PROTECTIVE SURFACE COATINGS FOR DAGUERREOTYPES
M. Susan Barger, A.P. Giri, William B. White, William S. Ginell, & Frank Preusser
ABSTRACT—In certain instances, it may be desirable to protect a daguerreotype from corrosion by the application of a surface coating. Protective coatings must be both chemically and physically non-reactive with the daguerreotype image material, and, more importantly, must not interfere with the optical properties of daguerreotypes. Sputtered coatings such as silicon dioxide, boron nitride, lanthanum hexaboride, aluminum nitride, and titanium dioxide, as well as a vapor-deposited polymeric coating, Parylene C, have been tested. The results of these tests are discussed.
1 INTRODUCTION
THE IDEA OF USING protective coatings and varnishes on daguerreotypes is nothing new. There are many references in the literature of both the daguerreian era and after which recommend various coatings for daguerreotypes. However, the known incidence of coated nineteenth-century daguerreotypes that have survived is scant. The oldest surviving American daguerreotype, Joseph Saxton's “View of the Central High School,” is coated with a copal varnish.1 Of the nearly two hundred daguerreotypes analyzed at the Materials Research Laboratory, only one other was found to be coated. It appears to have been coated with a sugar solution. These two are the only experimentally confirmed examples of coated ninteenth-century daguerreotypes.
The first use of coatings was to improve the mechanical stability of the image of ungilded daguerreotypes. In that instance, the image is made of a variety of silver amalgam crystals. Characteristically, these crystals are somewhat unstable solids that gradually gain mechanical strength over time due to mercury loss or more complete amalgamation by a process called hardening.2 For a time, daguerreotypes made without gilding remain quite fragile and the image can be removed by the slightest touch. Daguerre himself tried several of the standard organic coating materials in common use at that time—amber, copal, caoutchouc, wax, and other resins—to protect the freshly made images.3 He observed that all of these coatings considerably altered the appearance of the daguerreotypes by making the highlights appear less white and by dulling the black tones. The aging of these organic coatings caused the images to become increasingly less distinct. Others also recommended coatings, such as dextrose.4
The damaging optical effects from the coatings appear to have outweighed any advantage that they may have offered and they were not adopted for general use. Instead, what has become the traditional daguerreian package consisting of daguerreotype, mat, cover glass, and tape seal in a frame or case was adopted to protect the image. In addition, the discovery of gilding, in 1840, improved both the mechanical and optical strength of the image. The universal use of this processing step removed the need for a varnish to protect the image from immediate physical damage.
From time to time, other coatings to protect daguerreotypes from corrosion have been suggested, but again there is little evidence that these were used. The corrosion-resistant coatings include all of the varnishes listed above and also materials such as heated egg albumen.5 As with the varnishes used for mechanical protection, the alteration of the daguerreotype appearance was an unacceptable loss, disproportionate to any prophylactic qualities of the coatings.
Despite this dismal history, there may be occasions when a carefully chosen protective coating is desirable for the preservation of a daguerreotype. The prime function of such a coating should be to prevent corrosion. It is unlikely that any thin layer will have much effect on the inherent ductility of silver and copper in the daguerreotype plate. Most appropriately, a coating can be used to stop the repeated cleaning and corrosion cycle that has been used to treat so many of our most valuable daguerreotypes. Certain coatings may also provide some enhancement of the optical properties of a daguerreotype. For instance, an anti-reflectance coating could be used to improve the apparent contrast6 of an over-cleaned daguerreotype. The traditional daguerreotype package provides protection from mechanical damage, and it is also a very effective means of inhibiting corrosion. Although protective coatings provide an additional corrosion barrier, they should not be used as a substitute for casing these images.
Of course, the decision to coat a daguerreotype with anything is extraordinary and should not be done without the careful consideration and advice of a curator familiar with the value and importance of a particular daguerreotype. Coating should be viewed as a drastic measure to be used only when the corrosion-cleaning-corrosion cycle must be absolutely halted. While we recognize that the widespread use of protective coatings may not be warranted, we have tested a variety of modern coating materials that might be useful at times when such coatings are desired. The coatings used in this study all require some degree of specialized equipment. All of the coatings are used in various industries, and it is possible to make arrangements for coating applications on a fairly routine and relatively inexpensive basis. The coating materials tested include sputtered coatings of insulating metal oxides and nitrides—silica (SiO2), titania (TiO2), boron nitride (BN), and aluminum nitride (AIN); sputtered coatings of metallic lanthanum hexaboride (LaB6); and vapor-deposited coatings of Parylene C, a polymeric film. The sputtered coatings were applied at the Materials Research Laboratory (MRL) of the Pennsylvania State University. The Parylene C coatings were applied at McDonnell Douglas Astronautics Company in a cooperative effort with the J. Paul Getty Conservation Institute and the J. Paul Getty Museum. All physical characterization was done at the MRL.
2 EXPERIMENTAL DETAILS
2.1 Samples
Fifteen nineteenth-century daguerreotypes from the MRL working collection7 were used for the major analysis of protective coatings. These samples were chosen because they are representative of the commonly found variations in daguerreotypes caused by past handling, storage, and aging. Each of these daguerreotypes was arbitrarily cut into three portions. One portion was left uncoated, one received a sputtered coating, and one was coated with Parylene C. This allowed a direct comparison of the various coatings on the same daguerreotype. In addition, some tests were done on modern daguerreotype step tablets and on polished, unexposed daguerreotype plates. Experimental results using step tablets are not substantially different from those on nineteenth-century images. However, it is very difficult to access the visual effect a coating will have on the optical properties of images using step tablets. Further, modern step tablets give no information about the physical and chemical interaction of coatings with the variety of corrosion products found on daguerreotype surfaces. For these reasons, the tests on modern step tablets are not discussed here.
2.2 Sputtered Coatings
The sputtered coatings were deposited in an rf diode sputtering unit, MRC model number SES 8632. Each daguerreotype was placed in the sputtering chamber without any special preparation. A small glass cover slip was placed on the surface so that the coating thickness could be measured using a stylus profilometer. The chamber was closed and pumped down to a base pressure of ∼3 � 10−7 Torr, which increased to ≃3 � 10−6 Torr after throttling and before sputter gas admission. Various gases were injected into the sputtering chamber using leak valves. Gas flow and pressures were monitored using an MKS Flow Ratio Controller Model 254. Sputtering conditions for the various coatings are given in Table 1. All of the targets were two inches in diameter, except the SiO2 and the aluminum targets which were five inches. Aluminum nitride (AIN) was obtained by reactive sputtering of Al-metal target in an Argon (Ar)—Nitrogen (N2) atmosphere. Different coatings (i.e., different compositions, physical structures, or thicknesses) obtained by either changing the sputtering conditions or the time of deposition.
Table 1. Sputtering Conditions
The coating materials were chosen for their optical properties, for their chemical inertness and stability, and for corrosion resistance. The optical properties of all the coating materials are given in Table 2. The composition and structure of the coatings were controlled by the range of gas partial pressures and total pressures used. This, in turn, allowed the optical properties and corrosion resistance of the coatings to be controlled.
Table 2. Optical Properties of Coating Materials
2.3 Vapor-Deposited Polymeric Coating
Parylene C is an unusual plastic film material that is used extensively in industry as a protective coating for electronic and biomedical components. Its principal advantages are: non-toxicity, very low water and gas permeability, high strength, insolubility in all common solvents, transparency in the visible region, and ability to form adherent, pinhole-free conformal coatings. Parylene C films are highly crystalline and are produced by polymerization of a diradical species, di-monochloro-paraxylylene, directly onto a surface.8 The diradical is obtained by vaporization of the solid dimer and pyrolysis of the vapor at high temperatures and low pressures. The highly reactive gaseous diradical will deposit directly from the vapor and will polymerize on any cool surface. Because deposition occurs at very low pressures, films formed by this process follow the contours of the substrate, as well as being uniform and continuous. Parylene C films can be removed from surfaces with orthodichlorobenzene.9 It has no effect on either silver, the image microstructure, or the assorted corrosion products found on daguerreotype surfaces.
The daguerreotypes used in this study were coated in a Union Carbide Corporation (UCC) deposition system, Model 1050. Prior to coating, the samples were washed in ethanol, vapor degreased in Freon 113, and primed with UCC A-174, a substituted silane. The use of a silane primer is usually recommended to improve the adhesion of Parylene C to metallic surfaces. Priming does not affect the visual appearance of the coated daguerreotype. Various coating thicknesses ranging from 1 to 5 μm were tried and deposition conditions altered to determine the effects of these variables on the visual appearance of the daguerreotype image.
The absorption spectrum of Parylene C is featureless from about 300 to 2000 nm except for interference fringes. Transmission in the visible is ∼100% at the interference maxima for a 1.0 μm film. Only slight traces of interference colors were observed on daguerreotypes coated with Parylene films thicker than 2–3 μm.
2.4 Film Characterization Methods
All of the coatings were examined and characterized after deposition to determine film structure, composition, and the optical effect of the films on the daguerreotype image. Film structure and composition were analyzed using an ISI DS 130 scanning electron microscope (SEM) with a Kevex Energy Dispersive X-Ray Detector (EDX). The optical properties of the films in the near IR-visible-near UV regions of the spectrum were assessed using a Beckman DK2A Reflectance Spectrometer with a lead sulfide detector for λ = 2000-500 nm and a photomultiplier tube detector for λ 550-300 nm. The spectrometer has a barium sulfate coated integrating sphere, and barium sulfate was also used as the reference material. The spectrometer was used to measure total (diffuse + specular) reflectance. For each portion of the sample daguerreotypes, the reflectance of a uniform section (�″ diameter) of the image background (a midtone) was measured.10 This type of spectroscopy gives information about the optical effects of the coating on the daguerreotype and on the physical state of the image microstructure.
Fourier Transform Infrared Spectrometry (FTIR) in the region of 4000-500 cm−1 was used to evaluate the interaction of the coating materials with daguerreotype corrosion products. The mid-IR spectra of daguerreotypes contains information concerning corrosion and past treatments of daguerreotypes. It was felt that, especially for permanent coatings such as the sputtered films, the absorption spectra of the coating should not mask this region of the reflection spectrum of the daguerreotype.
Both a Talysurf 10 stylus profilometer and an interferometer were used to try to measure the thickness of the sputtered films. Neith of these techniques was successful because the daguerreotypes were not flat enough for accurate measurements. The film thickness of all the sputtered films was estimated on the basis of coating deposition conditions and varied between 0.1 and 0.2 μm. The thickness of the Parylene C films was determined by measurements made on copper witness plates that were coated along with the daguerreotype samples. An eddy current probe was used for this purpose. Table 3 lists the measured film thicknesses for all the samples produced.
3 RESULTS AND DISCUSSION
OF THE FIVE SPUTTERED COATINGS, two, SiO2 and BN, produced coatings of interest. TiO2 and AIN were not acceptable because the sputtered coatings were non-stoichiometric. Although in principle these materials have desirable optical features, the sputtered films did not match the optical properties of the bulk materials. LaB6 produced a metallic, absorbing film that gave the daguerreotypes the appearance of detailed tintypes. Because LaB6 is a metal, this is not surprising. However, it was of interest to test a metal-on-metal coating to see if it would severely affect the daguerreotype appearance. In addition, TiO2 and LaB6 both exhibited poor adhesion to the daguerreotype surface. These coatings were excluded from further study, and for that reason no micrographs or spectra of the materials are included. However, these samples are included in Table 4 which provides a subjective description of the appearance of all the daguerreotypes studied.
The failure of the TiO2 coatings was due, primarily, to the non-stoichiometry of the sputtered films. Pure TiO2 would have been an acceptable coating material. However, even small deviations from stoichiometry (one or two parts per thousand) produce a material with high electrical conductivity and strong optical absorption. Clearly, this would not be a desirable coating. Our coating procedure produced TiO2-x, where x varied between ∼0.1–0.4. All of these coatings were strongly colored and tended to overwhelm the image. The coating structure was inconsistent and had poor adhesion to the daguerreotype surface.
The coatings of AIN were very consistent and the film structure closely mimicked the underlying daguerreotype. Unfortunately, all of these coatings were bright pink due to non-stoichiometry of the film. Decreasing the film thickness only reduced the intensity of the pink coloration. The AIN coating maintained the specularity characteristic of the daguerreotype image. In some cases, the apparent contrast of the daguerreotype was increased by the AIN.
Silica was sputtered successfully to produce coatings of SiO2. The SiO2 films had a pinkish-to-greenish cast, but the coloration was not pronounced. The films retained the specular, metallic appearance of uncoated daguerreotypes.
A typical set of reflectance spectra that illustrate the difference between the uncoated and SiO2-coated portions of one of the sample daguerreotypes is shown in Fig. 1 (Dag 19–60). Ideally, the visible spectrum of a coated daguerreotype should match that of an uncoated sample. Mismatches indicate that the optical properties of the daguerreotype have been altered because of the coating. The degree of matching is directly related to the appearance of the coated daguerreotype. The short-dashed line in Fig. 1 shows the typical reflectance spectrum of an SiO2 film on a daguerreotype surface. The regular undulations in the spectrum are due to interference fringes. Generally, an SiO2 film on a daguerreotype surface is slightly more absorbing than an uncoated daguerreotype.
Fig. 1.
Total (diffuse + specular) reflectance for an SiO2 coating, a Parylene coating, and an uncoated portion of sample Dag 19–60. All reflectance measurements were made on a uniform piece of the image background.
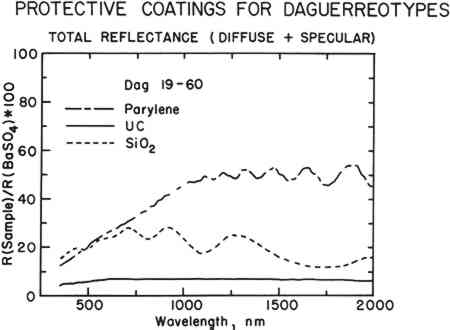 |
The SiO2 coatings also have a shimmery appearance which comes about because of their film structure. SiO2 tends to form sheets of small silica spheres whose diameters are less than 1 μm. The micrographs in the top row of Fig. 2 show the general sphere structure of these coatings and the changes with film thickness. The SiO2 film shown for sample Dag 19–64 does not show the spherical structure because of the deposition conditions.11 The thickest film has the highest spherical SiO2 particle density. This structure is typical of silica, and it is what gives rise to the fire in gem opals. Figure 3 shows individual SiO2 spheres at two high magnifications. Surprisingly, even though these SiO2 spheres are about the same size as daguerreotype image particles, their presence does not seriously interfere with the light scattering from the image. On the contrary, these films tend to slightly enhance the apparent contrast of the image.
Fig. 2.
Secondary electron images of both coated and uncoated portions of Dag 19–60, Dag 19–70, and Dag 19–64. The top row of micrographs shows the microstructure of SiO2 coatings. The bottom row shows Parylene coatings. Uncoated portions of the coated daguerreotypes are shown in the middle row. Each vertical column shows the data for one daguerreotype. The scale is indicated by the bars in each micrograph.
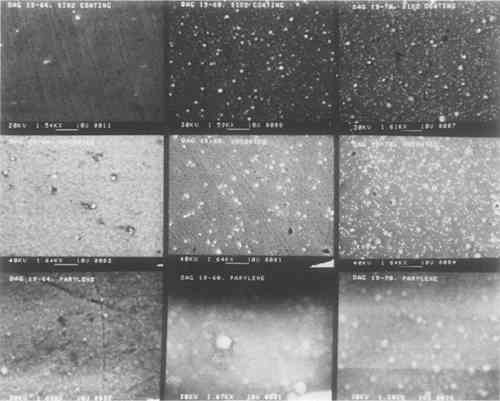 |
Fig. 3.
Individual SiO2 spheres that make up the microstructure of the SiO2 coatings at two different magnifications. Scale is shown by the bars in each micrograph.
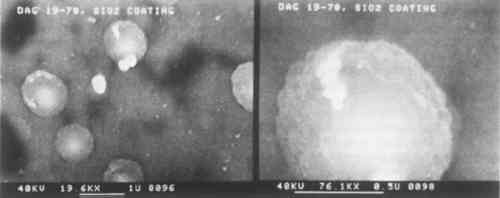 |
The sphere-like structure does not provide a continuous coating for the daguerreotype. Spaces between the spheres are likely places for the initiation of corrosion. This effect was demonstrated in the accelerated aging tests described below.
BN forms a consistently dense, continuous film that closely follows the underlying daguerreotype surface, as seen in the first row of micrographs in Fig. 4. Thicker films tend to be the most dense. These coatings compare favorably with the uncoated portions of the sample daguerreotypes (middle row of Fig. 4). Although BN is usually formed with a graphite-like hexagonal structure (i.e., soft) and is susceptible to moisture attack, proper control of the sputtering conditions produes a very hard, diamondlike BN phase which, not surprisingly, has many of the properties of diamond, i.e., optical transparency, resistance to chemical attack, and high hardness.12 The preparation of this metastable cubic BN phase has just recently been discovered and has a number of potential applications, including coatings on cutting tools.
Fig. 4.
Secondary electron images of both coated and uncoated portions of Dag 19–79, Dag 19–10, and Dag 19–50. The top row of micrographs shows the microstructure of BN. The bottom row shows the Parylene coatings. Uncoated portions of the daguerreotype are shown in the middle row. Each vertical column shows the data for one daguerreotype. The scale is indicated by the bars in each micrograph.
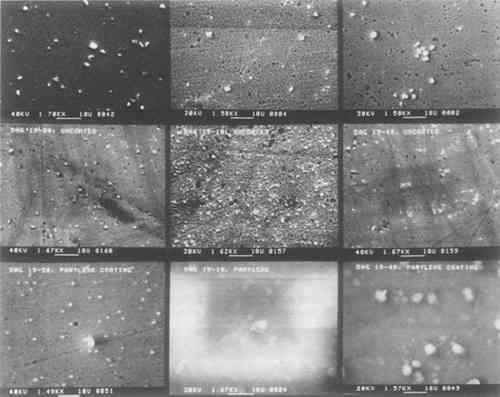 |
Freshly deposited BN coatings have a gold or rose-gold cast. As the coating ages, this coloration tends to dissipate and the films become colorless. This change in appearance usually occurs in a matter of weeks and the exact reason for this change has not been determined. Thicker films may not become colorless for a few months. A typical spectrum for BN coatings is shown by the short-dashed line in Fig. 5. The BN spectrum closely matches that of the uncoated sample. All of the BN spectra follow the curve shape of the uncoated daguerreotypes, but some of these coatings were from 5–10% more absorbing than the uncoated daguerreotypes. The visual appearance of these films, disregarding the coloration, is very close to that of the uncoated samples. The more absorbing films cause some enhancement of the apparent contrast of the daguerreotypes.
Fig. 5.
Total (diffuse + specular) reflectance for a BN, a Parylene coating, and uncoated portion of sample Dag 19–10. All reflectance measurements were made on a uniform piece of image background.
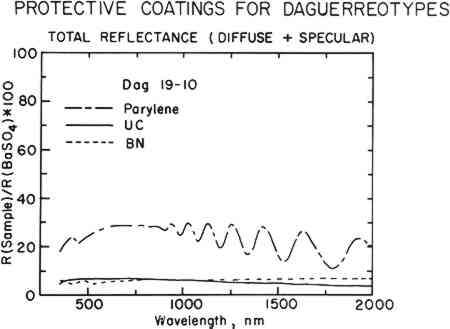 |
One portion of each of the fifteen daguerreotype samples used in this study was coated with Parylene C. This coating is transparent and colorless, but there are noticeable interference fringes on many of the Parylene C-coated samples. However, their occurrence and severity may be controlled by altering the coating thickness. Unlike any of the other coating materials, Parylene C may be removed using orthodichlorobenzene.
The structure of the Parylene C films varied from sample to sample. Some coatings have many clumps of unreacted dimer material distributed over the film surface, as seen in the left micrograph (Dag 19–18) of Fig. 6. A few of the Parylene C films were totally structureless and appear to be draped over the image microstructure. This is shown in the right micrograph (Dag 19–11) of Fig. 6. These two micrographs show the extremes of Parylene C film structure. Other Parylene C films are shown in the bottom rows of micrographs in Figs. 2 and 4. Some of the micrographs appear indistinct because of interactions between the non-conducting polymer and the electron beam of the SEM. These films were not gold coated prior to SEM examination because the gold would have interfered with any subsequent visual examination of the coatings.
Fig. 6.
Secondary electron images showing the extreme variations in Parylene film structure. The left micrograph (Dag 19–18) shows crystalline clumps embedded in the polymer coating. The right micrograph (Dag 19–11) shows the conformal, structureless polymer film. Scale is indicated by the bars in each micrograph.
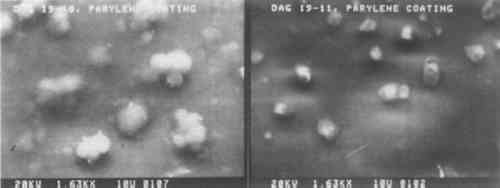 |
Parylene C films are more absorbing than any of the other coatings, primarily because they are from 5 to 40 times the thickness of the sputtered films. The long-and-short dashed lines in the spectra of Figs. 1 and 5 show typical reflectance data for these films. The undulations in the curves are due to interference fringes. All of the Parylene C films have similar spectra, although some coatings are less absorbing than others because of film thickness variation among the samples. Most of the Parylene C films reduce the specularity of the daguerreotype and cause the surface of the image to appear dulled or matte. The image remains visible, but there is a loss of contrast and detail. In some cases, the image tone was shifted from a “warm” to a “cold” cast. The image contrast appears almost reversed (positive to negative) in the most severe cases. Thus, while the image is still visible, the characteristic image quality associated with daguerreotypes is lost to a large extent.
Daguerreotypes are usually cased and the case provides a fairly effective barrier to corrosion. Unlike most other silver objects, cased daguerreotypes are more vulnerable to oxidation rather than to sulfidation. Some silver sulfide is present on daguerreotype surfaces, but the prevalent corrosion product is silver oxide. This is because of the vastly greater availability of oxygen in the general environment over the availability of unreduced sulfur, as well as to the physical constraints of the traditional daguerreotype package. Fluctuations of the environment within the daguerreotype package, such as changes in water vapor (humidity), temperature, and air exchange, control the corrosion rate. The size of the environment inside the package also affects corrosion. The presence of small amounts of harmful materials can have a greater effect because there is only negligible dilution from the outside atmosphere. For these reasons, the environment within the package can be quite harmful to the daguerreotype.
A preliminary test of the ability of BN, SiO2, and Parylene C films to provide protection against corrosion of daguerreotypes was carried out using sulfur-containing gases. These tests were carried out in sulfur gas atmospheres because the kinetics of sulfur attack of silver are much faster than those of oxygen attack. Thus, in a relatively short time, these tests give a very good qualitative clue as to the protective characteristics of the coatings. This is particularly true with regard to mechanical completeness or freedom from pinholes and flaws. These tests are not very effective for accessing the resistance of the films to corrosion initiated by diffusion of gases through the film (unless the transport mechanism for sulfur or oxygen is the same for a given film). They also give very little information about chemical attack of the film itself. Since it is expected that daguerreotypes will always be sealed in cases, these last two points are of minor interest compared to the completeness of the films.
Samples of BN and SiO2-coated unimaged daguerreotype plates were subjected to gaseous H2S (vapor over a saturated aqueous K2Sx solution) and SO2 (vapor over a 0.5 M NaHSO3 solution) in separate sealed beakers over a 72-hour period. A Parylene C-coated daguerreotype (19–79) was exposed only to the H2S environment. Uncoated areas on the samples served as controls. The concentrations of H2S and SO2 were not measured, but these exposure conditions are orders of magnitude more severe than would be expected during normal storage of daguerreotypes. Within one hour, the uncoated controls showed evidence of corrosion. At the end of the test, most of the BN-coated plates and the Parylene C coated sample were unaffected, but the SiO2 films did not prove protective.
The mechanical adhesion of the BN and Parylene C coatings to the daguerreotype surface was tested using Method B described in the ASTM Standard D3359-83, Standard Methods for Measuring Adhesion by Tape Test. This is a very simple test used to establish adequate adhesion of coating materials to metal substrates. A razor blade is used to score the coating; a piece of transparent tape is placed over the scored area and burnished using the eraser end of a pencil; finally, the tape is pulled off the surface. The scored area is inspected under a light microscope for removal or peeling of the coating. The tests for both the BN and Parylene C showed either no peeling or removal (Classification 5B). This is good evidence of the strong adhesion of these films to the daguerreotype surface.
The long-term stability and aging properties of the coatings were not examined. Although all organic materials oxidize and are subject to accelerated degradation in the presence of light, antioxidizers and stabilizers are available that will prolong the useful life of polymeric coatings. Tests have demonstrated that the generic Parylenes have excellent oxidation resistance which can be enhanced further by incorporation of selective antioxidants.13 However, should degradation occur, the coating is reversible and may be replaced.
The sputtered film materials were chosen because they are inert and should not interact with either the environment or the daguerreotype over the course of time. However, these films cannot be easily removed if they fail. It would be possible to sputter the coating off the daguerreotype surface, but this may not always be desirable. If the sputtered coatings are used, they should be considered a permanent addition to the daguerreotype.
4 CONCLUSIONS
THE JUDICIOUS USE OF protective coatings on certain daguerreotypes can be a useful step in their long-term preservation. In particular, coatings offer a way to break the harmful cycle of repeated corrosion and cleaning that has been used to treat so many daguerreotypes.
The most important characteristic of any daguerreotype coating is that it must not modify the optical properties of the image. A daguerreotype should not be regarded solely as a metal object containing a photographic image. The image on a daguerreotype is unique and quite unlike any other kind of photographic image. This image is the result of visible light scatter from the image microstructure. As such, it is a very narrowly defined physical phenomenon. In this set of physical constraints, very small physical changes can result in large changes of the image appearance.14 Any coating that overpowers the optical properties of the image is not acceptable.
Additionally, a coating should offer protection from corrosion over and above that offered by the daguerreotype package alone. A coating should have a high degree of long-term physical and chemical stability. It is also desirable that coating structure and deposition should be controllable and predictable.
Several modern coating materials were tested for use on daguerreotypes. Of those tested, the best coatings were the sputtered coatings, silica and boron nitride, and the polymer coating, Parylene C.
The inherent sphere-like structure of SiO2 coatings makes them the least corrosion resistant of the final three coatings. These films are slightly more colored than would be acceptable for daguerreotype coatings, although this coloration can be eliminated by using thinner films.
The Parylene C films tested modified the optical properties of the daguerreotypes and the films were more absorbing in the visible than either SiO2 or BN. These effects are due to the relatively thick films (2–4 μm) deposited on the test samples. Reduction of film thickness to the 0.1–0.2 μm range should alleviate this problem. The variability in film structure from one run to the next is a processing problem that requires closer control of the deposition variables. Although the long-term stability of organic coatings is of some concern, the reversibility of Parylene C allows the removal and replacement of the coating as required.
BN produced a dense, highly corrosion-resistant coating. The film structure was consistent and controllable from sample to sample. Initially, BN films have a gold or rose-gold color, but the coloration disappears over time. Controlling film thickness can eliminate this effect altogether. Most importantly, the BN coatings do not interfere with the optical properties of the image. The UV-VIS-near IR reflectance data show that anti-reflectance coatings were achieved by the BN as seen by the close match in the reflectance spectra. BN is inert and should not cause any long-term damage to a daguerreotype. It cannot be considered a removable film, and this must be accepted if BN is used. The FTIR data show that BN does not distort the corrosion and past treatment information contained in the 4000-500 cm−1 region of the spectrum. Hence, the presence of a BN coating does not preclude future analysis of a daguerreotype by SEM, x-ray, UV-VIS-near IR spectroscopy, or FTIR.
On the whole, BN, of all the coatings tested to date, has the most of the desirable film properties required for protection of daguerreotypes. However, because two of the materials studied have the potential for providing acceptable coatings, additional work should be done on stoichiometric TiO2 and on uncontaminated, much thinner Parylene C films.
ACKNOWLEDGEMENTS
THE PORTION OF THIS WORK performed at the Materials Research Laboratory of The Pennsylvania State University was funded by the Andrew W. Mellon Foundation. A Lucretia V.T. Simmons Project Renew Grant from the State College (PA) Branch of the American Association for University Women to one of the authors (MSB) provided for the purchase of the nineteenth-century daguerreotypes used for testing.
The Parylene C work is part of a more extensive study being conducted under Zdravko Barov at the J. Paul Getty Museum Antiquities Conservation Department on the use of this material for conservation purposes. We wish to thank John Thomas and the McDonnell Douglas Astronautics Company for making the Parylene C deposition equipment available for this study.
REFERENCES
Barger, M. Susan (1983). “Robert Cornelius and the Science of Daguerreotypy,” in William F.Stapp, Robert Cornelius: Portraits from the Dawn of Photography. Washington, DC: Smithsonian Press, p. 123.
Barger, M. Susan, R.Messier and W.B.White. “Gilding and Sealing Daguerreotypes,” Photographic Science and Engineering27 (1983): 141–146
DaguerreL.J.M. (1839). “Practical description of the process called the Daguerreotype”, (John F. Frazer, trans.) Journal of the Franklin Institute24 (1839): 303–311
Daguerre, L.J.M. “The Daguerreotype”, Journal of the Franklin Institute, 29 (1840): 142
Shoemaker, W.L. “Cleaning the Daguerreotype”, Philadelphia Photographer14 (1877): 233.
“Apparent contrast” and “apparent density” are terms used in connection with daguerreotypes because the appearance of contrast and density in these images is due to light reflectance and scatter, rather than to light absorption. Formally, true optical density is due to light absorption, which does not apply to the daguerreian system. For a more detailed discussion see: Barger, M. Susan, R. Messier, and W.B. White. “A Physical Model for the Daguerreotype,” Photographic Science and Engineering26 (1982): 285–291.
There has been ongoing, extensive research into the material properties and preservation of daguerreotypes at the Materials Research Laboratory of The Pennsylvania State University since 1979. The working collection of some 150 sample daguerreotypes has come from a variety of sources and are chosen because they range over the entire spectrum of daguerreotypes found in collections. Overall, very few of these daguerreotypes have been subjected to destructive testing. All daguerreotypes in the working collection are carefully checked before experimental work is done on them to make sure that no daguerreotypes of historic or aesthetic value are harmed. In addition, close to fifty more daguerreotypes have been submitted for analysis by other institutions and private owners. These daguerreotypes are treated as part of the study, but, of course, are returned to the owners when analyses are completed.
Gorham, W.F. “A New, General Synthetic Method for the Preparation of Linear Poly-pxylylenes”;, Journal of Polymer Science Part A-1, 4 (1966): 3027–3039.
To remove the Parylene C film, a daguerreotype is immersed in hot (165�C) orthodichlorobenzene which converts the film to a gelatinous mass that can be easily removed with gentle swabbing. This process must be carried out in a fume hood that is suitable for handling chlorinated solvents. The toxicity of orthodichlorobenzene is about the same as paradichlorobenzene, a commonly used mothproofing insecticide.
BargerM. Susan, R.Messier and W.B.White, “Nondestructive Assessment of Daguerreotype Image Quality by Diffuse Reflectance Spectroscopy” Studies in Conservation29 (1984): 84–86.
During the deposition of this film, there was an uncontrollable drop in voltage due to a temporary power failure.
R.Messier, personal communication.
Baker, T.E., G.L.Fix and J.S.Judge. “Modified Poly-Paraxylylene Coatings and Films with Improved Oxidation Resistance”, Journal of the Electrochemical Society127 (1980): 1851–1852.
Barger, M. Susan, R.Messier, and W.B.White. “A Physical Model for the Daguerreotype”, Photographic Science and Engineering26 (1982): 285–291
Section Index |