THE OZONE FADING OF TRADITIONAL NATURAL ORGANIC COLORANTS ON PAPER
Paul M. Whitmore, Glen R. Cass, & James R. Druzik
ABSTRACT—This report details the results of an ozone exposure experiment performed on a large number of traditional natural organic colorants applied to watercolor paper with no binder. These colorants were exposed in an environmental chamber to an atmosphere containing 0.397 � .007 parts per million (ppm) ozone at 72�F and 50% RH in the absence of light for 12 weeks. This ozone concentration is typical of that found in the Los Angeles atmosphere during a heavy smog episode. The total ozone dose delivered to the samples is equivalent to about four years of exposure to outdoor air in Los Angeles or to about eight years inside a typical air-conditioned building in Los Angeles.The ozone sensitivity of these colorant systems was evaluated by monitoring the color changes which occurred during the exposure. Almost all colorant systems tested showed some degree of fading after ozone exposure, and a few of these (curcumin, dragon's blood, indigo, and madder lake) should be considered very ozone-fugitive.
1 INTRODUCTION
RECENT STUDIES HAVE demonstrated that significant amounts of ozone (O3) present in the outdoor atmosphere can invade a museum environment.1,2 This chemically reactive gaseous air pollutant could prove hazardous to works of art and cultural property, for the damage to organic materials, particularly certain polymers,3 textiles,4 and textile dyes,5 from prolonged ozone exposure has been well documented. Little of this previous work, however, has been concerned specifically with the action of ozone on artists' materials; thus, the extent of the danger to museum collections and works of art has not been fully explored.
In an effort to identify those artists' materials which are vulnerable to ozone attack, our laboratory is currently engaged in a series of chamber exposure studies to determine the colorfastness of artists' pigments exposed to an atmosphere containing ozone. In the first exploratory experiment, reported elsewhere,1 a small number of modern watercolors on paper, representing a variety of organic and inorganic pigments, was exposed to ozone. Subsequently, a second experiment tested the ozone resistance of a more complete selection of modern artists' watercolors (from the Windsor & Newton line) containing organic pigments.6 These two investigations succeeded in targeting the synthetic alizarin lakes and a lake of Basic Violet 14 (a triphenylmethane dye) as being extremely reactive towards ozone.
Almost all the pigments used in modern artists' paints are man-made chemicals, developed in many cases as substitutes for rare, expensive, or unstable natural colorants of plant, animal, or mineral origin. Since works of art which pre-date the development of modern synthetic colorants were produced using these traditional pigments, the pollution resistance of natural coloring agents has a direct bearing on the preservation of museum collections.
This report describes a chamber exposure experiment performed to test the ozone sensitivity of a number of traditional natural colorants. Pigment samples were obtained from collections at the Harvard University Art Museums, the Balboa Art Conservation Center, and the Los Angeles County Museum of Art. The pigments were applied to watercolor paper, and color measurements were made instrumentally at intervals during the exposure test. The samples were exposed at 72�F, 50% RH, and in the absence of light, to 0.40 parts per million (ppm) ozone for 12 weeks. This average ozone concentration, although several times greater than that normally encountered in an indoor environment, was nevertheless within the range observed during a heavy smog episode in an outdoor metropolitan setting.7 The total ozone dose during the 12-week exposure was equivalent to about four years of exposure to outdoor air in Los Angeles, or to about eight years inside a typical air-conditioned building. The relative ozone sensitivity of the natural colorants was determined on the basis of the rate and severity of the color changes that occurred during this ozone exposure.
2 EXPERIMENTAL
THE AUTHENTICITY OF THE natural pigments obtained from museum collections was tested by a variety of analytical techniques. Solubility tests and color reactions8–11 were usually performed first, for not only did these tests provide a great deal of information (often sufficient for a definitive identification), but the solutions generated in these tests could also be used in subsequent analyses. Transmission spectra of pigment solutions were recorded in the 380 nm–700 nm range on a Diano Match Scan II spectrophotometer and were compared to literature results12 or to spectra of reliable samples obtained from suppliers of artists' materials (Windsor & Newton, Fezandie & Sperrle, Cerulean Blue, A. F. Suter Co.), chemical supply houses (Aldrich, Sigma), and from Prof. Helmut Schweppe. Thin layer chromatography on polyamide plates was performed according to published procedures,11 and comparison to authentic reference samples permitted differentiation of closely related colorants, such as cochineal and lac lakes, or various yellow lake pigments derived from different plants. The results of these analyses indicate that most of the pigments tested which bear the names of natural colorants were genuine. Only these natural colorants whose chemical compositions could be established were included in the ozone exposure test. The complete list of the natural organic colorants tested is shown in Table 1, along with the Color Index designation for each. Also shown are the number of different examples of each colorant which were tested. These multiple samples reportedly represented various shades, manufacturers, or dates of manufacture for a single coloring agent, but this information could not be substantiated in most cases.
Table 1 The traditional natural organic colorants tested in this experiment. Also shown are the Color Index designation and the number of different examples of each colorant.
Because of the limited availability of many of the natural colorants tested, a technique was developed which allows preparation and study of very small (milligram) quantities of pigment on paper in the absence of any binder. A suspension of pigment in about 1 ml of volatile solvent was airbrushed (Iwata HP-A) onto a 1″ � 2″ piece of watercolor paper (cut from 22″ � 30″ sheets of Arches 140 lb. hot-pressed paper) through a stainless steel mask with a 1 cm diameter hole. This produced an even, matte 1 cm spot in the center of the paper, and the pigment particles were embedded into the paper securely. Repeated color measurements of a number of control samples were made prior to exposure to verify that routine handling did not produce a measurable color change due to loss of pigment from the samples. (Four colorants—curcumin, dragon's blood, gamboge, and saffron—were applied as dye solutions rather than as pigment suspensions.) An effort was made to produce samples whose reflectance at the wavelength of maximum absorption was about 40%, for at this initial reflectance the color change has been found to be most sensitive to changes in the colorant concentration.13
Reflectance spectra were measured using the Diano Match Scan II, with the spot size of the light beam limited to 7 mm using the small-area-view option. This small sample size reduces the signal-to-noise ratio of a measurement and also increases the sensitivity to inhomogeneity of the pigment samples, so a jig was made to hold the paper samples in a reproducible position in front of the sample port of the spectrophotometer. This allowed precision of a few percent in reflectance measurements, or an uncertainty in ΔE of less than about 1 unit. The instrument was calibrated with a standard white tile (referenced to an NBS standard) and, owing to the translucence of the paper samples, this tile was used as a backing during reflectance measurements. The calibration and reflectance spectra were all recorded with the specular beam excluded. Reflectance measurements were made at 2 nm intervals from 380 nm to 700 nm and were stored on floppy disks. Chromaticity coordinates and color differences (using the CIE 1976 L∗a∗b∗ formula) were calculated for the CIE Illuminant C.14 Munsell color notations were subsequently calculated from these chromaticity coordinates.15
The ozone exposure apparatus has been described in detail elsewhere.1 Briefly, an airstream was pumped through a series of filters to remove existing pollutants. Following humidification, the air flow was irradiated by ultraviolet light from a mercury vapor lamp housed in a commercial ozone generator (Ultra Violet Products SOG-2) and then admitted to the exposure chamber through a perforated Teflon tube. The ozone concentration inside the chamber was measured continuously with a UV photometric ozone monitor (Dasibi Model 1003 AH), and a computer interfaced to the ozone monitor automatically collected and stored the average ozone concentrations during the course of the exposure.
Samples of all the colorants on paper were prepared and mounted on anodized aluminum panels. These panels were hung in the exposure chamber adjacent to the chamber walls, with the samples facing the interior of the box. The colorants were exposed to 0.397 � .007 parts per million (ppm) ozone at 72�F and 50% RH in the absence of light for 12 weeks. The samples were removed and reflectance spectra recorded after 1, 2, 3, 4, 6, 8, and 12 weeks of exposure.
3 RESULTS
THE FADING OF AN OZONE-SENSITIVE colorant system can be characterized by the change in its visible reflectance spectrum upon exposure to ozone. Figure 1 illustrates the evolution of the reflectance spectrum of indigo on paper during its 12-week reaction with ozone. Indigo absorbs light across the visible spectrum, giving the indigo/paper system a bluish gray appearance. As the colorant is consumed (and presumably decolorized) by ozone, the reflectance across the visible spectrum increases, and the sample appears a lighter gray (i.e., faded). In this example, the wavelength of maximum reflectance also shifts from 450 nm (blue) to 500 nm (green) during the ozone reaction.
Fig. 1.
Reflectance spectrum of the indigo/paper system at several stages during its exposure to 0.40 ppm ozone at 72�F, 50% RH, in the absence of light.
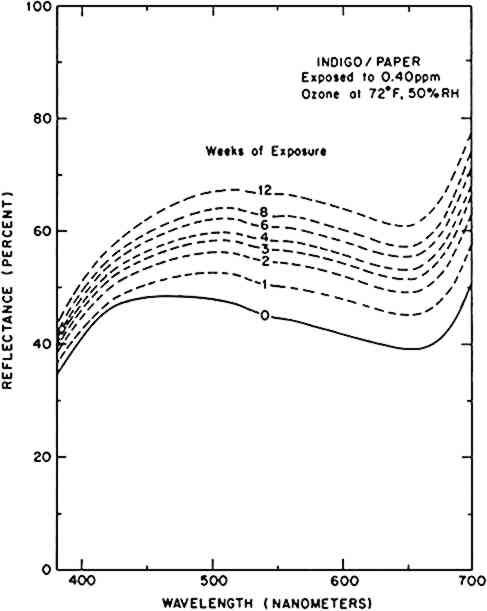 |
Although the reflectance spectrum contains all the information necessary to characterize the color during an ozone reaction, it is not the most convenient means to quantitatively assess or compare complicated color changes, which could involve shifts in hue as well as lightness. It is common, then, to reduce the spectral information to a set of parameters according to one of several conventions adopted for describing color.14Table 2 utilizes several of these methods to characterize the color under CIE Illuminant C during the ozone exposure of the indigo/paper sample whose reflectance spectrum is shown in Figure 1. Two frequently used color notations are the CIE tristimulus values (X, Y, Z) and the CIE chromaticity coordinates and luminous reflectance (x, y, Y). The chromaticity coordinates (x, y) also allow two-dimensional plotting and determination of such aspects of color as the dominant wavelength and purity under a standard illumination source. Figure 2 illustrates this use, showing the trend toward green (under Illuminant C) as the predominantly blue indigo/paper sample fades toward the yellow hue of the plain paper substrate. (Under this standard illuminant, the plain paper substrate appears yellow, and its color remains unchanged throughout the exposure. The observed yellowing of the indigo/paper sample during ozone exposure probably arises, at least in part, from the optical mixing of the yellow paper and the blue indigo. The possibility that some of this hue shift results from the generation of a yellow reaction product is the subject of further investigation.)
Table 2 Observed color change in indigo/paper system during exposure to 0.40 ppm ozone at 72�F, 50% RH, in the absence of light. CIE tristimulus values (X, Y, Z) and CIE chromaticity coordinates and luminous reflectance (x, y, Y) are calculated for CIE Illuminant C from the measured reflectance spectra. Munsell color notations (hue, value/chroma) are calculated from the tristimulus values. Color differences, ΔE, are calculated using the CIE 1976 L∗a∗b∗ formula.
Fig. 2.
Observed color change of the indigo/paper system during its exposure to 0.40 ppm ozone at 72�F, 50% RH, in the absence of light. The CIE chromaticity coordinates (x, y) for CIE Illuminant C are plotted, and the dominant wavelengths of the samples and plain paper are indicated.
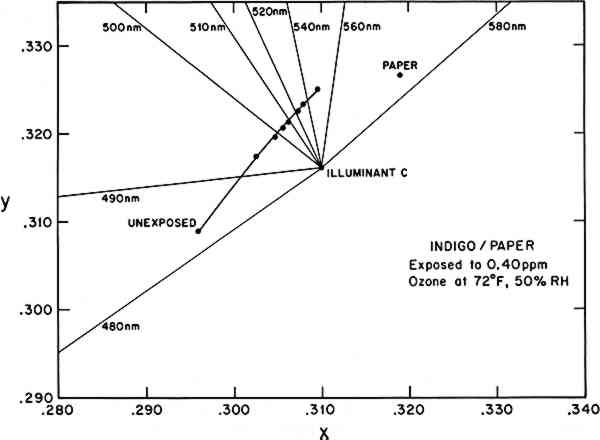 |
These parameters can also be used to calculate the Munsell notation for the color.15 This system denotes the color in terms of an average observer's perception of its hue, value (lightness), and chroma (saturation or purity). The Munsell description of the indigo/paper color change is easily interpretable in terms of its apparent shift in hue from blue toward green and an increase in its value or lightness. An added advantage of this approach is that a reported color can be visualized easily by referring to the appropriate color chips in the Munsell Book of Color.16
Finally, a single quantity representing the total perceived color difference between two specimens, combining changes in hue and lightness, can be calculated from two respective sets of tristimulus values.14 In this work the CIE 1976 L∗a∗b∗ formula has been used to determine the color difference, ΔE, of a colorant system before and after a given ozone dose, thus providing an approximate indicator of the magnitude or extent of the total color change caused by ozone reaction. (On the average, a ΔE value less than 0.5 is not perceptible, and a ΔE value less than 1 is usually considered a good color match.) The calculated color difference (vs. unexposed sample) during ozone exposure for the indigo/paper system is tabulated in Table 2 and illustrated graphically in Figure 3.
Fig. 3.
Observed color differences (versus unexposed sample) of the indigo/paper system during its exposure to 0.40 ppm ozone at 72�F, 50% RH, in the absence of light. Color differences, ΔE, were calculated for CIE Illuminant C from the measured reflectance spectra using the CIE 1976 L∗a∗b∗ formula.
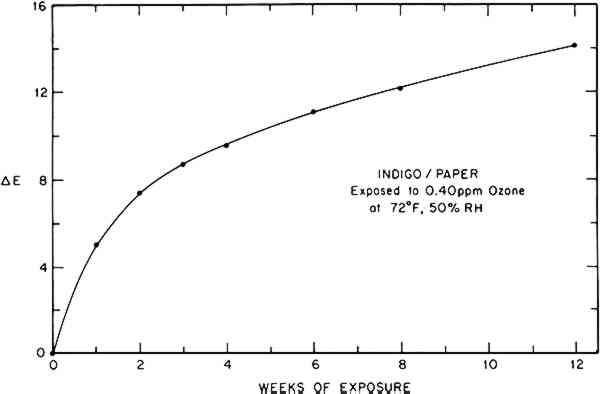 |
The results of the 12-week ozone exposure for the other natural colorant/paper systems are summarized in Table 3. Again, the reflectance spectra were measured, and the colors of the samples before and after exposure are described in terms of the calculated tristimulus values, chromaticity coordinates, Munsell notations, and color differences, ΔE. The time dependence of the color changes for all the colorant systems is shown in Figure 4. (For those colorants which were represented by samples from several sources, the results are reported for a typical example.)
Table 3 Observed color change of natural colorant/paper systems upon exposure to 0.40 ppm ozone at 72�F, 50% RH, in the absence of light. CIE tristimulus values (X, Y, Z), chromaticity coordinates (x, y) and calculated Munsell notations (hue, value/chroma) for CIE Illuminant C are reported before and after the 12-week ozone exposure. Color differences between exposed and unexposed samples are calculated using the CIE 1976 L∗a∗b∗ formula.
Fig. 4.
Plot of the observed color changes of natural colorant/paper systems during exposure to 0.40 ppm ozone at 72�F, 50% RH, in the absence of light. Color differences, ΔE, were calculated for CIE Illuminant C using the CIE 1976 L∗a∗b∗ equation. Measured color differences for the AATCC Gray Scale are also indicated for reference. Color changes are shown for a) very reactive, and b) moderately reactive, colorant systems (note different scales for vertical axes).
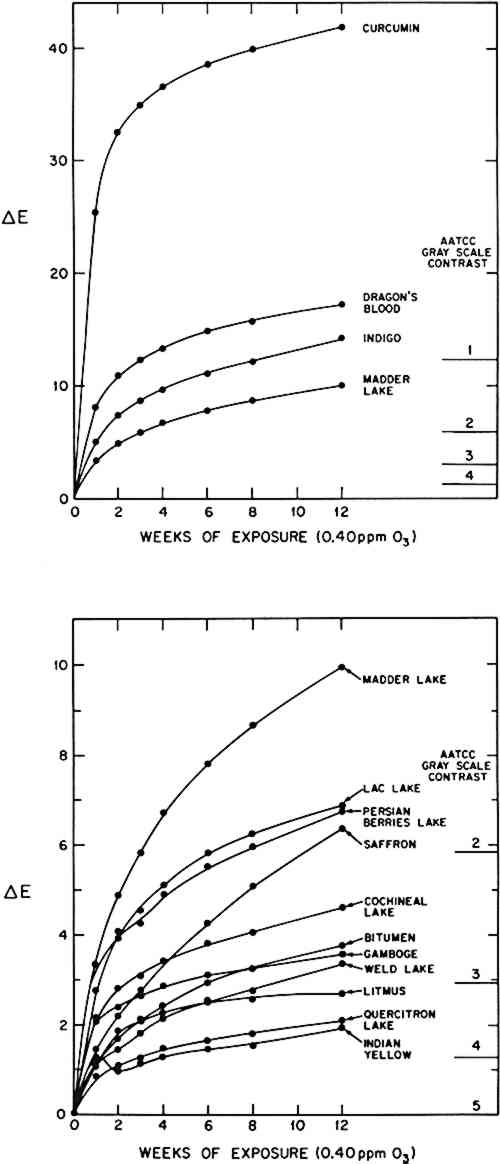 |
4 DISCUSSION
THE REACTION OF OZONE with organic materials has been widely studied17 and has found application in various processes ranging from organic syntheses to water sterilization. It has been determined that, for the most part, ozone attacks organic molecules at carbon-carbon double bonds, both in olefinic (straight carbon chain) and, more slowly, in aromatic (benzene ring) structures. This reaction oxidizes the organic molecule, ultimately cleaving the carbon atom framework at the site of initial ozone attack. Detailed studies of the mechanism of the ozone reaction with alizarin lakes18 and with indigo19 are presented elsewhere that illustrate the chain of events involved. Since these double bonds often form part of the chromophore in organic colorants, their destruction from ozone reaction usually leads to a loss of color. The observed sensitivity of the natural colorants in this study can be rationalized on the basis of this accepted scheme of ozone reaction.
However, the pertinent issue is not whether a particular colorant will react with ozone—if the colorant contains carbon-carbon double bonds, it will react—but rather, how quickly a colorant system will fade upon exposure to ozone at concentrations found in museum environments. The observed ozone fading of a colorant system is actually a very complicated process governed by a variety of factors. First among these is the chemical reactivity of the colorant towards gaseous ozone. This property depends on the molecular structure of the colorant, particularly on the number and types of chemical bonds and atomic sites vulnerable to ozone attack. Often the chemical reactivity of a colorant can be altered by processing during manufacture (with included additives or particle coatings, or by variations in particle shape or size); during application (through interaction with mordants or substrates, through control of the colorant concentration or degree of aggregation, and through the use of binders or coatings); or during the aging of a museum piece (which could experience extensive oxidation or other chemical degradation in reactions with light, the atmosphere, and pollutants). Finally, the color change produced upon ozone reaction of the colorant system is related in a complicated way to the colorant particle size, shape, concentration, and optical properties, as well as to the physical structure of the colorant system.
The thorough investigation of all these factors on the ozone fading behavior of museum pieces is beyond the scope of the present study. However, to the extent that these factors could be controlled during sample preparation, the physical structure of the colorant systems should be uniform enough to allow meaningful comparisons of the ozone sensitivity of the colorants. In addition, some colorant samples were available from several sources (see Table 1), and these materials, representing different manufacturers, formulations, or eras, were tested and compared. In nearly every case, different samples of a colorant demonstrated only slight variations in ozone reactivity, suggesting that the formulation, particle size distribution, and aging history probably played relatively minor roles in determining the ozone resistance of the colorant systems in this study. The sole exception to this trend was observed in the sample of “Burnt Madder Lake,” which, unlike the other madder lakes, was practically inert towards ozone (ΔE < 1 after 12 weeks). The reason for this lack of reactivity is unclear, but it could be the result of prior oxidation of the pigment in a charring process,9 which might render the product less susceptible to further oxidation by ozone.
The results of this exposure test are summarized in Table 4, in which the most ozone-sensitive colorants on paper have been identified on the basis of their (average) rates of fading and the extent to which they had faded after the 12-week ozone reaction. Since the ozone exposure was relatively mild and of short duration (representing an ozone dose equivalent to only a few years inside a museum), it is possible to differentiate only among the most reactive colorant systems. The time dependence of the ozone fading, shown in Figure 4, was qualitatively the same for all the reactive colorant systems. In these cases, rapid fading began almost immediately upon exposure to ozone, with the largest color changes (as measured by ΔE) occurring during the first week. Subsequent weeks of ozone exposure produced successively smaller color changes. By the end of 12 weeks, most of the colorants are fading at a rate that if extrapolated to longer exposure times would still not alter the relative rank ordering among the colorants tested based on total color change (ΔE) observed in response to a given ozone exposure. Because of this ordering of the time dependence of the ozone fading, the colorant systems can be grouped according to the extent of fading after 12 weeks into rather arbitrary categories of Very Reactive (ΔE > 9 after 12 weeks at 0.40 ppm ozone), Reactive (ΔE = 3 − 8 after 12 weeks), and borderline cases (ΔE = 1 − 3 after 12 weeks but with a monotonic increase in ΔE value over time). The remaining colorant systems showed no definite color change after the 12-week exposure and are termed “Unreactive” towards this ozone dose.
Table 4 Classification of the natural colorant/paper systems according to their observed fading during the 12-week exposure to 0.40 ppm ozone at 72�F, 50% RH, in the absence of light. (See text for the criteria upon which these categories were based.)
The interpretation of the observed fading rates in terms of the chemical reaction kinetics requires knowledge of the relation between the measured spectral reflectances and the concentrations of the colorants in these reactive systems. This connection has been made previously in the study of acrylic paint layers13 and glazes20 using a computer color-matching formula based on a simplified Kubelka-Munk analysis. However, the application of such an approximate theoretical treatment based on a model of a thick homogeneous paint layer, having smooth boundaries and whose color changes only from reduction of the colorant concentration, is inappropriate for the analysis of the ozone fading of the colorant systems in this study. The optical absorption and scattering properties of these samples, comprised of pigment particles embedded to some depth in the paper, are very complex compared to those of a thick, well-characterized paint layer. Further, by its nature the decolorization of the samples by ozone reaction involves alteration of the pigment particles themselves (not just their concentration), possibly leaving the particles coated with colorless ozone reaction products (which would alter their optical properties), or distorting and eventually decreasing the pigment particle size distribution. These effects will in turn change the optical response of the colorant system in a very complicated way throughout the course of the ozone exposure. Clearly, a more complete theoretical formalism and more thorough characterization of the colorant systems are needed before the chemical kinetic details of the ozone reaction can be inferred from color measurements alone.
For now, then, the ozone fading results are presented to illustrate the effect of prolonged ozone exposure on the appearance of these colorant systems. The apparent slowing of the observed ozone fading of these freshly prepared colorant samples suggests the possibility of very different ozone fading kinetics for older works of art, which may have experienced substantial oxidation due to atmospheric or photo-oxidation over the years and in which the rapid initial reaction (or reactions) may already have been completed. Exposure testing of aged samples is necessary to explore these variables. Nevertheless, there is compelling evidence prompting the routine protection of works of art containing natural colorants from prolonged exposure to atmospheric ozone.
5 CONCLUSION
THE OZONE-INDUCED fading rate of a number of traditional natural organic colorants applied on paper has been examined. Samples were exposed in an environmental chamber to an atmospheric ozone dose equivalent to approximately six to eight years of residence in a conventional air-conditioned building in Los Angeles. Previous studies of the ozone sensitivity of modern synthetic artists' pigments showed that a large number of these materials were relatively stable towards an ozone dose of the magnitude employed here. By contrast, almost all of the traditional natural colorants tested in this work showed some degree of fading after exposure to ozone. This procedure was successful in identifying many natural colorant/paper systems which should be considered ozone-fugitive, and a few (curcumin, dragon's blood, madder lake, and indigo) which are very ozonesensitive. While these results are strictly applicable only to other similar colorant systems (such as watercolors or pastel drawings), at present this study should nevertheless be useful as a guide in the judgment of those natural colorants which, in other applications, warrant particular observation or precautions.
ACKNOWLEDGEMENTS
THIS RESEARCH WAS PERFORMED under a contract with the Getty Conservation Institute, and this article is based on a paper presented at the 14th Annual Meeting of the American Institute for Conservation in Chicago, Illinois, 21-25 May 1986. Natural colorants were made available with the cooperation of Eugene Farrell and Richard Newman of the Harvard University Art Museums, and Gary Wade Alden, of the Balboa Art Conservation Center. The Munsell conversion calculations were performed using a computer program supplied by Max Saltzman and Dr. Fred Billmeyer. Discussions with Mr. Saltzman were helpful in the spectroscopic analyses of the pigments. The authors also wish to acknowledge the assistance of Dr. Helmut Schweppe, whose advice and reference materials aided the thin layer chromatographic identification of the natural colorants.
REFERENCES
C. L.Shaver, G. R.Cass, and J. R.Druzik, “Ozone and the Deterioration of Works of Art,” Environmental Science and Technology17 (1983), 748–752
T. D.Davies, B.Ramer, G.Kaspyzok, and A. C.Delany, “Indoor/Outdoor Ozone Concentrations at a Contemporary Art Gallery,” J. Air Pollution Control Assoc., 31 (1984), 135–137.
K. E.Stephens, and C. L.Beatty, “Effects of Ozone on the Physical Properties of Butadiene and Styrene/Butadiene Copolymers,” Polymeric Materials Science and Engineering, 47 (1982), 277–281.
L.Jaffe, “The Effects of Photochemical Oxidants on Materials,” J. Air Pollution Control Assoc.17 (1967), 375–378.
J. C.Haylock, and J. L.Rush, “Studies on the Ozone Fading of Anthraquinone Dyes on Nylon Fibers,” Textile Research Journal, (January, 1976) 1–8.
K.Drisko, G. R.Cass, P. M.Whitmore, and J. R.Druzik, “Fading of Artists' Pigments due to Atmospheric Ozone,” in Wiener Berichte �ber Naturwis-senschaft in der Kunst, Bd. 2/3;, 1985/86, ed.A.Vendl, B.Pichler, and J.Weber. (Verlag ORAC, Vienna, 1985/86).
W. F.Hunt, T. C.Curran, R. B.Faoro, N. H.Frank, and E.Mask, National Air Quality and Emissions Trends Report, 1981, document EPA-450/4-83-011 (U.S. Environmental Protection Agency, Research Triangle Park, 1983).
F. W.Weber, Artists' Pigments (Van Nostrand, New York, 1923).
R. J.Gettens, and G. L.Stout, Painting Materials (Van Nostrand, New York, 1942).
“Colour Index,” 3rd edition, revised, Society of Dyers and Colourists, Bradford, Yorkshire, England, 1971, Vols. 3 and 4.
H.Schweppe, “Identification of Dyes on Old Textiles,” J. American Institute for Conservation, 19 (1980), 14–23.
F. W.Billmeyer, Jr., R.Kumar, and M.Saltzman, “Identification of Organic Colorants in Art Objects by Solution Spectrophotometry,” J. Chem. Educ. 58 (1981), 307–313.
R.Johnston-Feller, R. L.Feller, C. W.Bailie, M.Curran, “The Kinetics of Fading: Opaque Films Pigmented with Alizarin Lake and Titanium Dioxide,” J. American Institute for Conservation, 23 (1984), 114–129.
F. W.Billmeyer, Jr., and M.Saltzman, Principles of Color Technology, 2nd edition (John Wiley and Sons, New York, 1981).
“Standard Method of Specifying Color by the Munsell System,” Designation D 1535–80, American Society for Testing and Materials, Philadelphia Pennsylvania, 1980.
“Munsell Book of Color,” Macbeth Division of Kollmorgen Corp., Baltimore, Maryland, 1976.
P. S.Bailey, Ozonation in Organic Chemistry, Vols. 1 & 2 (Academic Press, New York, 1978 and 1982).
D.Grosjean, P. M.Whitmore, C. P.DeMoor, G. R.Cass, and J. R.Druzik, “Fading of Alizarin and Related Artists' Pigments by Atmospheric Ozone: Reaction Products and Mechanisms,” Environmental Science and Technology (submitted).
D.Grosjean, P. M.Whitmore, G. R.Cass, and J. R.Druzik, “Ozone Fading of Natural Organic Colorants: Mechanisms and Products of the Reaction of Ozone with Indigos,” Environmental Science and Technology (submitted).
R.Johnston-Feller, and C.Bailie, “An Analysis of the Optics of Paint Glazes: Fading,” in N. S.Brommelle and G.Thomson, Science and Technology in the Service of Conservation (International Institute for Conservation, London, 1982) 180–185.
Section Index |