FIBER IDENTIFICATION IN PRACTICE
Martha Goodway
ABSTRACT—Brief case studies of the problems in identification of a wide variety of ethnographic and archaeological fibers are given. The strategy of identification varied with the purpose (choice of treatment, assessment of damage or identification of its cause, or authentication) but most of all with the condition of the fibers. Fibers from ethnographic or archaeological sources tend to be aged, and are sometimes fragmentary or decayed, fossilized or charred. With fibers in such condition, the simpler methods of preparation for microscopic observation were found more successful than the classical biological methods of soaking, clearing and staining.Not all fibers could be identified. Fur fibers from characteristic areas of the pelt were usually diagnostic as to species as well as to genus. Vegetable fibers were often not mophologically specific to species. Unless “guide elements” were present, or special limitations on species distribution were known, the identification of the genus of a vegetable fiber was often the best that could be done. Instructions for an optical test for flax, and a report form for the observation of fur and wool fibers, are included.The identification of fibers in archaeological and ethnographic objects in practice is a great deal more difficult than the texts on fiber identification lead one to believe. For example, the solubility tests which are a mainstay of synthetic fiber identification are of no use since archaeological and ethnographic fibers are natural ones. The various chloroiodine stains, Herzberg's and others which stain cellulose red, violet or blue and ligno-cellulose yellow, seldom act on old and dessicated fiber unless at nodes or points of fracture. These and many other methods recommended for use with textile fiber identification often give equivocal results when applied to archaeological or ethnographic material.Experience has shown that complicated and lengthy preparation procedures do not repay the time and effort they require and, more importantly, often fail altogether. Perhaps the single most useful generalization that can be made about archaeological and ethnographic fiber identification is that not all fibers can be identified on the basis of the information we now have. Nevertheless, a surprising number of these fibers can be identified, or at least classified, by microscopical observation and a few simple tests. The following examples may suggest useful approaches in dealing with material of this sort.
IN PRACTICE, THE FIRST STEP in identification of natural fiber was to classify it by material, whether of animal or vegetable origin. To differentiate between cellulose and protein fiber where this was not obvious, an ash test detected protein by the characteristically nitrogenous odor of burnt hair. If it was not protein then the twist test quickly differentiated right-hand vegetable fibers such as flax and ramie, from the left-hand fibers such as hemp, jute, and most ethnographic fibers. Right-hand fibers were also distinguished from left-hand fibers microscopically, by a method described in the Appendix.
In the twist test, care was taken that only a single fiber was wetted so that the direction it rotated upon drying could be clearly observed. Flax, a right-hand fiber, was used as a comparison standard in these tests so as to establish the direction of rotation. Flax rotated swiftly on drying, but the left-hand fibers were often more subtle. If not repeated several times, the test could be misleading. An example of this occurred in examination of a pulley rope from the sunken warship Tecumseh, in which a red marking thread was found. The naturally colored fibers of the rope consistently gave a slow but distinct left rotation on drying, confirming their microscopical identification as manila hemp. The red-colored fibers, repeatedly wetted and dryed, gave contradictory results. When this fiber was also examined microscopically (Figure 1) it was obviously cotton. Cotton, a seed hair, has the form of a collapsed hollow tube. It collapses randomly, with a right-hand twist in one short section followed by a left-hand twist in another; this randomness of twist was faithfully reflected in the contradictory results of the twist test.
Fig. 1.
Collapsed-tube morphology and random twist characteristic of cotton fiber. 500x, 70� cross polars.
 |
With natural fibers, microscopy was the method of choice for identification because the morphology of these fibers can be distinctive. Unfortunately, the differences in morphology among some species are not always sufficiently distinctive to allow precise identification since nature tends to use the same repertoire of cells in constructing the fibers of different plants.
In mounting a fiber for microscopical observation, care was taken that adventitious material—such as cotton from clothing, or processed wood fibers from tissue—were not embedded with the sample in the preparation. Most fibers from objects already bore a large amount of extraneous material, which was distracting and could be misleading. It was also possible for an entire sample to consist of adventitious material. For example, a long, black animal fiber which had come from a cave being excavated proved to be a bristle from a brush used as a tool in excavation.
It has become customary to use a mounting medium such as Monsanto's Aroclor 5442, which is permanent and has an index of refraction about a tenth higher than that of natural fibers, whose indices in general are about 1.54 or 1.55, as a convenient method of enhancing contrast at the surface of the fiber. Some degree of contrast is essential so that surface detail becomes visible. These high-index media contain nasal irritants and when heated the vapours should not be inhaled, in addition to observing the rules for the use of PCBs imposed by the Environmental Protection Agency.
The most usual stem or bast fiber encountered was flax. Since linen was in common use before the spread of cotton, its identity is sometimes useful in problems of dating. In one such case a conservator was interested in the date of a repair to the joint of an 18th-century harpsichord stand, where a piece of cloth had been used as a filler. Flax fiber was identified, and the use of linen indicated to him, along with other observations, that he was dealing with an early repair. In another case, the presence of flax was useful in establishing the late date of a Plains Indian ceremonial object.
When fresh, stem fibers were generally more flexible than the fiber from leaves (hard fiber). Most vegetable fiber samples examined were left-handed. Of the commercial stem fibers, only flax and ramie are right-hand fibers. An optical method for determining the direction of the cellulose, whether it is right or left handed, mentioned by Luniak1 proved to be particularly useful, in addition to the observation of a fine lumen visible at the center and the cross hatchings called nodes, in identifying flax. See Plates 1–3 and the Appendix on the red plate test for details of this method.
Fig. .
Flax fibers in crossed polarized light. Note vertical fiber which is nearly totally extinguished (dark). 165x, 90� crossed polars.
 |
Fig. .
Red plate test of flax. Note the yellow color of the vertical fibre. 165x, 90� crossed polars, first order red compensator plate in 45� position.
 |
Fig. .
Red plate test of flax. Yellow fibre of plate 2 rotated 90� to horizontal position. Note blue color. 500x, 90� crossed polars and red plate.
 |
Silk from very early objects was usually in excellent condition. It was more recent silk, especially weighted silk of the 19th century, that showed signs of splitting in characteristic green-stick fracture (Figure 2). Silk that appeared in this condition was already recognized by the conservator as brittle, but cracks at the cross-over indentations of silk (Figure 3) were taken as early warning of a brittle condition, so these indications of incipient brittleness were searched for specifically. The cross-over indentations in silk fibers also gave a characteristic silhouette to silk (Figure 4) in addition to its triangular cross section.
Fig. 2.
Partial fractures in silk fiber, indicating brittleness. Green-stick fracture is characteristic of silk. Crossover indentation in lower fiber. 500x, 73� crossed polars.
 |
Fig. 3.
Partial fractures at a crossover indentation, an early indication of brittleness in silk. 500x, 71� crossed polars.
 |
Fig. 4.
A pair of crossover indentations characteristic of silk. 500x.
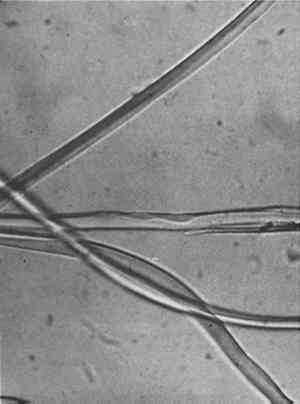 |
It is from such characteristic silhouettes that it was possible to classify fibers, if not always to identify them precisely, when they were charred or fossilized. Once charred, a fiber transmits no light, so only silhouette and size remained to be observed. It became routine to measure the width of every fiber as a check on tentative identifications. This dimension was included in all reports of microscopical observations, however brief.
If the material was a bast or leaf fiber, but not flax, there was a more difficult problem of identification and not one that could be solved in every case. These fibers could be particularly featureless. In such a case what in English are called “guide elements,” from the German “Leitelemente,” were searched for. These associated structures, such as parenchymatous cells or so-called baggy cells in tula ixtle, spiral vessels in sisal and some other agaves (Figure 5), stem hairs in esparto (Figure 6), and also crystals, such as oxalate crystals in hemp, were often diagnostic.
Fig. 5.
Spiral vessels characteristic of sisal and other agaves. These occurred in Tula ixtle. An example of a “guide element.” 200x.
 |
Fig. 6.
Stem hairs characteristic of exparto, shown here in cross section. Another example of a “guide element.” 325x.
 |
Cross-sections adequate for identification were prepared quite successfully without a microtome using equipment no more elaborate than cork, a C-clamp, and a razor blade. In practice a smooth, featureless area of the cork was chosen and the fibers were clamped between two pieces. Thin slices containing the fibers were then carefully shaved off. Sometimes this procedure was made easier if the fibers were glued to the cork first. The mounting medium itself was used as the glue. Hand-sectioning succeeded even with a single fiber of less than 15 μm diameter (Figure 7a and b). In most cases cross sections were not required because the cross section could be inferred by slowly focusing up and down upon the fiber at fairly high powers. This “optical sectioning” was a quicker and much less tedious procedure than cutting cross sections.
In the preparation of vegetable fibers the usual biological methods, such as soaking in order to separate individual cells, were either unnecessarily complicated or actually misleading. The cells from different comparison vegetable fibers tended to look very nearly alike. For example, cells of known specimens of agave resembled those of coir or of cotton. When applied to these separated cells, the red plate test also was not particularly informative. So-called “clearing” of thick vegetable fibers in methyl salicylate did render them more nearly transparent for microscopical observation, and was compatible with the Aroclors, for which methyl salicylate is a solvent.
Ethnographic samples were particularly difficult to identify because the necessary references in fiber atlases were wanting. Comparison material from herbarium samples in the National Herbarium, housed at the Smithsonian, were equally dessicated and as difficult to prepare as ethnographic material. The identification of the species of all but a few of the non-commercial vegetable fibers was not possible from their morphology. Matthews2 proved to be the single best source of the possibilities to explore. The situation as far as the scaled fibers—wool and furs—were concerned was, however, somewhat better.
The scales on wool or fur fibers were themselves an insufficient basis for identification, but the pattern could be used as confirmation. The scale pattern was best observed directly, using a high-index medium rather than taking a cast of the scales by pressing the hair into a film of dried but still plastic clear nail polish (Figure 8) or some other casting medium, then removing the fiber. The result of attempting a cast of a fiber which was not fresh was usually to pull the scales off the fiber, if not to break it altogether.
Fig. 7.
Cross section made by hand using a razor blade, with fiber clamped in cork. This example is vicuna, and is only 20 μm thick. 283x. a: end view; b: side view.
 |
Fig. 8.
Scale cast using clear nail polish. Photographed without cover slip. 500x.
 |
Keys for the identification of the fur of animals of specific geographical areas are listed in the Appendix on fur and wool fibers. The keys were useful outside their geographical limits for the larger species, which have fewer members but wider ranges. The use of a key suggested that the fur from a single pelt large enough to be used as a saddle pad was from a particularly large marten found in the West, the Mustela caurina(Figure 9). This is a marten so uncharacteristically large for its genus that at first an expert on mammals failed to name it as a possibility. The keys correctly directed attention to the mustelidae, which was confirmed by the expert once he saw the entire pelt. The keys had several limitations. They were usually based upon the longest guard hair, occurring only at the midline of the back. Another limitation was that the underfur of most animals is closely similar. Color was sometimes a clue, but burial tended to stain fibers various shades of brown.
Fig. 9.
Guard hair of Mustela caurina, a large western marten. 283x, 70� crossed polars.
 |
Sometimes when attempting fiber identification it is possible to identify the dye as well. For example, in an early Spanish writing box from Peru pieces of dark blue wool textile were discovered jammed under its lock escutcheon, which was slightly loose. The cloth had been put there apparently to keep the lock from rattling. The wool was very fine. The scales were ashed, using a red-hot dissecting needle on a short length of the blue wool fiber while it was resting on a microscope slide. The scales were separated by this procedure, and quite unexpectedly a blue haze condensed on the cold glass slide (Figure 10). This condensate suggested that the blue dye might be indigo, since indigo sublimes at temperatures above 300�C. As confirmation, another blue fiber was bleached in nitric acid and the resulting orange crystals were identified as isatin by their optical characteristics. The fiber itself (Figure 11) was differentiated from sheep wool by its even pigmentation, faintness of scale pattern, scarcity of medulla, and a round rather than oval cross section, all characteristic of camel hair. It is easy to be misled by current-day criteria of value and utility in projecting the possible identities of a fiber sample. This wool sample came from one of the South American camels, the vicuna, and a material one would not expect to find used in so offhand a way. Also possible are mixtures of fibers, and of later mending materials.
Fig. 10.
Condensed indigo, sublimed from ashed fiber of dyed vicuna. 433x.
 |
Fig. 11.
South American camel wool (vicuna), bleached of indigo dye in nitric acid. 283x.
 |
Sometimes microscopical examination revealed insect damage (Figure 12). In other cases the insects were exonerated, such as those blamed for broken fibers lost from Admiral Byrd's parka. It was thought to have been made from seal skin, but the fiber was not from the seal but from the caribou (Figure 13). Though perishable, because its very thin cortex results in a brittle fiber, it was an excellent choice for a parka because caribou fur is in fact warmer than seal.
Fig. 12.
Damage to horsehair by carpet beetles, typical of insect damage. 17x.
 |
Fig. 13.
A very thin cortex is typical of the fur of the deer family. This example is caribou. 500x.
 |
Several quite different categories of fiber are barbed. For example, the dandelion is barbed with the barbs alternating on each side of the fiber (Figure 14). It differs from feather fibers, where the barbs are opposite each other. Thus opposing barbs on fibers in a thread described as “mammal yarn” from a tapestry from Spiro Mound, Oklahoma (Figure 15) indicated the use of feathers. Until this sample was examined microscopically, feathers spun into thread had not been identified in Indian textiles east of the Pacific Northwest.3
Fig. 14.
Dandelion fiber, with alternating barbs. 500x.
 |
Fig. 15.
Feather fibers, with opposing barbs. From thread of tapestry, Spiro Mound, Oklahoma. 500x.
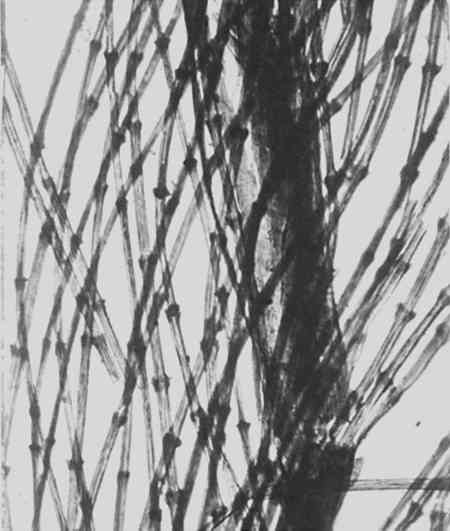 |
The most frustrating were samples from excavations in which the fiber appeared intact but whose internal structure had begun to disintegrate. Fibers of this description were excavated in Labrador from a level just above the permafrost. No matter what the magnification, their microstructure could not be brought into focus, and it seemed that the microscope would require a major realignment. When a preparation containing fresh comparison material was observed, however, the fresh material came into sharp focus. Apparently the excavated fibers had decayed to the point where the microstructure had lost its definition so that in effect it was no longer there. Excavated cotton fiber has also been observed whose surface was so distressed that it had an appearance very similar to the surface scales found on wool or fur fibers, except that those of wool or fur would have been more regular in pattern.
These examples demonstrated some of the problems encountered when attempting the identification of fibers from archeological or ethnographic materials. Even where these fibers eluded precise identification, it was possible to make a general classification and an assessment of their condition which made the effort to identify them worth while.
REFERENCES
BrunoLuniak, Identification of Textile Fibres. Qualitative and Quantitative Analysis of Fibre Blends, London1953, p. 123; A.Herzog, Textile Forchung4 (1922):58.
R.Mauersberger, editor, Matthews' Textile Fibers, sixth edition (New York1954).
Mary ElizabethKing and Joan S.Gardner, “The Analysis of Textiles from Spiro Mound, Oklahoma”, in Anne-Marie E.Cantwell, James B.Griffin, and Nan A.Rothschild, editors, The Research Potential of Anthropological Museum Collections, Annals of the New York Academy of Sciences376 (1981):123–139.
APPENDIX
1 APPENDIX
1.1 THE RED PLATE TEST
LUNIAK, FOLLOWING HERZOG, suggested the possibility that the direction (right- or left-hand) of cellulose could be determined optically using a polarizing microscope.1 Specifically, he states:
For the differentiation of flax and hemp fibres their opposite behaviour in polarized light between crossed Nicol prisms after insertion of a selenite plate Red I in the 45� position may be noted. Flax fibres display addition colours when parallel to the plane of the polarizer, and subtraction colours when at right angles; hemp fibres vice versa. A part of the fibres may be neutral; in general the differences are not so pronounced with hemp fibres. Comparison with known samples is advisable.
In order to put this suggestion into practice it is necessary to have a polarizing microscope with a compensator slot and a first-order, so-called red plate. Actually, the color of the field of view when the polars are crossed and the red plate is inserted in the compensator slot may be more accurately described as magenta. It is more convenient if the microscope, as do most polarizing microscopes, has a rotating stage.
Since bast and leaf fibers are polycrystalline they do not behave optically as neatly and unequivocally as a single crystal, and complete extinction under crossed polars does not occur in these fibers. For this test an individual fiber is more likely to give a definite response than a bundle of fibers, but not all individual fibers are good subjects. Colors under the red plate often cannot be observed when the fiber is in the 45� position which gives maximum brightness under crossed polars alone. To yield diagnostic colors when the red plate is inserted, the fiber should be observed first under crossed polars in a “nearest-to-extinction position” (since they do not extinguish completely) of 0� or 90� that is, the fiber should be parallel to the optical direction of either the polarizer or analyser. Rather than as an extinction position, this might be described better as the position in which the fiber appears most gray, or darkest.
The fiber to be identified must be observed first without the red plate in order to locate exactly an area of the fiber most nearly totally extinguished by crossed polars (Plate 1). When the red plate is inserted, areas that were black under crossed polars become either yellow or blue (Plate 2). If the stage, with the slide, is then rotated 90� the other color should appear, blue where it was yellow or yellow where it was blue (Plate 3). The colors depend on the orientation of the polarizer, the analyzer, and the red plate. Therefore each time this test is used, the orientation of the optical elements should be determined by using a comparison standard known to be flax. A left-hand fiber will give the same colors but each in a direction 907deg; from that or flax or ramie. In some cases the color shift will not be very distinct. That is why locating those areas of the fiber which are darkest under cross polars before the red plate is put in, and using only those areas for identification is so important.
Fig. .
FUR AND WOOL FIBERS, REPORT OF MICROSCOPIC OBSERVATION
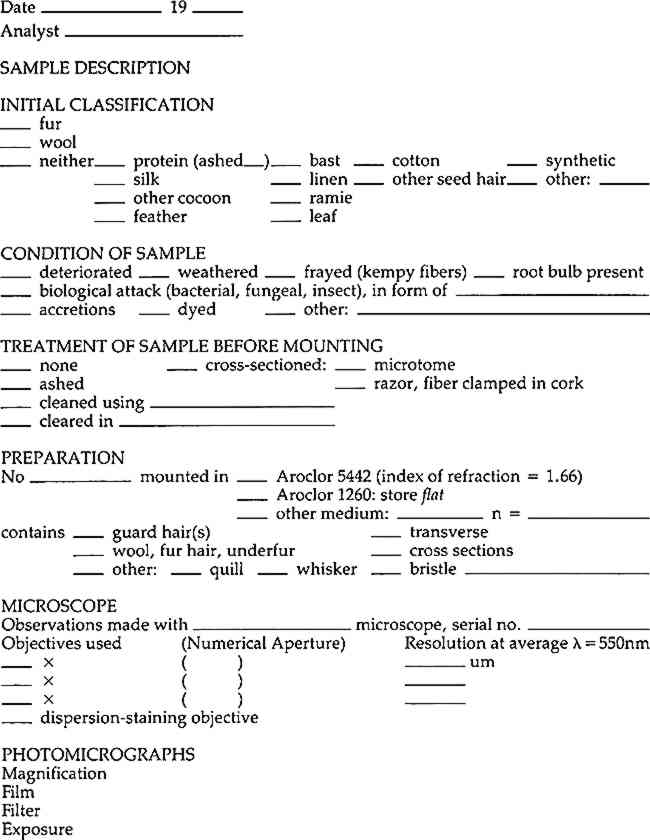 |
1.2 IDENTIFICATION FROM
- Appleyard, H. M., Guide to the Identification of Animal Fibres, second edition, Leeds 1978.
- Martin Brown, F. “The microscopy of mammalian hair for microscopists,” Proceedings of the American Philosophical Society 85 (1942) 250–274.
- Brunner, Hans and Coman, Brian J., The Identification of Mammalian Hair, Melbourne 1974.
- Hausman, L. A. “Structural characteristics of the hair of mammals,” The American Naturalist 54 (1920) 496–523.
- Hicks, John W., Microscopy of Hairs, A Practical Guide and Manual, Washington 1977.
- Luniak, B., The Identification of Textile Fibres, London 1953.
- Mathiak, Harold A. “A key to the hairs of the mammals of southern Michigan,” Journal of Wildlife Management 2 (1938) 251–268.
- Mauersberger, R., editor, Matthew's Textile Fibers, sixth edition, New York 1954.
- McCrone, Walter C. and Delly, John, The Particle Atlas Edition Two, volume II, Ann Arbor 1973, articles 39, 40, 43, 44, 45, 46, 47, 48, 49, 50, 51, 52, 53, 54.
- McCrone, Walter C., Delly, John Gustav, and Polenik, Samuel James, The Particle Atlas Edition Two, volume V, Ann Arbor 1979, articles 837, 838, 839, 840, 841, 842, 843, 844, 845, 846, 847, 848, 849, 850, 851
- Moore, Tommy D., Spence, Liter E., Dugnolle, Charles E., Hepworth, William G., editor, “Identification of the Dorsal Guard Hairs of some Mammals of Wyoming” (Wyoming Game and Fish Department Bulletin No. 14) Cheyenne 1974.
- Stoves, J. L., Fibre Microscopy, Princeton 1958.
- Von Bergen, Werner and Krauss, Walter, Textile Fiber Atlas, New York 1942.
- Wildman, A. B., Microscopy of Animal Textile Fibres, Leeds 1954.
- Williams Cecil S. “Aids to the identification of mole and shrew hairs with general comments on hair structure and hair identification,” Journal of Wildlife Management 2 (1938) 239– 250.
Section Index |