DIGITAL RADIOGRAPHY IN THE ANALYSIS OF PAINTINGS: A NEW AND PROMISING TECHNIQUE
A. Everette James, S. Julian Gibbs, Malcolm Sloan, Ronald R. Price, & Jon J. Erickson
ABSTRACT—Recent advances in computer processing of images and the development of electronic x-ray detectors have made possible the development of digital radiographic techniques, which offer great potential for the analysis of paintings. The ability to distinguish very small differences in x-ray attenuation characteristics provides the possibility of quantitative analysis of pigment structures. The very sophisticated image processing techniques that have been developed for the space industry and medicine also may be applied to the analysis of paintings through the use of the digital radiographic images. In this communication, digital fluoroscopy, scanned projection radiography, and scanned point-source methods will be described. The virtues and limitations of each are noted. An initial experience with two of these methods will be the major subject of this communication.
RADIOGRAPHIC TECHNIQUES have been used for many years in the analysis of works of art, utilizing conventional silver halide films for recording, storing, and displaying the images so obtained.1 Recently, digital radiographic techniques have been developed, using computer methods for all three functions formerly assigned to film. These computer techniques are rapidly gaining widespread acceptance and use in medical radiology. Although digital units were introduced to medical radiology less than five years ago, some 45 manufacturers now offer such units and more than 1,000 are in use. A major text has recently been published on the subject.2
Conventional film has several disadvantages as an image recording medium for the radiography of paintings. The x-ray attenuation characteristics of any given painting are unknown before several radiographs are exposed, developed, and evaluated. Thus, several trial exposures must frequently be made before a radiograph of optimal density is obtained. Further, it is not always possible for the observer to perceive the entire density range from a single radiograph. Several films obtained at differing exposures may be required to display the complete spectrum of density differences providing maximum radiographic information obtained from a single painting. Finally, the inherent radiographic subject contrast of many paintings is quite low. Thus, important image details may be difficult to perceive in film radiographs because of the subtle gradations in density. Digital imaging techniques overcome many of these difficulties. Our experience with certain of these computerized methods is summarized in this introductory communication.
1 Digital Fluoroscopy
Digital fluoroscopy3, 4 is accomplished using a modified version of the standard radiographic system in which the image is produced by passing x-rays through an object (in this discussion, a painting) to fall on an image intensifier tube acting as the receptor (Fig. 1). In conventional systems, the output of the image intensifier is viewed by a video camera for display or by a film camera system for permanent recording. In the digital fluoroscopy system, a video camera designed for maximum fidelity and minimum noise and distortion is required if the full advantages of digital technology are to be gained. The output of the video camera is converted from its analog electrical signal (voltage) to a digitized format which is stored in the computer.
Fig. 1.
Schematic drawing of a digital fluoroscopy system. The attenuated x-ray beam, after passage through the object, is detected by the image intensifier. Output from the camera which views the intensifier is amplified and passed through the analog to digital converter (ADC) to provide digital information for computer storage. At display time, the image is converted back to the analog video signal through the digital to analog converter.
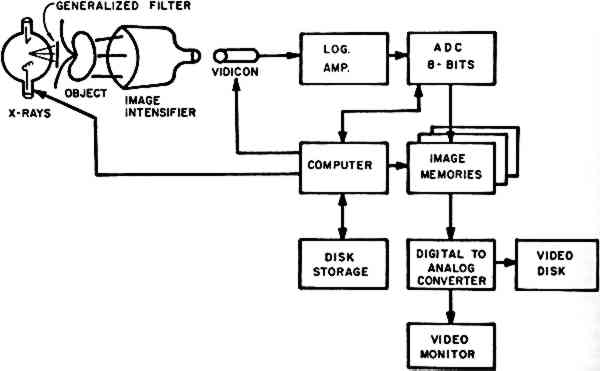 |
A major advantage of this technique is the instantaneous display of the radiographic image on a television screen. This means that the image may be evaluated for proper exposure and positioning factor while it is being obtained. A second advantage is the very fine spatial resolution that is possible with this imaging device, which is important if one is interested in small details in the images of the paintings. Current image intensifiers have the capability of showing details as small as 0.25 mm in size, allowing the display of fine detail in individual brush strokes in the painting. The major difficulty with the use of this imaging modality is the limited area that can be imaged at one time. The largest image intensifier currently available is only 14 inches in diameter. Thus, the examination of larger areas would require multiple exposures. This system is thus useful for the detailed analysis of a small painting or a portion of a large one, while other digital techniques described subsequently in this communication may be used for overall analysis of works of major size. The digital imaging techniques offers new nondestructive ways to evaluate a painting as well as to compare the components of the work, the artistic technique employed, and the condition of the work.
The digital fluroscopic images in Figure 2 show an example of one of the more common techniques of computer image processing, known as subtraction. If two radiographic images of the same subject are obtained under different conditions and one is subtracted from the other, only the differences between the two images are displayed. One of such a pair of images is shown in Figure 2C. The second image of this pair is not shown, since no differences between the two can be detected visually. These images were obtained by slight movement (called “pixel shift”) of the painting between the two exposures. When one of this pair of images is subtracted from the other, the result provides enhancement, or the exaggeration of contrast boundaries (Fig. 2D). Thus, this method makes perceptible some features of the radiographic images that were totally invisible to the unaided eye.
Fig. 2.
Harp of the Winds, by Homer Dodge Martin. Oil on wood.
- Photograph of the painting.
- Film radiograph. Note the low contrast because of the high uniform attenuation of the wood.
- Digital fluoroscopic image of a portion from the right center of the painting. Note increased contrast relative to the film radiograph.
- Subtraction image using the “pixel shift” method. See text for details.
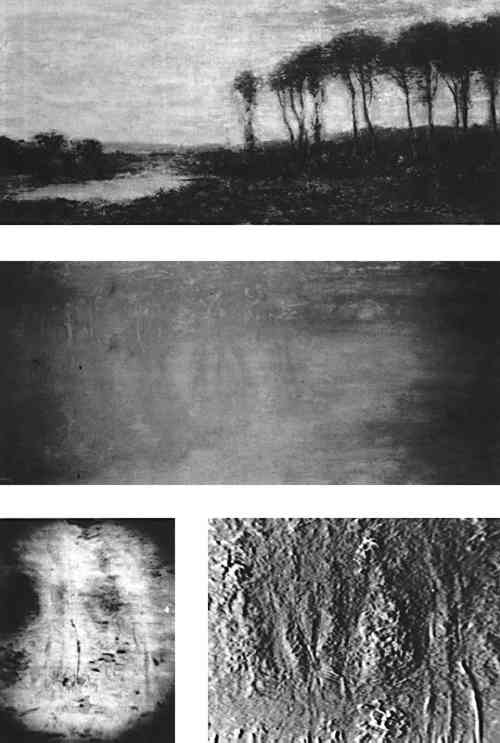 |
Other possibilities for this subtraction technique include energy and temporal subtraction. Energy subtraction is based on the well established fact that the attenuation properties of all materials are functions of the energy of the incident x-ray beam. The ability to perform subtraction using digital radiographs obtained at two different x-ray energies may provide additional information in the analysis of paintings. For example, two different pigments may have attenuation characteristics which are nearly identical at a given x-ray energy, making it difficult to differentiate between the two on the basis of contrast in a single image. However, the energy dependence of the attenuation of the two pigments may differ, allowing enhanced contrast by energy subtraction.
Temporal subtraction involves the use of two images obtained at different times, with the interval ranging from seconds to years. It may be used to study the deterioration of paintings with time, since only the changes in the attenuation pattern will be displayed in the subtracted image. Further, it could be useful in the authentication of paintings. A digital image of a painting obtained and stored could at some later date be subtracted from a similar image of a suspected copy. Any difference between the two would be exaggerated, making identification of the painting from which the later image was obtained relatively simple and quite precise.
2 Scanned Projection Radiography
One of the outgrowths of modern computed tomographic (CT or CAT) scanning instrumentation is a method for performing digital radiography which has many of the features of an ordinary radiographic study but in which each data point (“voxel” or small rectangular portion of the painting) is recorded in a discrete, finite manner.5 This technique is often called the “scout view.” At present, it is available in any hospital radiology department with computed tomography equipment. The method consists of placing the x-ray tube and detector assembly in such a position that the painting may be moved linearly through the x-ray beam (Fig. 3). As it is moved, data are collected by the computer from the detector array, and the image of the painting is thus stored in computer memory for subsequent manipulation and display.
Fig. 3.
Schematic of a scanned projection system. In the scanner, the fan-shaped x-ray beam is stationary, striking a linear array of electronic detectors positioned along an arc of a circle such that all are equidistant from the source. The painting is then passed linearly through the beam at a constant speed while the computer samples each detector at frequent, uniform intervals. Again, the analog signal is digitized and stored in the computer for future display.
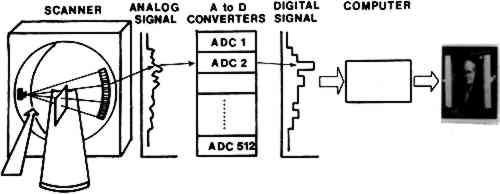 |
The collected image, with or without digital processing, is displayed on a video monitor. In this image, the intensity of each individual point (“pixel”) in the image is proportional to the quantity of x-rays passing through the corresponding point in the painting. The spatial resolution of this system is acceptable but not as good as that obtained by digital fluoroscopy.
A major advantage of the scanned projection technique is that x-ray energies over a wide range may be used. This is possible because the electronics of the detector array can be optimized for the x-ray energy being employed. This calibration procedure is not available for either conventional radiography or digital fluoroscopy. Large fields-of-view are also possible (Figs. 4 and 5). The equipment is expensive ($800,000 to $1,200,000), but is widely available in major medical centers. We have had the opportunity to evaluate several of these systems and feel that they offer great promise in the evaluation of paintings.
Fig. 4.
Self portrait, by Cornelius Hankins. Oil on canvas. Scanned projection radiographs windowed at different levels. See text for details. In A, the image is processed to display details of the canvas painting support (arrows). In B, processing is aimed to show the distribution of pigment. In C, the wood and metal supports of the painting are demonstrated.
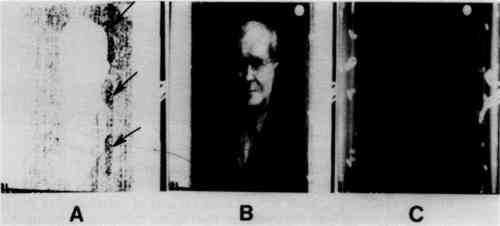 |
Fig. 5.
Mountain Lake, by Nell Choate Jones. Oil on canvas.
- Photograph of the painting.
- Film radiograph. Note lack of contrast and of correlation of the radiographic image with the painting. That is, the thickness of pigment application is independent of the visual display.
- Scanned projection radiograph, windowed to show most painting details. See text. Pigment density pattern is seen in more detail than in B, but stll does not correlate with the painting.
- Another scanned projection radiograph, windowed to show image details overlying the wood frame.
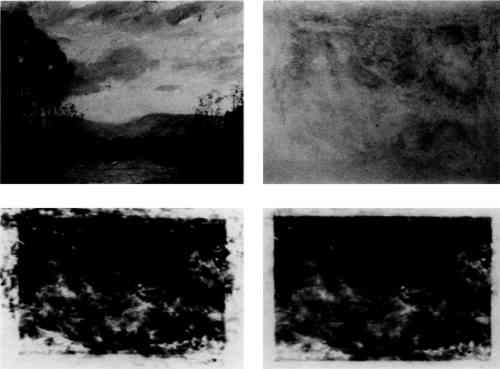 |
The scanned projection images in Figures 4 and 5 demonstrate a second common technique of digital image processing known as “windowing.” Image intensity, or “brightness,” using this equipment is measured on an arbitrary scale in units called Hounsfield units (HU). For the typical machine, the dynamic range is −1024 to 1024 (or more) HU, where −1024 is assigned to the attenuation of air, 0 to water, and 1024 (or the equipment maximum) to the densest material in the subject. If the entire dynamic range of image intensity is displayed simultaneously, the contrast resolution is low, as in film radiography. However, the windowing technique allows the viewer to select only a portion of the dynamic range for display at any one time. For example, in Figure 5C, the window center is −445 HU, and its height is 61. That is, in this display any pixel whose intensity is less than −506 HU (−445 −61) is displayed as black, and any whose intensity is greater than −384 HU (−445+61) is displayed as white. The intensity range between −506 and −384 is spread over the entire −1024 to 1024 dynamic range of the display from black to white, greatly increasing the contrast in the region of interest. To display the more intense portions of the image overlying the wooden frame, a second window whose center is at −376 and whose height is 100 was used (Fig. 5D). These static images can never demonstrate the information display capacity of this technique. It can be accomplished in “real time;” that is, the display can be altered as the window level and height are changed, providing for a continuously varying image that almost resembles a moving picture as it displays the various features of the painting.
3 Scanned Point Source System
A third digital radiographic method is the “flying spot” or scanned point source system.6 In this system, the x-ray image is formed by scanning a fine pencil beam of x-rays over the area being imaged (Figure 6). After passing through the object, the attenuated x-ray beam is detected by a large scintillation crystal whose light output is measured, digitized, and stored in computer memory. The formation of the pencil beam of x-rays is accomplished by a mechanical system of collimators which block the beam and move in such a manner that the beam is scanned over the imaged area in a rectilinear raster. The time required for imaging a painting of moderate size (16 � 24 or 20 � 24 inches) is of the order of five seconds.
Fig. 6.
Scanned point-source system. The x-ray beam arising from the x-ray tube (a) is collimated to a fan shape by the primary collimator (b); these elements move in a linear direction (large arrow) as a subject is scanned. The secondary rotating collimator (c) further collimates the beam to a small pencil shape (d) which is detected by a crystal (e), digitized, and stored.
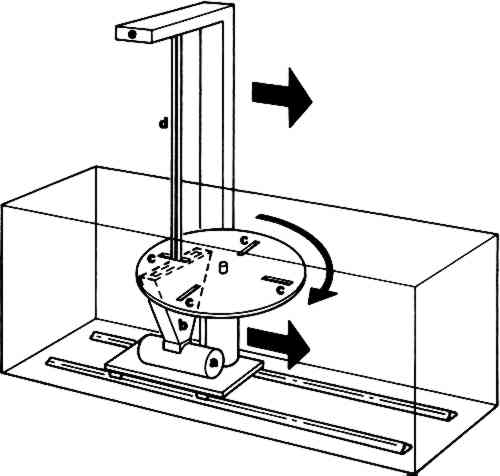 |
This technique has not been generally available because, until recently, the imaging time was of the order of fifteen seconds, and the spatial resolution was significantly less than with other methods. Although these time and resolution limitations are not so important in radiography of paintings, they are in clinical circumstances. Thus, these machines have not been widely employed in medical facilities. At present, images can be obtained with resolution of about two line pairs per millimeter. In addition, images can be stored and repeated images of the same area can be summed to provide higher quality pictures. Data can be manipulated to change contrast and other characteristics as for the other systems of digital radiography. This technique has not as yet received sufficient use to permit us to evaluate it and to compare it with the other two more widely accepted computer methods.
REFERENCES
Bridgeman, and C. Keck, S.1961. The Radiography of Paintings. Rochester, New York: Eastman Kodak Co..
Price, Ronald R., Rollo, F. David, Monahan, W. Gordon, and James, A. Everette, eds.1982. Digital Radiography: A Focus on Clinical Utility. New York, New York: Grune & Stratton, Inc.
Kruger, R. A., Mistretta, C. A., Lancaster, J., et al. 1978. “A digital video image processor for real-time x-ray subtraction imaging,” Optical Eng.17, 652–654.
Nudelman, S., Capp, M. P., and Fisher, H. D. et al. 1978. “Photoelectronic imaging for diagnostic radiology and the digital computer.” Proc. SPIE, 164, 138.
Brody, W. R., Macowski, A., and Lehmann, L., et al. 1980. “Intravenous angiography using scanned projection radiography: Preliminary investigation of a new method.” Invest. Radiol., vol. 15, 220–223.
Tateno, Y., and Tanaka, H.1976. “Low dosage x-ray imaging system employing flying spot x-ray microbeam (Dynamic Scanner).” Radiology, vol. 121, 189–195.
Section Index |