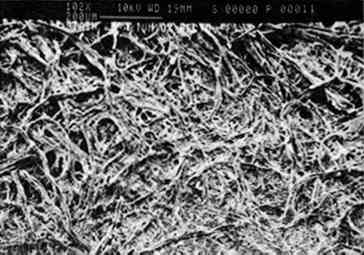 |
Fig. 6.
Scanning electron micrographs of paper stained by Penicillium notatum. (Photo is 60% of original size.)
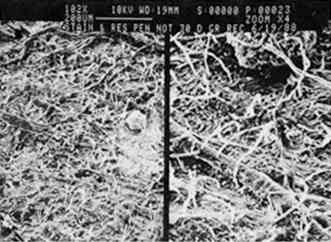 |
Fig. 7.
Scanning electron micrograph of paper stains digested by Chaetomium globosum. (Photo is 60% of original size.)
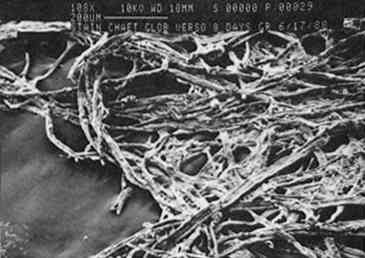 |
The exception is paper stained by C. globosum; after 8 days of growth on paper, C. globosum has apparently caused extensive digestion of the paper matrix (see fig. 7). After 30 days the paper was completely digested by this highly cellulolytic fungus.
3.2 EFFECT OF pH ON GROWTH AND STAIN PRODUCTION
A wide body of evidence indicates that optimum fungal growth occurs in acidic media; however, the range of pH that will permit growth varies with the species and the composition of the culture medium (Wolf and Wolf 1947). The pH dependence of pigment production probably depends on a variety of metabolic effects as well as the acid-base-indicator properties of fungal pigments (i.e., their ability to exhibit color changes at different pH values). An understanding of the pH dependence of fungal growth and stain production could suggest possible methods of paper treatment that would preclude fungal growth and stain production.
The four fungal species were grown on paper equilibrated with medium at pH values ranging from 5 to 8; growth and stain produced to the paper were examined, and the results are summarized in table 1. Although the extents of growth and stain production were not rigorously quantified, the differences observed were sufficiently distinct that trends can be clearly seen through a qualitative assessment. Both growth and stain production were assessed relative to the pH 5.6 results described above and divided into three categories: (1) maximum growth-staining (++) corresponding to levels visually indistinguishable from the pH 5.6 results; (2) moderate growth-staining (+) corresponding to levels that were clearly less than half those observed at pH 5.6; and (3) the absence of visually detectable growth or stain production. There was no measurable change in pH of the medium during the time the fungi were growing. This is not surprising because the enriched medium is expected to contain a high concentration of substances that can act as buffers.
TABLE 1 EFFECTS OF PH ON GROWTH RATE AND STAIN PRODUCTION
All species except C. globosum showed a strong dependence of stain production on pH, regardless of growth rate; in every case stains were most intense at pH 5. P. notatum exhibited no detectable difference in growth rate over the entire pH range; however, stain production showed a definite pH dependence. Stain production was most intense at pH 5; a bright yellow stain was visible on the verso surface after 4 days of growth. By contrast, at pH 8 there was no color produced, and the fungus itself was white and had a leathery texture. A. solani showed a marked pH dependence for both growth rate and stain production; at pH 5 a very intense black color was visible on the verso surface, whereas no color was apparent on the substrate at higher pH values. At pH 8 there was no detectable growth of A. solani after four days. F. oxysporum showed similar growth rates over the entire pH range. A purple stain was secreted to the paper when it was grown at pH 5 and pH 6, but the stain was more intense at pH 5. Very little stain was secreted to the paper at pH 7 and pH 8; however, at pH 8 the mycelium were more intensely purple than at the lower pH values. C. globosum did not show any pH dependence of either growth rate or stain production; growth and stain production were comparable at all pH values tested.
3.3 EFFECT OF TEMPERATURE ON GROWTH AND STAIN PRODUCTION
The temperature dependence of fungal growth has been well documented (Wolf and Wolf 1947). Studies of a wide variety of fungal species indicate that for each fungus there exists a minimum, a maximum, and an optimum growth temperature, the so-called cardinal temperatures. The first two of these correspond to temperatures that inhibit fungal growth, while the latter is the temperature that presumably encourages maximum metabolic activity. Most of the fungal species that have been studied have minimum temperatures between 3�C and 5�C, maximum temperatures between 30�C and 37�C, and optimum temperatures between 22�C and 29�C.
Based on these reported temperature ranges, we studied the effect of temperature on growth as well as stain production for each of the four fungal species (table 2). As described above, a qualitative assessment of the differences in growth rate and stain production was deemed sufficient. We chose three temperatures representative of the three cardinal temperatures: 4�C, 25�C, and 37�C. After several months there was no evidence of growth for any of the strains at 4�C. Surprisingly, C. globosum grew rapidly at 37�C, and stains were apparent after the second day; in fact, growth and stain production by this fungus were greatest at 37�C. In contrast, this temperature completely inhibited the growth of P. notatum and markedly reduced the growth rates of F. oxysporum and A. solani. The optimum temperature for both growth and stain production by these three fungi was 25�C. At 37�C F. oxysporum grew slowly on culture media as well as on paper but only produced stain to the medium, not on the paper. On the other hand, A. solani did not grow on paper at 37�C but grew slowly and produced stain on culture media.
TABLE 2 EFFECTS OF TEMPERATURE ON GROWTH RATE AND STAIN PRODUCTION
Our results for F. oxysporum are consistent with previous studies (Hulme and Stranks 1976; Linfield 1986). However, our results disagree with those of Hulme and Stranks (1976) for C. globosum, for which they reported an optimal temperature of 25�C, this disagreement may be due to differences in growth medium and light conditions.
Overall, the results are not surprising with the exception of C. globosum. Unlike the pH dependence results described above, both growth rate and stain production showed essentially the same temperature dependence.
3.4 EFFECT OF LIGHT ON GROWTH AND STAIN PRODUCTION
The effects of radiation on fungal growth are widely varied and depend on both the wavelength of radiation and the fungal species (Wolf and Wolf 1947). For example, in some cases UV and visible light have stimulatory effects on fungal growth, while in other cases the effects are inhibitory. The results reported here deal only with varying exposure to fluorescent light (0, 8, or 16 hours per day) at 25�C. For all four fungal species growth was better with exposure to light, although there was no detectable difference in growth when light exposure was increased from 8 hours per day to 16 hours per day. There is no apparent effect of light exposure on stain production by A. solani, C. globosum, or P. notatum; however, stain production by F. oxysporum showed a marked dependence on light. Figures 8 and 9 show F. oxysporum grown for 5 days either in the dark or exposed to light for 16 hours per day. When grown in the absence of light, there is a reduction in the amount of pinkish orange pigment present in the mycelium that is obvious from the top view (see fig. 8). Moreover, growing the fungus in the dark results in a significant decrease in the amount of pigment secreted to the medium, as seen by viewing the bottom of the culture plate (see fig. 9).
Fig. 8.
Growth of Fusarium oxysporum in the absence of light (left) and as exposed to light for 16 hours per day (right) as viewed from the top of culture plate
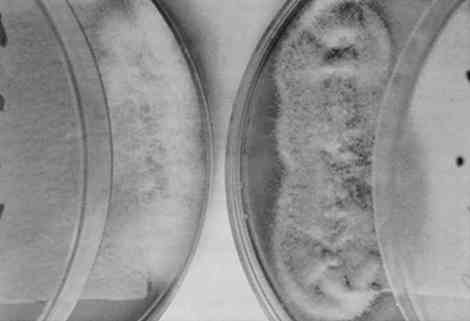 |
Fig. 9.
Growth of Fusarium oxysporum in the absence of light (left) and as exposed to light for 16 hours per day (right) as viewed from the bottom of culture plate
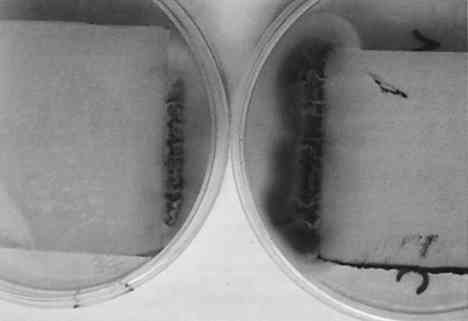 |
3.5 TREATMENT OF FUNGAL STAINS WITH ORGANIC SOLVENTS
Several components of the pigments from the four fungal species have been isolated and characterized. Xanthocillin and Citronen are yellow-colored antibiotics produced by P. notatum, which are probably responsible for some of staining by this fungus (Rothe 1950; Wolf and Wolf 1947). (Xanthocillin is actually a complex that consists of at least three distinct components, xanthocillins X, Y1, and Y2). Chaetomidin, a pigment extracted from the mycelium of Chaetomium species, was found to be identical to the bright yellow dye, oosprein, originally isolated from Oospora colorans(Itabashi et al. 1955). Fusarium species produce the red pigment, fusarubin (Ruelius and Gauhe 1950). Intracellular black pigments, which are not secreted under laboratory conditions, have been isolated from Alternaria species (Stoessl 1969; Zaprometova et al. 1971). Preliminary characterizations of these pigments suggest a resemblance to humic acids and melanins (Stoessl 1969). Pigments isolated from the mycelium of A. solani were shown to contain a mixture of anthraquinones and have been named altersolanols A and B (Zaprometova et al. 1971).
Based on the general solubilities of these and other dyes, we tested the stain-removing abilities of the following solvents on stains produced by each of the four fungal species: dimethyl sulfoxide, 1,4-dioxane, N, N-dimethylformamide, triethylamine, pyridine, and 1,4-butanediol diglycidyl ether. Short-term exposure of any of these solvents to the stained paper did not have any detectable effect on any of the stains. However, after 24 hours of soaking, 1,4-dioxane completely removed the stain produced by F. oxysporum and significantly reduced stains produced by P. notatum and C. globosum. Twenty-four hour exposure to N,N-dimethylformamide significantly reduced the stains produced by C. globosum and P. notatum and slightly reduced the stain produced by F. oxysporum. There was also a slight effect of this solvent on the surface of the stain produced by A. solani. Treatment with pyridine for 24 hours had a slight effect on the stains produced by C. globosum and F. oxysporum. Dimethylsulfoxide, triethylamine, and 1,4-butanediol diglycidyl ether had no detectable effect on any of the stains. Attempts at stain removal using some more common solvents such as acetone, ethanol, and xylene also had no detectable effect on stained paper.
Following exposure to the stained paper, the solvents were analyzed for the presence of pigment by recording their UV-visible spectrum (180–800 nm) against a pure solvent blank. In every case where stain had been reduced or eliminated, the solvent had absorption peaks that were not present in the pure solvent.
To assess the effects of the solvents on the characteristcs of the paper, samples of paper were soaked in 1,4-dioxane, N,N-dimethylformamide, or pryidine for different lengths of time and then tested by Crane and Co., Inc. Figure 10 shows the results of dry and wet tensile tests (tests of paper strength) on samples that had been exposed to solvents for the indicated time periods. Relative to the untreated paper (at time = 0), there does not appear to be any significant change in tensile strength after continued exposure to any of the solvents. To assess paper sizing, an ink test was conducted by Crane and Co. in which the ratings are given as good, fair, or poor: results with untreated paper, paper treated with N,N-dimethylformamide (1, 3, 6, or 18 hours), and paper treated with 1,4-dioxane (1, 3, or 6 hours) were rated good; results from short-term exposure to pyridine (1 hour) or 18-hour exposure to 1,4-dioxane were rated fair; and results from long-term exposure to pyridine (3, 6, or 18 hours) were rated poor. Thus it appears that N,N-dimethylformamide and short-term exposure to 1,4-dioxane do not significantly affect paper sizing, whereas exposure to pyridine, even for short periods of time, is detrimental to sizing. Other test results showed a great deal of variability that was independent of solvent exposure time and are therefore not included here.
Fig. 10.
Effects of solvent exposure on tensile strength of paper. Results from dry tensile tests (open symbols) and wet tensile tests (closed symbols) after soaking in 1,4-dioxane (▪,□), N,N-dimethylformamide (♦, ⋄), or pyridine (▴, ▵) for 0,3,6, or 18 hours
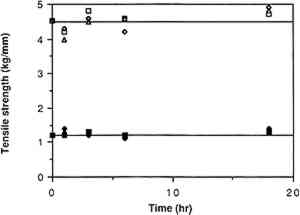 |
It should be noted that the solvents that were effective at removing fungal stains present varying degees of toxicity and should be handled with extreme care: 1,4-dioxane is a flammable liquid that produces a harmful vapor and may be irritating to skin, lungs, and mucous membranes; N,N-dimethylformamide produces a harmful vapor and is irritating to skin, eyes, and mucous membranes; pyridine is a flammable liquid that produces a harmful vapor and is irritating to skin and the respiratory tract. All of these solvents should be handled with gloves and used in a ventilation hood.
4 CONCLUSIONS
We have described a method for the rapid production of fungal stains on paper. The ability to generate such stains facilitates the systematic study of stain removal and stain prevention and obviates the need to experiment on valuable artworks. Our initial results suggest practical approaches to the removal of the four fungal stains we examined: The purple-pink stain of F. oxysporum may be completely removed by the long-term application of 1,4-dioxane; the green stain of P. notatum can be significantly reduced by either 1,4-dioxane or N,N-dimethylformamide; and the yellow-brown stain of C. globosum can be significantly reduced by either 1,4-dioxane or N,N-dimethylformamide.
Our results dealing with the effect of pH, temperature, and light on fungal growth and stain production suggest conditions that will minimize fungal invasion: Storage of objects at 4�C should prohibit fungal gowth; treatment of paper objects with agents that will either neutralize acidic paper or produce slight alkalinity should significantly reduce fungal infestation. While the growth of the fungal species examined here is apparently not dependent on light, stain production by F. oxysporum is reduced in the absence of light; thus storage in the dark should inhibit stain production by this fungus in particular.
The work described here is a first step toward cataloging practicable procedures for fungal stain removal. Future investigations of stain extraction using other solvents and promise to provide paper conservators with an assortment of techniques for the effective treatment of paper objects damaged by fungal infestation. Moreover, the continued characterization of the effects of environmental conditions on fungal growth and stain production should reveal more defininitive guidelines for the prevention of fungal invasion.
ACKNOWLEDGEMENTS
We wish to thank the Samuel H. Kress Foundation for its generous support of this project; William Moomaw, Chemistry Department, Tufts College, Williamstown, Massachusetts, for many helpful suggestions; Nancy Piatczyc, Williams College for assistance with scanning electron microscopy; Gary Wolpert and J. Preston Clark, III, Crane and Co. Papermakers, Inc., Dalton, Massachusetts, for paper analysis; and Robert Futernick for helpful comments.
REFERENCES
Agrawal, P. N., G. M.Verma, R. K.Verma, and D. D.Sahgal. 1963. Decomposition of cellulose by the fungus Chaetomium globosum. Indian Journal of Experimental Biology1:46–50.
Hulme, M. A., and D. W.Stranks. 1976. Heat tolerance of fungi inhabiting chip piles. Wood Science8:234–41.
Itabashi, M., Y.Murakami, and H.Nishikawa. 1955. Biochemistry of the filamentous fungi. X. Structure of tomichaedin. Tohoku Journal of Agricultural Research5:281–83.
Linfield, C. A.1986. A comparison of the effects of temperature on the growth of Fusarium oxysporum F. sp. Narcissi in solid and liquid media. Journal of Phytopathology166:278–81.
Nol, L., Y.Henis, and R. G.Kenneth. 1983. Biological factors of foxing in postage stamp paper. International Biodeterioration Bulletin19:19–25.
Peterson, G.1963. Separation of cellulases on Sephadex-100. Biochemica et Biophysica Acta77:665–67.
Rautela, G. S., and E. B.Cowling. 1966. Simple cultural test for relative cellulolytic activity of fungi. Applied Microbiology14:892–98.
Rothe, W.1950. New antibiotic. Pharmazie5:190.
Ruelius, H. W., and A.Gauhe. 1950. Fusarubin, a naphthoquinone coloring matter from Fusaria Annalen569:38–59.
Stoessl, A.1969. Metabolites of Alternaria solani. Canadian Journal of Chemistry47:767–76.
Strzelczyk, A. B.1981. Microbial deterioration of paintings on paper. In Microbial Deterioration, ed.A. H.Rose. Economic Microbiology6:218–20.
Szczepanowska, H.1986. Biodeterioration of art objects on paper. Paper Conservator10:31–39.
Szczepanowska, H., and C. M.Lovett, Jr.1988. Fungal stains on paper: Their removal and prevention. In The conservation of Far Eastem art, ed.J. S.Mills, P.Smith, and K.Yamasaki. London: International Institute for Conservation of Historic and Artistic Works. 13–14.
Verona, O.1969. Sulle Cause che provocano maculature rosa-violacee su carta. Cellulosa Carta5:27–31.
Wolf, F. A., and F. T.Wolf. 1947. The fungi. New York: John Wiley and Sons. 2:83–86, 96–164.
Zaprometova, K. M., T. G.Mirchink, D. S.Orlov, and A. A.Yukhnin. 1971. Characteristics of black pigments of the dark-colored soil fungi. Pochvovedenie7:22–30.
SOURCES OF MATERIALSFungal StrainsCarolina Biological Supply, Burlington, North Carolina Sabouraud dextrose agar and Bacto-agarDifco Laboratory, Detroit, Michigan
AUTHOR INFORMATION
HANNA MARIA SZCZEPANOWSKA is a certified conservator of art on paper and parchment. Recently she received a grant from the Fulbright Foundation and the Samuel Kress Foundation to work as a conservator and a consultant for the collection of the Knights of Malta at the National Library in Valletta and the collection of Prints and Old Master Drawings at the Cathedral Museum in Mdina Malta. She received her Masters Degree from the University of Nicolaus Copemicus in Torun, Poland in 1978. Her areas of specialty are medieval illuminated manuscripts and damage to art works caused by fungi. Address: United States Holocaust Memorial Museum, 2000 L St., N.W., Suite 717, Washington, D.C. 20036.
CHARLES M. LOVETT JR. is associate professor of chemistry at Williams College in Willamstown, MA. He received a Ph.D. in Biochemistry from Cornell University in 1985, and an M.S. in Chemistry from California State Polytechnic University in 1980. Address: Department of Chemistry, Williams College, Williamstown, MA 01267.
Section Index |