A STUDY ON DEVELOPMENT OF CRACKS ON PAINTINGS
ADAM KARPOWICZ
ABSTRACT—Humidity-induced movements were studied on biaxially stretched samples of sized and gessoed fabric and on films of size and gesso without fabric. In-plane strains were detected through observation of crack patterns formed in a brittle coating applied on the samples. Different patterns recorded during drying and humidification were found to depend on tension, humidity range, and time. The explanation of results is based on viscoelastic and anisotropic properties of the size layer resulting from changes in molecular conformation brought about by drying in restraint.
1 INTRODUCTION
OVER THE past 10 years there have been substantial developments in research on the mechanical behavior of paintings. The knowledge of processes leading to mechanical failure of paintings materials and their properties has been greatly enhanced through pioneering work by Mecklenburg (1982). It has been established that environmental changes induce dramatic responses in fabric and size. The layer of size is responsible for stress development during humidity decrease; high stiffness of fabric dominates in high humidity. The most brittle component of the composite—aged oil paint—fails when movements in the supporting size and fabric exceed its rupture strains.
Stress distribution on paintings and the resulting cracks in the paint layer were simulated on a computer with the Finite Element Method (FEM) (Kilpatrick 1980; Colville et al. 1982). Elastic properties of materials were assumed and based on uniaxial tests.
In this work the humidity-induced movements were observed on biaxially stretched samples of primed fabric with a commercially available brittle coating taking the place of aged paint. The coating is widely used in industrial stress analysis as a form of strain gauge; it cracks when strains in a support exceed its breaking strains. The results reveal nonelastic properties of size and are consistent with a study on dimensional response of size in changing humidities (Karpowicz 1989).
2 METHOD
SAMPLES OF fabric (Belgian linen, 500 g/sq.m, thread count 13 [warp] � 12.5 [weft]/sq.cm) were stretched on spring-loaded frames with tension 250 g per 1 cm of stretcher bar (1.4 lb/in); they were thoroughly wetted and dried under tension. Care was taken to avoid deviation of fibers from straight lines. The samples were transferred onto wooden strainers without the loss of tension and sized with 6% w/v rabbit-skin glue gel (M. Grumbacher, Inc., New York, New York 10001). The sizing was followed by 6% glue/chalk gesso, coated with a thin layer of 3% size. Dimensions of samples were 28 � 56 cm (11 � 22 in), warp direction lengthwise. Total amount of size (including the size in gesso) was approximately 0.0046g/sq. cm. The stretched samples were stored at 45%–55% RH/21�C (later referred to as normal humidity) for 1 week to 3 months prior to testing.
In certain cases, to eliminate the influence of canvas on the direction of movements, samples without fabric were prepared by applying size and gesso onto a stretched sheet of Mylar (0.5 mil, Dupont). The dried samples were protected from the front with tissue/Beva facing (Beva 371, Adam Chemical Co.). Both Mylar and the facing were removed prior to tests.
In preparation for testing, the samples were transferred onto a frame equipped with a system of pulleys and springs to achieve different tensions or onto rigid aluminum strainers. The strainers were used to investigate movements in restraint as they occur on actual paintings. Edges of the samples were attached to bars with contact adhesive, thus avoiding tacking margin effects, which were tested separately. The entire surfaces of samples were exposed from the front and back to eliminate the differential response to humidity changes of central parts of samples as compared with edges overlying stretcher bars. Tension adjustments were made in normal humidity prior to the application of the brittle coating.
The ready-made solution of the brittle lacquer (Tens-Lac, TL-500-85, Measurement Group Inc., Raleigh, North Carolina 27611) was applied by spraying, 20% v/v trichloroethylene having been added to slow evaporation and to prevent formation of air bubbles in the coating. Upon drying/conditioning, the coating became progressively more brittle. Estimation of breaking strains was made using a manufacturer-supplied calibrator and cantilever calibration bars. The bars and tested samples were sprayed with the coating together and kept in the same conditions. When the coating reached desired brittleness as tested on the bars with the calibrator (at least 22 hours), humidity was changed to induce in-plane movements in the supporting samples and cracks in the coating.
Cracks are always perpendicular to the principal direction of tensile stress and strain, and patterns of cracking represented direction of movements resulting in extension. These local displacements were not always reflected in actual enlargement of samples. They were also caused by the inability of samples to move along rigid stretcher bars and by the geometry of samples. If magnitudes of strain were equal in two perpendicular directions, random patterns occurred (Dally and Riley 1978).
Little data are available on mechanical properties of the coating. The tensile strength is generally estimated in the literature at about 200 psi in 50% RH (Kobayashi 1987), about half that of oil paint (Kilpatrick 1980). Stiffness of the coating was certainly less than the size layer, as it could not restrain movements resulting from free swelling of size in high humidities, over 93% RH.
Difficulties during tests were associated with the long time of conditioning for the coating (up to 170 hours for low rupture strains). During that time, samples underwent sufficient creep to show as cracks in the coating when conditioned in higher humidities. This effect tended to obscure cracks resulting from humidity change. Therefore, cracks had to be detected before and after the change for comparison. It was also essential that the stretched samples be even, without out-of-plane distortions that would later inevitably lead to uneven tensions and irregular crack patterns.
Cracks were visible, but to record the results they were developed with black ink. Excess ink and the coating was later removed to leave black crack lines on a white gesso background.
Cracks formed at higher humidities were always sparser but wider than cracks observed in lower humidities. In higher humidity more extension was possible, and stress could be released through extension of size within an already-formed crack. This effect, no doubt, applies to cracking of aged paint on paintings.
Approximate minimum strains are given with each movement. The strains measured with the calibrator were always smaller than the actual strains on samples due to the much longer times of loading for samples. For faster movements (up to 3 hours) the actual strains can be higher by about 30% to 100%; for slower movements (up to 20 hours), several times the given values of strain (Dally and Riley 1978). For movements in high humidities, actual values of strain could be much higher due to the extension of size in cracks.
3 RESULTS AND DISCUSSION
3.1 Drying
IT HAS been observed that patterns of cracking vary with tension applied before drying, with the rate of drying, and with humidity range. The movements ceased about 1 to 3 hours after the change of humidity.
A pattern resembling “waves” radiating from corners (fig. 1) was observed at tension 30g/cm and at no initial tension (rigid aluminum strainer for the latter), during drying from 93% RH to 83% RH, 93% to 75% RH, and 93% RH to normal humidity. Drying in the higher humidity range gave better-developed cracks than faster drying to normal humidity. From 93% to 83% strains were about 0.1% or more; from 93% to 75%, about 0.08%. Drying from 93% RH to normal humidity gave only faint cracks at 0.05% strain. The cracking always started at longer sides of samples near corners and progressed toward centers. Samples with or without fabric gave the same patterns, clearly resulting from shrinkage of the desiccated size layer.
Fig. 1.
Crack patterns observed during humidity decrease at low tension. One-quarter of sample shown
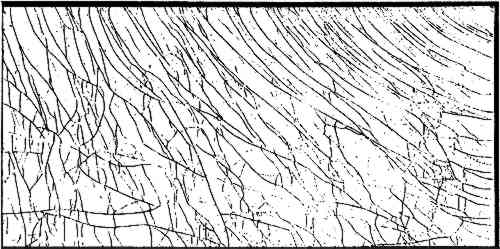 |
Samples with fabric stretched at higher tensions of 200 g/cm (1.11 lb/in) and 350 g/cm (1.96 lb/in) gave very little cracking at corners when dried from 93% RH to 75% RH (min 0.07% strain). The cracks tended to become random toward the center of samples and to concentrate at the edges.
Tacking margin cracks (fig. 2a) were in good agreement with Kilpatrick (1980).
Fig. 2.
Crack patterns at tacking margins: (a) during humidity decrease; (b) during humidifcation
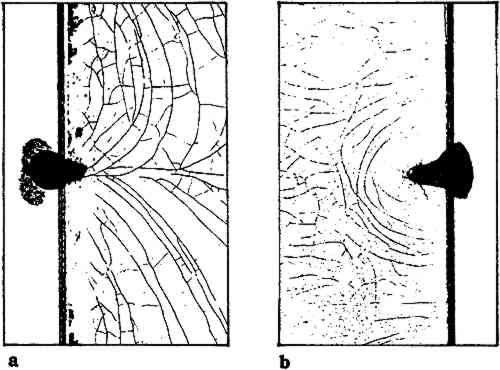 |
No cracks were observed on drying from 75% and from lower humidities, even at low rupture strains (0.05%) of the coating and high tension.
The pattern formed at low tension (fig. 1) has been generally recognized as the most typical craquelure on paintings (Keck 1969). Often the regular “waves” and lines perpendicular to edges of a painting become random or disappear toward the center (fig. 3).
Fig. 3.
Typical craquelure on paintings. One-quarter of sample shown
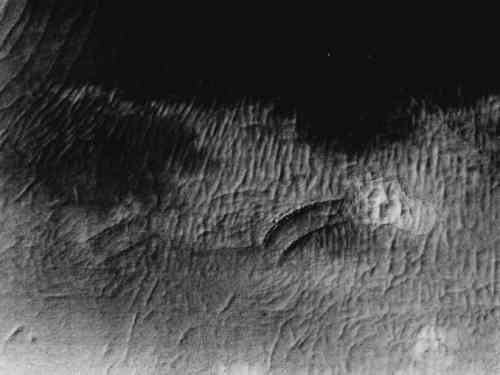 |
During restrained contraction of size upon drying, local stresses and overall tension continued to increase. Under low tension, shrinking of size was restricted mainly along rigid stretcher bars, causing extension and cracks perpendicular to the bars. The cracking started where stress was the highest, at areas farthest from the center of rectangular samples, at longer bars near the corners. Cracks would begin to curve, forming the “waves,” since shrinking of size continued toward the center of a sample. Overall tension increased as the humidity continued to drop. At a certain higher tension, stress across the sample and perpendicular to the bars began to reach levels equal to stress along the bars, and a random pattern occurred. With gradual decreases of humidity, more cracking took place along the “typical” lines; during faster drying, random patterns would dominate. It is interesting that the rapid increase in stress did not cause more strain and cracking, as one would expect assuming the elastic behavior of size. Also, strains in the size layer below 75% RH were too small to crack the coating, which was sensitized to very low rupture strain of 0.05%, despite very high stress. The inhibition of movement at high tension can result from an increase in stiffness of the size in the direction of loading. This effect is common for organic, viscoelastic polymers and was investigated for gelatin, the purer form of size (Gupta 1964).
In the elastic model (Kilpatrick 1980; Colville et al. 1982), the typical pattern results from differential drying of a central part of a painting as opposed to sections around edges and over stretcher bars. These sections remain “wet” and relatively unstressed. The typical pattern observed here occurred at low tension with entire samples exposed to free circulation of air. It seems the low tension takes the place of the unstressed outside edge in the elastic model, distribution of stress remaining the same. Many paintings, however, show the “typical” pattern exactly in areas over and along stretcher bars. The occurrence of typical craquelure at edges would not be possible in the FEM model but agrees well with the results of this work.
3.2 Humidification
The effects of humidification were dependent on initial tension applied, period of time elapsed after the change of humidity, and humidity range.
All stretched samples (30 g/cm tension and above) gave exactly the same pattern (fig. 4) when moved from lower to higher humidity, regardless of humidity range (humidities 10% to 33%, 33% to normal humidity, normal to 75%, and 75% to 93% RH were tested). The cracks originated at the corners and spread toward the center. The movement ceased after about 30 minutes for samples without fabric and after 3 hours for samples with fabric. The levels of strain did not seem to exceed 0.12% strain at 30 g/cm for all humidity ranges (not counting the extension within cracks in higher humidities). Samples under no tension did not give this pattern, but exhibited only random swelling with a tendency to expand more lengthwise. Also, when any stretched sample fragments were restrained from moving, the pattern became distorted. The same pattern showed on samples that were allowed to dry previously on rigid strainers and to develop tension of their own (Karpowicz 1985). For lesser tensions, the movement was simultaneous with less pronounced perpendicular cracking.
Fig. 4.
Crack patterns during humidification: expansion/tension-related movement. One-quarter of sample shown
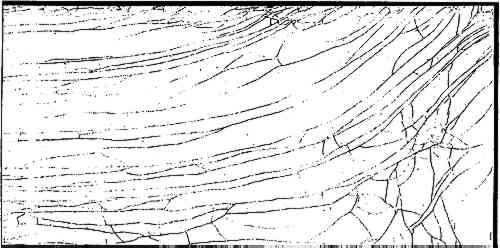 |
In higher humidities, for samples at tension 30 g/cm (with and without fabric), 100 g/cm (0.56 lb/in, with fabric) and at no tension on rigid strainers (without fabric), after the initial movement as above, direction of strains changed to give patterns similar to drying (figs. 5, fig. 6). Both movements were separated by placing samples in higher humidity immediately after applying the coating. The “wet” coating was not brittle enough to crack with the initial tension-related movements and cracked later, showing only the second movement. Higher strains were detected in 93% RH (min 0.1% after 20 hours, actual level of strain could approach 1%) than in 75% RH (min 0.05% after 192 hours). No movement was detected in normal and lower humidities.
Fig. 5.
Crack patterns resulting from restrained shrinkage of size in 93% RH. Samples without fabric; one-quarter of sample shown. Reprinted from Studies in Conservation 34(2):72 with the permission of the International Institute for Conservation of Historic and Artistic Works, 6 Buckingham Street, London, England WC2N 6BA.
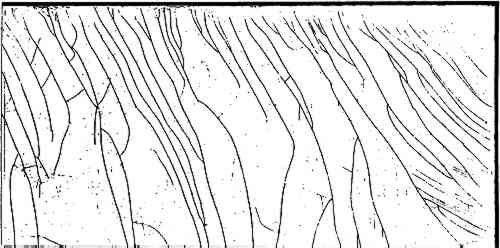 |
Fig. 6.
Crack patterns resulting from restrained shrinkage in 93% RH. Samples with fabric; one-quarter of sample shown. Reprinted from Studies in Conservation 34(2):72 with the permission of the International Institute for Conservation of Historic and Artistic Works, 6 Buckingham Street, London, England WC2N 6BA.
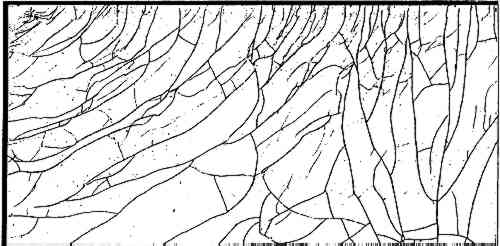 |
At higher tension (200g/cm and 350g/cm) in 93% RH (movements in 75% RH not having been investigated) and with the initial tension-related movements eliminated as above, different patterns were obtained (fig. 7).
Fig. 7.
Crack patterns resulting from creep of fabric at high tension in 93% RH. One-quarter of sample shown
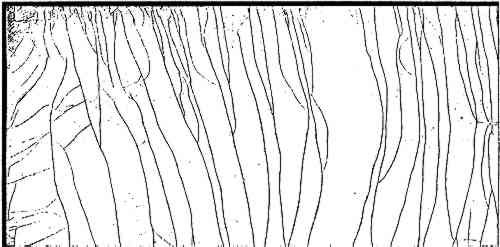 |
Upon humidification, tacking margins gave cracks exactly perpendicular to drying cracks (fig. 2b). This effect was simultaneous with the initial tension-related movement (fig. 4).
There is little doubt that the initial movement (fig. 4) is a manifestation of an outward pull exerted on the film of size and on the fabric by the rigid bars and is simultaneous with the expansion of the size layer through absorption of water in higher humidity. There is a marked similarity in cracking patterns with those computed as “keying-out” effects (Kilpatrick 1980; Colville et al. 1982). The movements are essentially the same as here, where the expanding size yields to the outside tension. The smaller perpendicular strains at low tension indicate continuation of the expansion in all directions; for higher tensions the overall expansion could have been absorbed by the initial movement.
The initial expansion/tension-related movements stopped after 3 hours for samples with fabric, apparently when the samples reached an equilibrium in higher humidity. This is in good agreement with results by Daly and Michalski (1986), where the dimensional response of fabric-supported samples to changed humidity ceased after 2 to 3 hours. The response of samples without fabric was found here to be faster (30 minutes).
At low tensions, after the initial expansion, distribution of stress changed, as if the size layer (and fabric, if present) began to contract (figs. 5, fig. 6, compare with desiccation on fig. 1). To investigate this effect, a new series of experiments was set up. Samples without fabric were stretched in normal humidity at 30 g/cm and at no tension on strainers. The change of humidity to 93% RH caused expansion, which was manifested in wrinkles perpendicular to edges. After about 20 minutes the samples began to shrink and flatten out; in 45 minutes they were flat and visibly taut.
The tests proved that a size layer is capable of contraction in higher humidities and of developing stress, the distribution of which results in the “typical” pattern of craquelure, provided the tension is low (here less than 200 g/cm for samples with fabric). This phenomenon would be difficult to explain on the basis of purely elastic behavior of size. However, it becomes understandable if nonelastic properties are assumed. For the latter, mechanical properties change depending on the range of humidities in which tests are performed.
The dimensional response of unsupported films of size in different humidities was investigated in detail and found to follow exactly the above expanding and contracting movements (Karpowicz 1989). The layer of size after application on canvas shrank with the loss of moisture. The shrinkage was restrained in plane of the film by the stretcher bars and the underlying fabric. The molecular structure of a film dried in restraint is not the same as that of freely contracted films. Its structure has become strained as well to reflect extension of the film. Below the moisture content of about 17% (Ueno and Ono 1963) (which corresponds to about 70% RH [Bull 1944]), upon reaching its glass transition temperature (Tg), the size enters its glassy state, in which the molecules are immobilized and the strains frozen in the film (Ueno and Ono 1963). When the sized fabric is taken off the stretcher only small elastic contraction is possible; large viscoelastic strains, which took place at higher water content, cannot be relieved. When the restretched sample is exposed to humidities above 70% RH (above Tg), the water content becomes sufficient to plastify the film and the “frozen-in” strains can be released by the overall contraction of the samples. The shrinkage of fabric, known to occur above 80% RH (Mecklenburg 1982), could also have contributed to the movements. Before samples reached moisture content corresponding to Tg, when they were exposed to any of the high humidity levels studied here, they all expanded by swelling to give the initial tension-related movements.
Cracking in the “typical” directions associated with these effects takes place at lower tensions. High tension in higher humidity gave a different pattern (fig. 7), apparently resulting from creep of fabric in the direction of warp.
3.3 Strain-Induced Orientation in the Size Layer
The tacking margin effects on humidification (fig. 2b) show an interesting phenomenon of expansion by swelling in higher humidity lateral to the previous direction of stress and strain, during drying, after application on canvas. The occurrence of an identical crack pattern has been reported for a painting rapidly humidified during a flood (Pocobene and Hodkinson 1987). This effect demonstrates an important property of size: in-plane orientation on the molecular level caused by strain.
The network of collagenlike fibrils in the gelled layer of size on fabric undergoes distortions in the direction of strains during drying and shrinkage (Karpowicz 1989). The fibrils become oriented, with the degree of orientation dependent on the magnitude of strain (Burdygina et al. 1969). At the same time, the decreasing thickness of the film causes compression of the network and orientation of the fibrils parallel to the plane of the film. The maximum orientation seems to occur when water content drops to 85%–78% in the gel (well above Tg) and will be enhanced by slow drying. Gradually the structure becomes too rigid at lower water contents; no orientation is possible below 15% of water in the film in the glassy region below Tg (Coopes 1968). The dried film can be expected to exhibit in-plane orientation of collagenlike fibrils, parallel to the plane of film and in the direction of strains induced by drying.
The effects of orientation would be the highest close to the outside perimeter of paintings, farther from the center, where the highest strains during drying occurred. Also, slow drying in the areas over the stretcher bars would allow more orientation there.
Oriented polymers swell mainly across the axis of fibril orientation, and the anisotropy of swelling is a measure of orientation in polymers (Turner 1948). At the tacking margin the layer of size expanded during humidification (fig. 2b) across the direction of drying strains (fig. 2a). It has been found that isotropic swelling is manifested only by gelatin films unrestrained during drying (Simms and Blake 1960). The presence of orientation in films of size dried in restraint was confirmed by observations of strain-induced birefringence (Karpowicz 1988).
4 CONCLUSIONS
DESPITE ITS limitations, the brittle coating method proved useful in studying humidity-induced movements on paintings. The film of size was found not only to expand in high humidity, but also, following the brief expansion, to contract when its moisture content reached sufficient levels. The magnitude of the contraction will vary depending on humidity range and the length of time a painting is kept in high humidity. Short exposures may not be sufficient to bring about cracking of paint. Subsequent periods of lower humidity, however, will induce further contraction of the size layer and progressive increase of stress and strain (Karpowicz 1989). At lower tension the direction of the strains followed that of the most common pattern of craquelure on paintings. This direction will be the same in high humidity and during later desiccation. At higher tension the stress distribution will be different. A recent paper, however, reported that sized fabric stretched at high tension appeared to relax rapidly and stabilize at lower levels of tension (Berger and Russell 1988).
During the process of viscoelastic recovery in high RH, the layer of size could have acquired anisotropic properties through molecular orientation in the direction of shrinkage (Karpowicz 1989)(figs. 5, fig. 6). It is possible that the stiffness of size became greater in the direction of orientation (Turner 1948). This may explain why strains during drying that followed were small, despite the development of high stress, and caused only limited cracking in the same typical direction. It is important to realize that the size, and presumably the fabric, are both nonelastic materials. The effects of time on the development of stress and strain are of primary importance.
Fabric, which also contracts in high humidity, may contribute to these movements and requires another study. The ultimate mechanical properties of aged paint, especially breaking strains at lower rates of loading, must also be investigated to take into account the viscoelastic, time-dependent behavior of size.
ACKNOWLEDGEMENTS
THE AUTHOR wishes to thank Stefan Michalski and Debra Daly, Canadian Conservation Institute, and the late Gerry Hedley, Courtauld Institute of Art, for their helpful remarks at the beginning stages of this project.
REFERENCES
Berger, G. A., and W. H.Russell. 1988. An evaluation of the preparation of canvas paintings using stress measurements. Studies in Conservation33:187–204.
Bull, H. B.1944. Adsorption of water vapor by proteins. Journal of the American Chemical Society66:1499–1507.
Burdygina, G. I., I. M.Fridman, P. V.Kozlov, and V. A.Kargin. 1969. Effects of orientation on structural transitions in gelatin in wide temperature interval. Vysokomolekuliarnye SoedineniiaA 11:912–19.
Colville, J., W.Kilpatrick, and M. F.Mecklenburg. 1982. A finite element analysis of multi-layered orthotropic membranes with application to oil paintings on fabric. In Science and technology in the service of conservation, ed.N. S.Brommelle and G.Thomson. London: International Institute for Conservation of Historic and Artistic Works. 146–50.
Coopes, I. H.1968. The optical rotation of gelatin films. Journal of Polymer Science6, pt. A-1:1991–99.
Dally, J. W., and W. F.Riley. 1978. Experimental stress analysis. New York: McGraw-Hill.
Daly, D., and S.Michalski. 1986. Recent developments in research in the Fine Arts Laboratory at CCI. Paper presented at the IIC–Canadian Group 12th Annual Conference, Winnipeg, Manitoba.
Gupta, R. C.1964. The influence of loading on the rigidity modulus and plastic flow in adhesive films. Applied Science Research13, sec. A:61–64.
Karpowicz, A.1985. Effects of stretcher construction on crack patterns in fabric-supported paintings: Preliminary results. Poster session presented at IIC-Canadian Group 11th Annual Conference, Halifax, Nova Scotia.
Karpowicz, A.1988. Viscoelastic and anisotropic properties of films of size on paintings. Paper delivered at the IIC–Canadian Group 14th Annual Conference, Toronto, Ontario.
Karpowicz, A.1989. In-plane deformations of films of size on paintings in the glass transition region. Studies in Conservation34:67–74.
Keck, S.1969. Mechanical alteration of the paint film. Studies in Conservation14:9–30.
Kilpatrick, W.1980. A finite-element analysis of multi-layered orthotropic membranes with application to fabric-supported oil paintings. Ph.D. diss., University of Maryland.
Kobayashi, A. S., ed.1987. Handbook on experimental mechanics. Sponsored by Society for Experimental Mechanics. Englewood Cliffs, N.J.: Prentice-Hall.
Mecklenburg, M. F.1982. Some aspects of the mechanical behavior of fabric-supported paintings. Report to the Smithsonian Institution.
Pocobene, G. and I.Hodkinson. 1987. A case of flood damaged paintings: A preliminary report on their mechanical behaviour and conservation. (Paper presented at the IIC–Canadian Group 13th Annual Conference, Victoria, British Columbia.
Simms, E. M., and J. N.Blake. 1960. The swelling of gelatine films. Nature187:998.
Turner, A., Jr.1948. Mechanical behavior of high polymers. New York: Interscience.
Ueno, W., and I.Ono. 1963. Creep of gelatin. Journal of the Society of Materials Science (Japan) 12:81–86.
AUTHOR INFORMATION
ADAM KARPOWICZ received his M.Sc. degree in chemistry from the University of Warsaw, Poland, and subsequently a M.A. degree in conservation of paintings from the Academy of Fine Arts, Warsaw. His research interests are in the structure and the dimensional response of paintings for the New Brunswick provincial government. Address: Owens Art Gallery, Mount Allison University, Sackville, New Brunswick, Canada EOA 3CO.
Section Index |