DEGRADATION IN WEIGHTED AND UNWEIGHTED HISTORIC SILKS
Janet E. Miller, & Barbara M. Reagan
ABSTRACT—The influence of type of silk, age, and weighting agent on the physical and chemical deterioration of silk fabrics was evaluated by comparing the strength, elongation, amino acid content, viscosity, and infrared spectra of 54 undyed, historic silk fabrics with new silk plain weave fabric. Fiber fracture patterns also were characterized. Metallic elements present on the silks in various amounts, as determined by neutron activation analysis, were tin and to a lesser extent iron, aluminum, chromium, copper, barium, and arsenic. In general, tenacity, elongation, and viscosity were inversely related to the age of the silk. The variety of fiber fracture patterns in the historic silks reflected the diverse histories and causes of deterioration.
1 INTRODUCTION
SILK FABRICS DATING FROM the late 19th and early 20th centuries often are severely degraded as a result of weighting agents that were used to increase or replace the weight lost during the degumming process. Weighted silk is usually heavier and thicker and has better draping qualities than unweighted degummed silk. However, both the process of weighting and the agents used may weaken the silk fiber, shorten its wear life, and make it more susceptible to degradation by acid, alkalies, high temperature, and light.3, 7 As a result, many antique silks exhibit lengthwise splits and cracks at folds and creases and are so weak that they must be supported to be handled or displayed.
Historically, the artificial weighting of silk has been practiced for hundreds of years using a variety of inorganic and organic compounds. In the 20th century, the most common weighting agents were tin salts, such as stannic or stannous chloride, which were used alone or in combination with phosphates and silicates.3, 13 Weighting agents usually were applied to silk during the dyeing operation. Because fabrics were often sold by weight, some dyers and finishers would overweight silks for greater profits, even though this practice severely weakened the fiber. The adverse effects of weighting agents on silk fabrics resulted in a 1938 Federal Trade Commission rule requiring manufacturers to designate on labels the percentage of weighting agents applied if greater than 10% by weight (or 15% for blacks).
The selection of appropriate conservation methods for historic silk textiles and costumes may be impeded by the lack of knowledge pertaining to the physical and chemical changes that occur in silk fibers during aging or to the deleterious effects of dyeing and finishing chemicals, such as weighting agents. Furthermore, research on fiber aging has focused primarily on cotton and wool, while few studies have been conducted on silk. Information characterizing degradation in historic silks has been extrapolated primarily from research conducted on new silks, which have been studied to a limited extent for quality control purposes (i.e., to minimize damage during manufacturing), or from artificial aging experiments. Bresee and Goodyear4 examined fiber fracture patterns in naturally aged silks that had been degraded by heat treatments and accelerated light exposure. Similarly, in artificial aging experiments on silk, Leene et al.9 evaluated changes in the degree of polymerization and fiber tenacity and elongation. These authors concluded that aging and deterioration resulted from a complex combination of degradation forces.
In a recent study by Kuruppillai8 et al., the extent to which a deacidifying agent and two antioxidants reduced degradation in new silk was evaluated after exposure to heat and light. These additives were not effective in retarding strength loss, but they did reduce the formation of amino groups. Needles et al.12 investigated the effects of natural dyes and mordants on degradation in silk and wool subjected to xenon light and soil burial tests. Results showed that the mordanting agents improved the lightfastness of the dyes but increased burial-induced color change.
This research was undertaken to characterize physical and chemical degradation and changes in proerties of naturally aged silk. This information will aid in developing and selecting appropriate methods of conservation. The samples evaluated were new, unweighted silk habutai and naturally aged, unweighted and weighted, historic silk fabrics. Habutai is a soft, light, closely woven Japanese silk fabric woven from raw silk filature with little or no twist.7 The silk habutai also was exposed to chemicals, boiling water, and heat to examine their effects on the physical and chemical structure of the fiber, but the results are not reported here.11 Methods of analysis included tests of yarn tenacity and elongation, dilute solution viscosity, amino acid content, photoacoustical infrared spectroscopy, and optical and scanning electron microscopy. The metallic weighting agents present on the weighted historic silks were identified using neutron activation analysis.
2 METHODS AND PROCEDURES
2.1 Fabrics
THE NATURALLY AGED, UNDYED, HISTORIC SILK fabrics used in this study were selected from samples obtained through letters of request sent to major museums, universities, historical societies, and private collectors in the United States. The 54 undyed, historic silk samples evaluated were between 20 and 400 years old and included both unweighted and weighted silk fabrics. Among the historic silk fabrics evaluated, 49 contained cultivated (Bombyx mori) silk, including 21 plain weaves, 10 satins, 12 pattern weaves, 2 crepes, 2 twills, and 2 stockinette knits. The remaining 5 fabrics were plain weave pongees containing wild (Tussah) silk. Both longitudinal and cross sectional slides of silk fibers from each fabric were examined under an optical microscope to identify and classify silk type. Wild silk fibers exhibited lengthwise striations and wedge-shaped cross-sections, compared to the smoother surface and more triangular cross-sections of cultivated silk fibers. The specific histories of the silk samples, except for the approximate age, were largely unknown. However, it was assumed that manufacturing methods, use, care, and storage conditions were typical. The new silk fabric used for comparison was an unweighted, undyed, 160 � 160, plain weave silk habutai (style #604, Test-fabrics, Inc.) constructed from 30 denier, multifilament yarns. Silk style #604 was degummed after it was woven.
2.2 Characterization of Historic Silk Fabrics
The general condition of the historic silks was classified as good, fair, or poor, based on the number of breaks, snags, and stains and whether it appeared to be strong enough to be displayed without reinforcement. Also determined for each sample were fabric count (yarns per inch) and warp yarn twist and denier according to the test procedures in ASTM D 3775–85, Standard Test Method for Fabric Count of Woven Fabric; ASTM D 1423–82, Standard Test Method for Twist in Yarns by the Direct Counting Method; and ASTM D 1059–83, Standard Test Method for Yarn Number Based on Short-Length Specimens, respectively.1
The historic silk fabrics were subdivided according to age and weighting groups (unweighted and weighted). The following nine age groups were established: 1 (new silk habutai), 2 (1960–75), 3 (1940–59), 4 (1910–39), 5 (1890–1909), 6 (1790–1889), 7 (1700–89), 8 (1600–99), and 9 (1500–99).
The presence and type of weighting agents in the historic silk fabrics were determined by neutron activation analysis (NAA). Specimens (2.5 cm � 5.0 cm) were weighed, placed in a Triga Mark II nuclear reactor, then analyzed using a germanium-lithium gamma detector connected to a Canaberra 8180 multichannel analyzer. Weighting standards were prepared for nine water soluble, metallic salts (SnCl2, CuSO4, Al(SO4)3, K2Cr2O7, FeSO4, InCl3, BaN2O6, ZnSO4, and AsCl3), pipetted onto samples of the silk habutai, then irradiated under conditions identical to those used for the historic silk samples. A computer program developed by Higginbothem5 was used to compute the approximate concentration of the metallic elements in the historic silks.
2.3 Methods of Analysis
2.3.1 Yarn Tenacity and Elongation
Strength and elongation tests are used widely for assessing fiber degradation in textile substrates. Thus, yarn-breaking tenacity and elongation tests were performed on the new silk habutai and historic silks to assess the effects of aging on fiber properties. Yarn-breaking load was measured on a Scott Model CRE, following the procedures in ASTM D 2256–80, Standard Test Method for Breaking Load (Strength) and Elongation of Yarn by Single Strand Method,1 except that a multiple-end test method consisting of five warp yarns per test was used. Ten multiple-end tests were performed on each sample. Mean yarn tenacity (based on yarn breaking load and denier) in grams of force per denier and percentage elongation at break were then calculated.
2.3.2 Dilute Solution Viscosity
Viscosity measurements also are used for detecting degradation in textiles. Polymer chain scission and bond breaking at side chains often results in appreciable decreases in viscosity. In this study, the intrinsic viscosities of the new and historic silk fabrics were determined, following the lithium bromide silk viscosity test (SNV 95595-1963) developed by the Swiss Standards Association.14 Specimens weighing 0.100 g each were treated in small test tubes with a saturated lithium bromide solution, then placed in a Precision Scientific laboratory oven for three hours at 60�C. After cooling, the silk-lithium bromide solutions were diluted with 5 ml of distilled water, filtered through an ASTM 40–60 fritted glass filter, and then placed in Cannon semimicroviscometers. After temperature equilibrium was reached in a constant temperature bath (20�.C), solution flow times were recorded. The average of three effluent times per specimen was used to compute intrinsic viscosity or viscosity number.
2.3.3 Amino Acid Analysis
Seventeen amino acids have been identified in silk fibroin; however, their specific ratios are dependent on the species and strain of silk worm and environmental rearing conditions, processing, use, age, and deterioration. The relative amounts of amino acids present influence the physical properties of the fiber. In this study, the amino acid content of the new and historic silk specimens was evaluated using a Dionex D 300 microbore, single column, amino acid analyzer with a Fisher recorder and a Columbia Scientific integrator. After the silk was hydrolyzed in a 3.0 N p-toluenesulfonic acid bath at 100� C for 30 hours, the amino acids were separated using high-pressure liquid chromatography (HPLC) with a five buffer, stepwise, ion exchange system, and then detected colorimetrically as ninhydrin derivatives.
2.3.4 Optical and Scanning Electron Microscopy
The surface characteristics and fiber fracture patterns in the historic silks were evaluated from longitudinal and cross sectional fiber slides viewed at 430x magnification with an American Optical trinocular light microscope. Photomicrographs were made of selected examples of fiber fracture patterns using an ETC Autoscan scanning electron microscope (SEM). The specimens were prepared by coating the fibers with a thin layer of carbon, followed by a thin layer of gold-palladium.
2.3.5 Photoacoustical Infrared Spectroscopy
An IBM Infrared Spectrophotometer, Model 98, with a photoacoustical accessory was used to record the IR spectra for selected samples of the new and historic silks. In order to eliminate water from the silk specimens, they were stored in a desiccator for one week prior to analysis and then tested in a nitrogen environment. A computer-assisted, subtraction technique was used to detect differences resulting from the experimental treatments. This technique allows for fibers or fabric to be analyzed without grinding the silk into powder to produce powder pellets.16
2.3.6 Statistical Analyses
The silk fabrics were subdivided into nine age classes and two weighting groups (weighted and unweighted). Regression and orthogonal trend analyses were performed on the data for tenacity, elongation, and viscosity using general linear model (GLM) least square tests because of the unequal cell sizes. Sample means were plotted to determine changes over time and with weighting. The analysis of variance test was used to determine significant differences in the amino acid contents among the naturally aged and new silk samples.
3 RESULTS
3.1 Characteristics of Historic Silk Fabrics
THE TYPE OF SILK (cultivated [Bombyx mori] or wild [Tussah] silk), fabric type and count, warp yarn denier and twist, condition, age group, and corresponding code for the naturally aged, historic silk fabrics are presented in table 1. Fabric counts ranged from 12 to 280 yarns per inch in the warp and from 32 to 158 yarns per inch in the filling. The mean fabric counts for the 54 fabric samples were 133 (warp) � 94 (weft). The warp yarn deniers, which were used in calculating breaking tenacity, also varied considerably and ranged from 22 gf/den. to 327 gf/den. Most of the warp yarns had little or no twist (i.e., TPI = ≤5 twist per inch). Over 50% of the historic fabrics were designated as being in good condition, whereas approximately 25% were in poor or fair condition.
Table 1 Construction Characteristics, Condition, and Age of Historic Silk Fabrics
A summary of the neutron activation analysis (NAA) data showing the approximate percentage by weight of the metallic elements present in the unweighted, new silk habutai (designated C-55) and the 54 historic silk samples, the total percentage of weighting elements, and the weighting classification (unweighted or weighted) are presented in table 2. Fabrics containing a total of 1% or more of metallic elements were classified as weighted, whereas those containing less than 1% were designated as unweighted. (Note that this is not the percentage of the metallic salt.) Fifteen of the 54 fabrics were considered weighted.
Table 2 Metallic Elements Present in Silk Fabrics
All of the historic silk fabrics and the new silk habutai contained Mn56, a daughter isotope of iron, indicating the presence of iron in amounts varying from <0.01% to 0.63%. Trace amounts (<0.01% to 0.15%) of aluminum were found in 44 samples. Barium, which has been used as both a delusterant and weighting agent, was present on 23 samples in amounts ranging from 0.01% to 1.68% in all age groups, except 2 (1960–75). Since iron and aluminum are common contaminants in water, perhaps these elements were deposited on the silks during wet processing. Na and Cl were found in all samples but were not quantified. NAA is very sensitive to both Na and Cl and can readily detect contamination from handling. Iron and aluminum were found in all age groups, including the unweighted, new silk habutai.
The highest element percentages were associated with tin, which was expected since tin salts were commonly used in silk weighting. Tin was detected in 13 historic silks, and in all but two specimens the concentrations exceeded 3% of the total fabric weight. Interestingly, the samples that contained tin and chromium were in age groups 4 and 5 (1890–1939).
Eleven silk fabrics in three of the four age groups between 1700 and 1939 contained potassium in amounts of 0.05% to 2.45%. Low concentrations (<0.01%) of copper were detected in six samples, all of which dated earlier than 1890; and five contained trace amounts of arsenic (<0.01% to 0.13%), with the same age group distributions as potassium. Indium, a rare earth metal not commonly used as a weighting agent, was detected in six fabrics at concentrations of <0.01%. Its presence may have been due to transformation of Sn125 to In 116M in some of the tin-weighted samples.
The silk weighting literature records that lead acetate, antimony, zinc, and other metallic salts were sometimes used for weighting silk but were not of great commercial importance. Lead could not be detected using the particular irradiation times, and antimony and zinc were not detected. The research reported here only analyzed undyed silk, so lack of zinc weighted samples and low iron concentrations were not unexpected. Both zinc and iron mordants and weighting were associated with silk dyed black or dark blue.3, 7, 15
A limitation of NAA is its inability to detect lead, a known weighting agent, because irradiated lead isotopes are stable and do not become radioactive except after extended periods of radiation. Other methods of elemental analysis may be more sensitive than NAA in detecting minute amounts of some of the known weighting elements such as copper. Hence, a combination of analytical methods such as NAA, atomic absorption, and X-ray fluorescence would be needed to identify all possible weighting elements.
Separate NAA tests were conducted on the warp yarns only from two badly deteriorated silk fabrics (with lengthwise splits) dating from 1910 to 1915. The filling yarns could not be analyzed because they disintegrated when raveled from the fabric. The total percentage of elements present for these fabrics was 9.47% and 10.98%, whereas the corresponding warp yarns contained only 4.40% and 1.97%. This result suggested that the filling yarns were more heavily weighted, resulting in lengthwise cracks and splits.
3.2 Yarn Tenacity and Elongation
The warp yarn tenacity and elongation means for the new and historic silk fabrics within type (cultivated or wild silk), age group, and weighting group are given in table 3. The new silk habutai (age group 1) had the highest mean tenacity (3.1 gf/den.) compared to the historic silk fabrics (age groups 2–9). In general, yarn strength progressively decreased with age. However, the tenacities for the individual historic silk fabrics within age groups varied considerably, and two of the silk samples had mean tenacities exceeding that obtained for the new silk habutai. Four of the historic silk fabrics had warp yarns that were too weak to be raveled from the fabric without breaking, resulting in tenacity values of 0.0 gf/den. The amount of tin weighting in three of these fabrics was in excess of 3.0%. The fourth sample was an unweighted silk from a Spanish War banner that had had excessive light exposure. As was expected, less variability in the tenacity values was obśerved for the newer unweighted silk fabrics (i.e., age groups 1 and 2) than for the older silks.
Table 3 Warp Yarn Tenacity and Elongation Means for Silk Fabric
However, generalizations are limited by 1) the unequal number of samples within silk type, age group, and weighting group and 2) the variability in the strength and elongation data within age groups (especially for group 5) because of inherent differences in fiber strength and elongation, yarn construction characteristics, and previous histories (including processing, use, and cleaning, etc.). For example, too few weighted silk samples were available in age groups 1–3, 6, 8, and 9 to make comparisons between the unweighted and weighted silks or to make predictions about changes in strength of silk as a function of aging.
A regression analysis was performed on the yarn tenacity means to further examine the relationship between yarn strength and age. T-tests showed that the differences in the mean yarn tenacities for the nine age groups were significant at the 0.001 level. Orthogonal trend analysis indicated a linear downward trend with increasing age. When the tenacity means of individual silk fabrics were plotted on the regression line (see figure 1) only five weighted samples had means equal to or greater than the predicted values, supporting the premise that weighting has a negative effect on strength. In addition, all of the fabrics in age group 4 (1910–39) had tenacities below the predicted value of 1.92 gf/den., indicating that the samples in this age group had greater degradation than was expected based on the regression line.
Fig. 1.
A scatter plot and regression line relating yarn tenacity in cultivated silk fabrics to age group
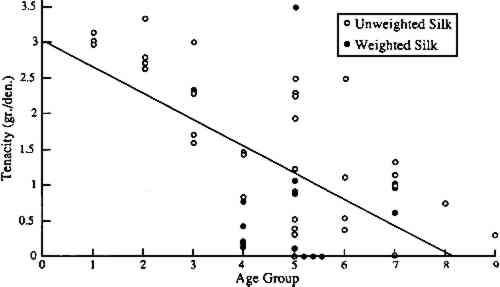 |
Comparison of yarn tenacity and NAA weighting results reveals an apparent disparity between strength of tin weighted and chrome weighted samples. Eleven of 13 silk samples containing combinations of tin and other metallic elements had tenacities of less than 1 gf/den. In contrast, one sample (C-37) that contained only 0.01% tin and 1.58% chromium had a tenacity of 3.5 gf/den., which is higher than the mean tenacity of the new silk. The other sample that contained chromium (2.38%) also contained 7.34% tin and 1.24% barium; however, warp yarns (C-20W) were analyzed separately and did not contain chromium.
There are too few weighted samples on which to base conclusions, but possibly different mechanisms may be working with tin and chromium weighting. It is generally believed that tin weighting is absorbed into the amorphous regions in the fibroin without bonding to the fiber. The high proportion of tin may interfer with the physical structure and integrity of the fiber, or tin may act as a photosensitizing agent, initiating phototendering or exacerbating oxidative degradation. In contrast, chromium may form a chromium-protein complex that could either tie up oxidative degradation sites or act as a crosslink between fibroin molecules. Further research is needed to determine the effect of different weighting agents on degradation of silk.
Differences also were observed in the elongation properties of the new and historic silk fabrics. The percentage elongation means associated with the nine age groups were significantly different at the 0.001 level. Results of the orthogonal trend analysis on the cultivated silk fabrics showed an inverse linear relationship between age and elongation (see figure 2), i.e., when age increased, elongation decreased. Similarly, the weighted silk fabrics exhibited less elongation than those that were unweighted. The elongation means for the unweighted silk fabrics were approximately twice as large as those recorded for the weighted silks.
Fig. 2.
A scatter plot and regression line relating yarn elongation in cultivated silk fabrics to age group
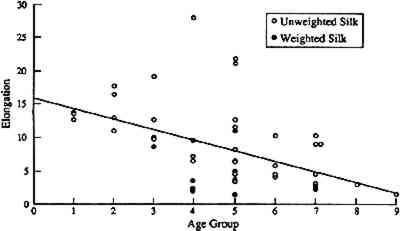 |
The percent elongation of warp yarn from the unweighted wild silk fabrics (25%) was substantially higher than that observed for the new and historic, cultivated silks. Lucas et al.10 reported that Tussah silk usually is weaker and more extensible than cultivated silk, because it is less crystalline and contains a larger proportion of long side-chain amino acids, which would contribute to greater extension under tension.
3.3 Dilute Solution Viscosity
Intrinsic viscosity tests were performed on only the unweighted, Bombyx mori silk fabrics. The other silk samples could not be tested because the weighting agents hindered dissolution and the weighting element mass altered flow times. Wild silks would not dissolve in the saturated LiBr solution. The mean viscosity of 3.1, obtained for the new silk habutai was significantly higher than the means obtained for the historic silk fabrics in age groups 2–9 which ranged from 0.7 to 1.4 (see table 4). Within specific age groups, only one historic silk sample had a viscosity number above 2.0; the majority had viscosities of <1.0. Because of the limited number of samples within specific age groups, a greater number of samples would need to be tested to obtain a better appreciation of the relationship between fiber aging or degradation and changes in viscosity.
Table 4 Intrinsic Viscosity Means for Unweighted, Cultivated Silk Fabrics
3.4 Amino Acid Analysis
The mean weights of the 17 amino acids (per 100 g of sample) that were detected in the unweighted, cultivated and wild silk fabrics are presented in table 5. The weighted silks were not analyzed because of the possibility of contaminating the analyzer's ion exchange column. In general, few differences were observed among the age group mean weights for specific amino acids within the two silk types (cultivated or wild silk). Compared to the samples containing wild silk, however, those that contained cultivated silk had somewhat larger amounts of the simpler amino acids (glycine, alanine, serine, and valine). Lucas et al.10 reported that glycine, serine, and alanine are the major amino acids in the crystalline portion of the fibroin, whereas the bulky side-chain amino acids are found in the amorphous regions. Since cultivated silk is more crystalline than wild silk, a larger amount of the simpler amino acids would be expected.
Table 5 Amino Acid Analysis of Unweighted Silk Fabrics
The amount of the specific amino acids present in the silk samples varied within age group. For example, in age group 5, the amount of glycine present in the 10 samples ranged from 27.1 to 34.0 g per 100 g of sample. However, in only one of the samples within this age group was the amount of glycine less than 29.0 g. This was a rather unusual sample because the yarns were coated with a clear substance. This sample also contained a detectible amount of arginine (5.9 g) not present in the other cultivated silk samples, as well as higher proportions of proline, methionine, isoleucine, leucine, and phenylalanine. The coating may have been a protein substance, which would account for the variation in proportions of amino acids in this sample. All of the wild silks, on the other hand, contained between 4.4 g and 14.8 g of arginine.
Because of the unequal number of samples within age groups and the variability among the samples from previous history and environmental conditions, further study is needed to determine the influence of aging on the amino acid content of silk. However, amino acid analyses provide an exciting research avenue for studying chemical changes in silks.
3.5 Optical and Scanning Electron Microscopy
Optical microscopic examination of all silk samples was done to evaluate their overall physical deterioration. Scanning electron micrographs were taken of selected samples to assess fiber fracture patterns by comparison to those characterized by Hearle6 and Bresee and Goodyear.4 Ten types of fiber fracture patterns were identified in the historic silks, some of which are shown in figure 3. Slightly less than half of the fiber fractures were combinations of two or more type of patterns, mostly granular and axial fracture types. Also widely observed were granular fractures resulting from transverse discontinuities within the fiber, �s well as axial splits with long tails related to cracks and surface flaws. Other fracture patterns observed to a lesser extent were axial angular splits, V-shaped fiber fractures, and fibrils or fibril bundles. Many of the heavily tin-weighted fabrics had broken filaments with smooth fiber ends. The great variety of fracture patterns observed among the silk samples reflected the diversity in histories and factors that contribute to silk aging.
Fig. 3.
SEM photomicrographs of silk fiber fractures. A: granular fracture showing transverse discontinuities within the fiber; B: combination fracture exhibiting internal voids and fibrils; C: combination of axial split with granular fracture; and D: rounded fiber ends
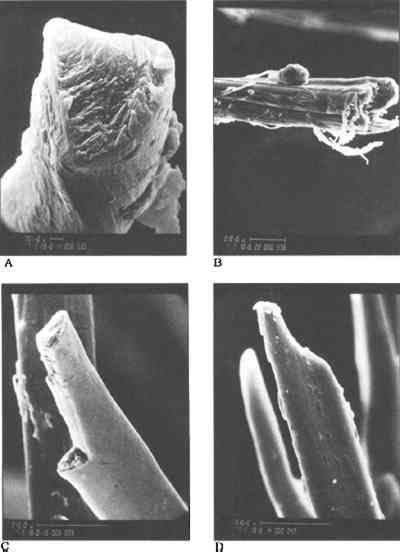 |
3.6 Photoacoustical Infrared Spectroscopy
The major, characteristic, infrared absorption bands or peaks as identified by Asia et al.2 were present in both the new silk habutai and in historic silk samples C-49 (200 years old) and C-22 (400 years old), as shown in figure 4. For example, the absorption band at 3300 cm−1 is attributed to N-H stretching of hydrogen bonds (i.e., −NH…O=C−). The absorption band at 1640 cm−1 is associated with C=O stretching vibrations. However, the infrared spectra of the chromium- and tin-weighted silks differed from that of the unweighted silk (figure 5). Some of the major absorption bands were rounded and/or flattened, indicating appreciable changes in chemical structure.
Fig. 4.
Infrared spectra of unweighted silk fabrics. A: new; B: 200 years old; and C: 400 years old
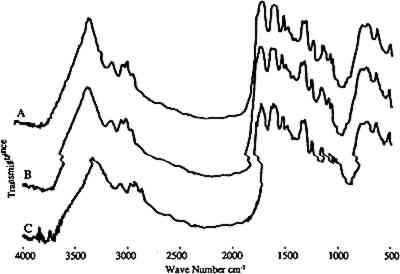 |
Fig. 5.
Infrared spectra of unweighted and weighted silk fabrics. A: unweighted new; B: tin weighted; 60 years old; C: chromium weighted, 80 years old
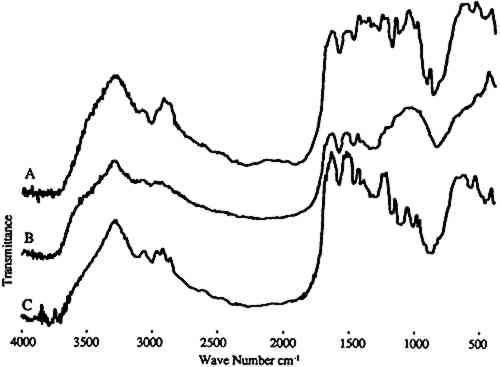 |
4 CONCLUSIONS
FIFTY-FOUR HISTORIC SILK FABRICS (10 to 400 years old) were evaluated and compared to new silk to determine the extent of degradation in relationship to the type of silk (cultivated [Bombxy mori] or wild [Tussah]), presence of weighting agents, and age. The silk samples, obtained by donations from universities, museums, and private collectors, varied in fabric count, denier, amount of twist, metallic elements present, and general condition (poor to excellent).
Of the 54 undyed, historic silk fabrics, 15 contained 1% or more total weight of metallic elements and were classified as weighted. All of the silk samples contained small amounts of iron. Other elements detected by NAA in various amounts were tin, aluminum, chromium, copper, barium, and arsenic. Combinations of metallic elements ranged from 0.02% to 14% of fabric weight. Combinations of metallic elements present differed within and between age groups. Additional research on weighting agents is recommended using a combination of methods of elemental analysis to determine if the amount and type of weighting agents might be a potential method for dating silk fabrics.
The highest element percentages were obtained for tin, which was commonly used as a weighting agent in the late nineteenth and early twentieth centuries. Six of the fabrics from 1890 to 1909 and five of the eight fabrics from 1910 to 1939 were weighted with tin in amounts of 3% to 14%. Tin weighting at levels above 3% appeared to be particularly detrimental to silk, resulting in low yarn tenacities, ranging from 0.0 to 1.06 gf/den., and somewhat lower elongation. In general, tenacity, elongation, and viscosity were inversely related to the age of the silk, based on regression and orthogonal trend analyses. The tenacity means for the silks in age groups representing ≥ 50 years of age (i.e., 4–9) were significantly lower than that for the new silk habutai (intrinsic viscosity of 3.1). Similarly, silks older than 80 years showed a marked decrease in viscosity. The majority of the historic silk fabrics had viscosities of <1.0.
The amino acid content of the new and historic silk fabrics were similar; however, some of the samples exhibited a decrease in bulkier side-chain amino acids (proline, valine, methionine, lysine) with a corresponding increase in the simpler amino acids (glycine, alanine, and serine). As in the other methods of analysis, considerable variability existed in the amino acid content of particular samples within age groups because of inherent differences and previous histories. Overall, the samples containing wild silk had lesser amounts of glycine, alanine, and serine and greater amounts of the bulky side-chain amino acids.
The variety of fiber fracture patterns in the historic silk also reflected the diverse histories and causes of deterioration in the naturally aged samples. However, few differences were observed in the infrared spectra for the new and the historic silks, except for the weighted silk. Some of the characteristic absorption bands for tinweighted silk were flattened and less pronounced.
Visual assessment of the general condition of the fabric did not always correlate with tenacity, elongation, or viscosity. Most of the fabric samples that were classified as in poor condition had low strength, but in some cases, fabrics that appeared to be in good condition were so weak that the yarns could not be raveled out for testing. Not all weighted silks were crisp or stiff, nor were cracks or splits in fabrics always associated with weighted silk. Hence, visual examination may be inadequate to judge the condition of historic silk fabrics.
Because of the weighting practices and severe manufacturing treatments reported in textile trade journals from 1890 to 1939, silk fabrics from this period may be more fragile and readily damaged than much older silks. Neutron activation analysis and other analytical methods may provide important information pertaining to the amount and type of weighting agents present in historic silks that in turn, may have a significant influence on the rate and extent of fiber degradation. Additional research is needed on the synergistic effects of weighting agents and other aging factors on silk degradation, the effects of wet and dry cleaning on weighted silks, and possible methods of retarding degradation.
ACKNOWLEDGEMENTS
THE AUTHORS EXTEND THEIR APPRECIATION to the following faculty, staff, and graduate research assistants at Kansas State University: Jack Higginbothem, Department of Nuclear Engineering, for his assistance with the neutron activation analysis; Charles yang and John Graham, Department of Chemistry, for their assistance with photoacoustic infrared spectroscopy; Scott Nelson, Department of Grain Science, for the amino acid analysis; John Kirchma, Department of Entomology, for his expertise in scanning electron microscopy; and Dr. Kenneth Kemp, Department of Statistics, for his assistance in the statistical analysis.
REFERENCES
Annual Book of ASTM Standards. Philadelphia: American Society for Testing and Materials, 07.01 and 07.02.
Asia. M. M., M.Tsuboi, T.Shimanouchi, and S.Mizushima. “Infrared Spectra of Polypeptides and Related Compounds.”Journal of Physical Chemistry59 (1955): 322–325.
Bogle, M.“Artificial Weighting and the Deterioration of Silk.”AIC Preprints, Sixth Annual Meeting of the American Institute for Conservation of Historic and Artistic Works, Fort Worth, Tex. June 1978. 31–40.
Bresee, R. R., and G. E.Goodyear. “Fractography of Historic Silk Fibers.”Historic Textile and Paper Materials: Conservation and Characterization, Advances in Chemistry Series 212, edited by H. L.Needles, and S. H.Zeronian, Washington, D.C.: American Chemical Society, 1986. 95–109.
Higgenbothem, J.Instrumental Neutron Activation of Coal and Coal Fly Ash. Master's thesis, Kansas State University, 1983.
Hearle, J. W. S.“An Atlas of Fiber Fracture #1–15.”Textile Manufacturer99 (1972): 1164–1170.
Howitt, F. O.“The Structure of Textile Fibers: V—The Structure of Proteins—Silk.”The Journal of the Textile Institute. 40 (1949): 465–474.
Kuruppillai, R. V., S. P.Hersh, and P. A.Tucker“Degradation of Silk by Heat and Light.”Historic Textile and Paper Materials: Conservation and Characterization, In Advances in Chemistry Series 212, edited by H. L.Needles, and S. H.Zeronian, Washington, D.C.: American Chemical Society, 1986, 111–127.
Leene, J. E., L.Demeny, R. J.Elema, A. J.DeGraff, and J.Surtel. “Artificial Aging of Yarns in the Presence as Well as in the Absence of Light under Different Atmospheric Conditions.”Proceedings of the International Council of Museums13 (1958): 107–242.
Lucas, F., J. T. B.Shaw, and S. G.Smith. “The Silk Fibroins.”Advances in Protein Chemistry131958: 107–242.
Miller, J. E.A Comparative Analysis of Degradation in Naturally Aged and Experimentally Degraded Silk. Phd. dissertation, Kansas State University, Manhattan, KS (1986).
Needles, Howard, L. VickiCassman, and MarthaCollins, “Mordanted, Natural-Dyed Wool and Silk Fabrics: Light and Burial-Induced Changes in Color and Tensile Properties.” In Historic Textile and Paper Materials: Conservation and Characterization, Advances in Chemistry Series 212, edited by H. L.Needles, and S. H.Zeronian, Washington, D.C.: American Chemical Society, 1986. 199–210.
Scott, Walter M.“The Weighting of Silk,” Parts I–IV. American Dyestuff Reporter20 (1931): 517–518, 539–540, 543, 557–562, 591–594, 621–622.
Swiss Standards Association. “Silk Viscosity Testing Using Lithium Bromide.”Textil Rundscheu19 (1964): 80–83.
Tyler, A. G.“Survey of Silk Weighting.”Textile Journal of Australia24 (1949): 404, 406–407, 464.
Yang, C. Q., and W. G.Fateley. “The Effect of Particle Size on Fourier - Transform Infrared Photoacoustic Spectra.”Journal of Molecular Structure146 (1986): 25–39.
Section Index |